Monocytes in tumor: The perspectives of single-cell analysis
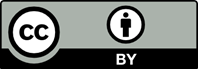
Infiltration of mononuclear phagocytes into the tumor microenvironment (TME) is known to orchestrate the tumor progression and is generally considered to interfere with the efficacy of immune checkpoint blockade therapies. For a more precise definition of monocytes, we review some recent advances in the functions of monocytes and macrophages in TME based on single-cell analysis. We also summarize the details of the different subpopulations of monocytes and macrophages involved in immunotherapy and their potential applications in clinical studies. In this review, we briefly introduce the developmental trajectory of mononuclear phagocytes, including monocytes, tumor-associated macrophages, dendritic cells and myeloid-derived suppressive cells, and their functions in TME. In this review, the potential of monocytes and their derived cells as diagnostic and therapeutic targets, with regard to the interaction between monocytes and immune checkpoint therapies, is also summarized.
van Furth R, Cohn ZA, 1968, The origin and kinetics of mononuclear phagocytes. J Exp Med, 128: 415–435.
https://doi.org/10.1084/jem.128.3.415
Engblom C, Pfirschke C, Pittet MJ, 2016, The role of myeloid cells in cancer therapies. Nat Rev Cancer, 16: 447–462.
https://doi.org/10.1038/nrc.2016.54
Pollard JW, 2004, Tumour-educated macrophages promote tumour progression and metastasis. Nat Rev Cancer, 4: 71–78.
https://doi.org/10.1038/nrc1256
Sica A, Schioppa T, Mantovani A, et al., 2006, Tumour-associated macrophages are a distinct M2 polarised population promoting tumour progression: Potential targets of anti-cancer therapy. Eur J Cancer, 42: 717–727.
https://doi.org/10.1016/j.ejca.2006.01.003
Yunna C, Mengru H, Lei W, et al., 2020. Macrophage M1/ M2 polarization. Eur J Pharmacol, 877: 173090.
https://doi.org/10.1016/j.ejphar.2020.173090
Krieg C, Nowicka M, Guglietta S, et al., 2018, High-dimensional Single-cell Analysis Predicts Response to Anti-PD-1 Immunotherapy. Nat Med, 24: 144–153.
https://doi.org/10.1038/nm.4466
Roberts SA, Waziri AE, Agrawal N, 2016, Development of a single-cell migration and extravasation platform through selective surface modification. Anal Chem, 88:2770–2776.
https://doi.org/10.1021/acs.analchem.5b04391
Chen YC, Allen SG, Ingram PN, et al., 2015, Single-cell migration chip for chemotaxis-based microfluidic selection of heterogeneous cell populations. Scientific reports, 5: 9980.
Zhuang J, Wu Y, Chen L, et al., 2018, Single-cell mobility analysis of metastatic breast cancer cells. Advanced Science (Weinheim, Baden-Wurttemberg, Germany), 5: 1801158.
https://doi.org/10.1002/advs.201801158
Trapnell C, 2015, Defining cell types and states with single-cell genomics. Genome Res, 25: 1491–1498.
Grün D, van Oudenaarden A, 2015, Design and analysis of single-cell sequencing experiments. Cell, 163: 799–810.
https://doi.org/10.1016/j.cell.2015.10.039
Topalian SL, Hodi FS, Brahmer JR, et al., 2012, Safety, activity, and immune correlates of anti-PD-1 antibody in cancer. N Engl J Med, 366: 2443–2454.
https://doi.org/10.1056/NEJMoa1200690
Powles T, Eder JP, Fine GD, et al., 2014, MPDL3280A (anti- PD-L1) treatment leads to clinical activity in metastatic bladder cancer. Nature, 515: 558–562.
https://doi.org/10.1038/nature13904
Rizvi NA, Hellmann MD, Snyder A, et al., 2015, Cancer immunology. Mutational landscape determines sensitivity to PD-1 blockade in non-small cell lung cancer. Science (New York), 348: 124–128.
https://doi.org/10.1126/science.aaa1348
Ansell SM, Lesokhin AM, Borrello I, et al., 2015, PD-1 blockade with nivolumab in relapsed or refractory Hodgkin’s lymphoma. N Engl J Med, 372(4): 311–319.
Robert C, Schachter J, Long GV, et al., 2015, Pembrolizumab versus ipilimumab in advanced melanoma. N Engl J Med, 372(26): 2521–2532.
https://doi.org/10.1056/NEJMoa1503093
Ostrand-Rosenberg S, Sinha P, 2009, Myeloid-derived suppressor cells: Linking inflammation and cancer. J Immunol (Baltimore, Md. 1950), 182(8): 4499–4506.
https://doi.org/10.4049/jimmunol.0802740
Auffray C, Sieweke MH, Geissmann F, 2009, Blood monocytes: Development, heterogeneity, and relationship with dendritic cells. Annu Rev Immunol, 27: 669–692.
https://doi.org/10.1146/annurev.immunol.021908.132557
Shi C, Pamer EG, 2011, Monocyte recruitment during infection and inflammation. Nat Rev Immunol, 11(11): 762–774.
https://doi.org/10.1038/nri3070
Ziegler-Heitbrock L, Ancuta P, Crowe S, et al., 2010, Nomenclature of monocytes and dendritic cells in blood. Blood, 116(16): e74–e80.
https://doi.org/10.1182/blood-2010-02-258558
Auffray C, Fogg D, Garfa M, et al., 2007, Monitoring of blood vessels and tissues by a population of monocytes with patrolling behavior. Science (New York), 317(5838): 666–670.
https://doi.org/10.1126/science.1142883
Movahedi K, Laoui D, Gysemans C, et al., 2010, Different tumor microenvironments contain functionally distinct subsets of macrophages derived from Ly6C(high) monocytes. Cancer Res, 70(14): 5728–5739.
https://doi.org/10.1158/0008-5472.CAN-09-4672
Mildner A, Schönheit J, Giladi A, et al., 2017, Genomic characterization of murine monocytes reveals C/EBPβ transcription factor dependence of Ly6C cells. Immunity, 46(5): 849–862.e7.
https://doi.org/10.1016/j.immuni.2017.04.018
van Galen P, Hovestadt V, Wadsworth Ii MH, et al., 2019, Single-cell RNA-Seq reveals AML hierarchies relevant to disease progression and immunity. Cell, 176(6): 1265–1281.e1224.
https://doi.org/10.1016/j.cell.2019.01.031
Zilionis R, Engblom C, Pfirschke C, et al., 2019, Single-cell transcriptomics of human and mouse lung cancers reveals conserved myeloid populations across individuals and species. Immunity, 50(5): 1317–1334.e1310.
https://doi.org/10.1016/j.immuni.2019.03.009
Puram SV, Tirosh I, Parikh AS, et al., 2017, Single-cell transcriptomic analysis of primary and metastatic tumor ecosystems in head and neck cancer. Cell, 171(7): 1611–1624.e24.
https://doi.org/10.1016/j.cell.2017.10.044
Passlick B, Flieger D, Ziegler-Heitbrock HW, 1989, Identification and characterization of a novel monocyte subpopulation in human peripheral blood. Blood, 74(7): 2527–2534.
Olingy CE, Dinh HQ, Hedrick CC, 2019, Monocyte heterogeneity and functions in cancer. J Leukocyte Biol, 106(2): 309–322.
https://doi.org/10.1002/JLB.4RI0818-311R
Villani AC, Satija R, Reynolds G, et al., 2017, Single-cell RNA-seq reveals new types of human blood dendritic cells, monocytes, and progenitors. Science (New York), 356(6335): eaah4573.
https://doi.org/10.1126/science.aah4573
Qian BZ, Li J, Zhang H, et al., 2011, CCL2 recruits inflammatory monocytes to facilitate breast-tumour metastasis. Nature, 475(7355): 222–225.
https://doi.org/10.1038/nature10138
Schmall A, Al-Tamari HM, Herold S, et al., 2015, Macrophage and cancer cell cross-talk via CCR2 and CX3CR1 is a fundamental mechanism driving lung cancer. Am J Respir Crit Care Med., 191(4): 437–447.
https://doi.org/10.1164/rccm.201406-1137OC
Li X, Yao W, Yuan Y, et al., 2017, Targeting of tumour-infiltrating macrophages via CCL2/CCR2 signalling as a therapeutic strategy against hepatocellular carcinoma. Gut, 66(1): 157–167.
https://doi.org/10.1136/gutjnl-2015-310514
Gordon IO, Freedman RS, 2006, Defective antitumor function of monocyte-derived macrophages from epithelial ovarian cancer patients. Clin Cancer Res, 12(5): 1515–1524.
https://doi.org/10.1158/1078-0432.CCR-05-2254
Sheng J, Chen Q, Soncin I, et al., 2017, A discrete subset of monocyte-derived cells among typical conventional Type 2 dendritic cells can efficiently cross-present. Cell Rep, 21(5): 1203–1214.
https://doi.org/10.1016/j.celrep.2017.10.024
Hanna RN, Cekic C, Sag D, et al., 2015, Patrolling monocytes control tumor metastasis to the lung. Science (New York), 350(6263): 985–990.
https://doi.org/10.1126/science.aac9407
Romano E, Kusio-Kobialka M, Foukas PG, et al., 2015, Ipilimumab-dependent cell-mediated cytotoxicity of regulatory T cells ex vivo by nonclassical monocytes in melanoma patients. Proc Natl Acad Sci U S A, 112(19): 6140–6145.
https://doi.org/10.1073/pnas.1417320112
De Palma M, Venneri MA, Galli R, et al., 2005, Tie2 identifies a hematopoietic lineage of proangiogenic monocytes required for tumor vessel formation and a mesenchymal population of pericyte progenitors. Cancer Cell, 8(3): 211–226.
https://doi.org/10.1016/j.ccr.2005.08.002
Venneri MA, De Palma M, Ponzoni M, et al., 2007, Identification of proangiogenic TIE2–expressing monocytes (TEMs) in human peripheral blood and cancer. Blood, 109(12): 5276–5285.
https://doi.org/10.1182/blood-2006-10-053504
Duan Z, Luo Y, 2021, Targeting macrophages in cancer immunotherapy. Signal Transduct Target Ther, 6(1): 127.
https://doi.org/10.1038/s41392-021-00506-6
Wynn TA, Chawla A, Pollard JW, 2013, Macrophage biology in development, homeostasis and disease. Nature, 496(7446): 445–455.
https://doi.org/10.1038/nature12034
Ginhoux F, Jung S, 2014, Monocytes and macrophages: developmental pathways and tissue homeostasis. Nat Rev Immunol, 14(6): 392–404.
https://doi.org/10.1038/nri3671
Fogg DK, Sibon C, Miled C, et al., 2006, A clonogenic bone marrow progenitor specific for macrophages and dendritic cells. Science (New York), 311(5757): 83–87.
https://doi.org/10.1126/science.1117729
Lee J, Breton G, Oliveira TY, et al., 2015, Restricted dendritic cell and monocyte progenitors in human cord blood and bone marrow. J Exp Med, 212(3): 385–399.
https://doi.org/10.1084/jem.20141442
Menezes S, Melandri D, Anselmi G, et al., 2016, The heterogeneity of Ly6C monocytes controls their differentiation into iNOS macrophages or monocyte-derived dendritic cells. Immunity, 45(6): 1205–1218.
https://doi.org/10.1016/j.immuni.2016.12.001
Gabrilovich DI, Bronte V, Chen SH, et al., 2007, The terminology issue for myeloid–derived suppressor cells. Cancer Res, 67(1): 425; author reply 426.
https://doi.org/10.1158/0008-5472.CAN-06-3037
Veglia F, Perego M, Gabrilovich D, 2018, Myeloid-derived suppressor cells coming of age. Nat Immunol, 19(2): 108–119.
https://doi.org/10.1038/s41590-017-0022-x
Filipazzi P, Valenti R, Huber V, et al., 2007, Identification of a new subset of myeloid suppressor cells in peripheral blood of melanoma patients with modulation by a granulocyte-macrophage colony-stimulation factor-based antitumor vaccine. J Clin Oncol, 25(18): 2546–2553.
https://doi.org/10.1200/JCO.2006.08.5829
Zea AH, Rodriguez PC, Atkins MB, et al., 2005, Arginase-producing myeloid suppressor cells in renal cell carcinoma patients: A mechanism of tumor evasion. Cancer Res, 65(8): 3044–3048.
https://doi.org/10.1158/0008-5472.CAN-04-4505
Srivastava MK, Bosch JJ, Thompson JA, et al., 2008, Lung cancer patients’ CD4(+) T cells are activated in vitro by MHC II cell-based vaccines despite the presence of myeloid-derived suppressor cells. Cancer Immunol Immunother, 57(10): 1493–1504.
https://doi.org/10.1007/s00262-008-0490-9
Hoechst B, Ormandy LA, Ballmaier M, et al., 2008, A new population of myeloid-derived suppressor cells in hepatocellular carcinoma patients induces CD4(+)CD25(+) Foxp3(+) T cells. Gastroenterology, 135(1): 234–243.
https://doi.org/10.1053/j.gastro.2008.03.020
Canè S, Ugel S, Trovato R, et al., 2019, The endless saga of monocyte diversity. Front Immunol, 10: 1786.
https://doi.org/10.3389/fimmu.2019.01786
Cassetta L, Bruderek K, Skrzeczynska-Moncznik J, et al., 2020, Differential expansion of circulating human MDSC subsets in patients with cancer, infection and inflammation. J Immunother Cancer, 8(2): e001223.
https://doi.org/10.1136/jitc-2020-001223
Hartwig T, Montinaro A, von Karstedt S, et al., 2017, The TRAIL-induced cancer secretome promotes a tumor- supportive immune microenvironment via CCR2. Mol Cell, 65(4): 730–742.e5.
https://doi.org/10.1016/j.molcel.2017.01.021
Hettinger J, Richards DM, Hansson J, et al., 2013, Origin of monocytes and macrophages in a committed progenitor. Nat Immunol, 14(8): 821–830.
https://doi.org/10.1038/ni.2638
Kawamura S, Onai N, Miya F, et al., 2017, Identification of a human clonogenic progenitor with strict monocyte differentiation potential: A counterpart of mouse cMoPs. Immunity, 46(5): 835–848.e4.
https://doi.org/10.1016/j.immuni.2017.04.019
Drissen R, Buza-Vidas N, Woll P, et al., 2016, Distinct myeloid progenitor-differentiation pathways identified through single-cell RNA sequencing. Nat Immunol, 17(6): 666–676.
https://doi.org/10.1038/ni.3412
Sasmono RT, Oceandy D, Pollard JW, et al., 2003, A macrophage colony-stimulating factor receptor-green fluorescent protein transgene is expressed throughout the mononuclear phagocyte system of the mouse. Blood, 101(3): 1155–1163.
https://doi.org/10.1182/blood-2002-02-0569
Van Dyken SJ, Locksley RM, 2013, Interleukin-4- and interleukin-13-mediated alternatively activated macrophages: Roles in homeostasis and disease. Annu Rev Immunol, 31: 317–343.
https://doi.org/10.1146/annurev-immunol-032712-095906
Nirschl CJ, Suárez-Fariñas M, Izar B, et al., 2017, IFNγ- dependent tissue-immune homeostasis is co-opted in the tumor microenvironment. Cell, 170(1): 127–141.e115.
https://doi.org/10.1016/j.cell.2017.06.016
Long AH, Highfill SL, Cui Y, et al., 2016, Reduction of MDSCs with all-trans retinoic acid improves CAR therapy efficacy for sarcomas. Cancer Immunol Res, 4(10): 869–880.
https://doi.org/10.1158/2326-6066.CIR-15-0230
Michielon E, González ML, Burm JL, et al., Micro-environmental cross-talk in an organotypic human melanoma-in-skin model directs M2-like monocyte differentiation via IL-10. Cancer Immunol Immunother, 69(11): 2319–2331.
https://doi.org/10.1007/s00262-020-02626-4
Sander J, Schmidt SV, Cirovic B, et al., 2017, Cellular differentiation of human monocytes is regulated by time-dependent interleukin-4 signaling and the transcriptional regulator NCOR2. Immunity, 47(6): 1051–1066.e12.
https://doi.org/10.1016/j.immuni.2017.11.024
Shen CK, Huang BR, Yeh WL, et al., 2021, Regulatory effects of IL-1β in the interaction of GBM and tumor-associated monocyte through VCAM-1 and ICAM-1. Eur J Pharmacol, 905: 174216.
https://doi.org/10.1016/j.ejphar.2021.174216
Franklin RA, Liao W, Sarkar A, et al., 2014, The cellular and molecular origin of tumor-associated macrophages. Science (New York), 344(6186): 921–925.
https://doi.org/10.1126/science.1252510
Jenkins SJ, Ruckerl D, Cook PC, et al., 2011, Local macrophage proliferation, rather than recruitment from the blood, is a signature of TH2 inflammation. Science (New York), 332(6035): 1284–1288.
https://doi.org/10.1126/science.1204351
Orecchioni M, Ghosheh Y, Pramod AB, et al., 2019, Macrophage polarization: Different gene signatures in M1(LPS+) vs. classically and M2(LPS–) vs. alternatively activated macrophages. Front Immunol, 10: 1084.
https://doi.org/10.3389/fimmu.2019.01084
Okabe Y, Medzhitov R, 2014, Tissue-specific signals control reversible program of localization and functional polarization of macrophages. Cell, 157(4): 832–844.
https://doi.org/10.1016/j.cell.2014.04.016
Devalaraja S, To TK, Folkert IW, et al., 2020, Tumor-derived retinoic acid regulates intratumoral monocyte differentiation to promote immune suppression. Cell, 180(6): 1098–1114.e16.
https://doi.org/10.1016/j.cell.2020.02.042
Chun E, Lavoie S, Michaud M, et al., 2015, CCL2 promotes colorectal carcinogenesis by enhancing polymorphonuclear myeloid-derived suppressor cell population and function. Cell Rep, 12(2): 244–257.
https://doi.org/10.1016/j.celrep.2015.06.024
Zhang J, Patel L, Pienta KJ. CC chemokine ligand 2 (CCL2) promotes prostate cancer tumorigenesis and metastasis. Cytokine Growth Factor Rev, 21(1): 41–48.
https://doi.org/10.1016/j.cytogfr.2009.11.009
Ma RY, Zhang H, Li XF, et al., 2020, Monocyte-derived macrophages promote breast cancer bone metastasis outgrowth. J Exp Med, 217(11): e20191820.
https://doi.org/10.1084/jem.20191820
Cortez-Retamozo V, Etzrodt M, Newton A, et al., 2012, Origins of tumor-associated macrophages and neutrophils. Proc Natl Acad Sci U S A, 109(7): 2491–2496.
https://doi.org/10.1073/pnas.1113744109
Yeap WH, Wong KL, Shimasaki N, et al., 2016, CD16 is indispensable for antibody-dependent cellular cytotoxicity by human monocytes. Sci Rep, 6: 34310.
https://doi.org/10.1038/srep34310
Kusmartsev S, Nefedova Y, Yoder D, et al., 2004, Antigen-specific inhibition of CD8+ T cell response by immature myeloid cells in cancer is mediated by reactive oxygen species. J Immunol (Baltimore, Md. 1950), 172(2): 989–999.
https://doi.org/10.4049/jimmunol.172.2.989
Bronte V, Serafini P, Mazzoni A, et al., 2003, L-arginine metabolism in myeloid cells controls T-lymphocyte functions. Trends Immunol, 24(6): 302–306.
https://doi.org/10.1016/s1471-4906(03)00132-7
Baniyash M, 2004, TCR zeta-chain downregulation: Curtailing an excessive inflammatory immune response. Nat Rev Immunol, 4(9): 675–687.
https://doi.org/10.1038/nri1434
Jakubzick CV, Randolph GJ, Henson PM, 2017, Monocyte differentiation and antigen-presenting functions. Nat Rev Immunol, 17(6): 349–362.
https://doi.org/10.1038/nri.2017.28
Ma Y, Adjemian S, Mattarollo SR, et al., 2013, Anticancer chemotherapy-induced intratumoral recruitment and differentiation of antigen-presenting cells. Immunity, 38(4): 729–741.
https://doi.org/10.1038/nri.2017.28
Kuhn S, Yang J, Ronchese F, 2015, Monocyte-derived dendritic cells are essential for CD8(+) T cell activation and antitumor responses after local immunotherapy. Front Immunol, 6: 584.
https://doi.org/10.3389/fimmu.2015.00584
Zhu C, Kros JM, Cheng C, et al., 2017, The contribution of tumor-associated macrophages in glioma neo-angiogenesis and implications for anti-angiogenic strategies. Neuro Oncol, 19(11): 1435–1446.
https://doi.org/10.1093/neuonc/nox081
Chittezhath M, Dhillon MK, Lim JY, et al., 2014, Molecular profiling reveals a tumor-promoting phenotype of monocytes and macrophages in human cancer progression. Immunity, 41(5): 815–829.
https://doi.org/10.1016/j.immuni.2014.09.014
De Palma M, Venneri MA, Roca C, et al, 2003, Targeting exogenous genes to tumor angiogenesis by transplantation of genetically modified hematopoietic stem cells. Nat Med, 9(6): 789–795.
https://doi.org/10.1038/nm871
Shojaei F, Wu X, Malik AK, et al., 2007, Tumor refractoriness to anti-VEGF treatment is mediated by CD11b+Gr1+ myeloid cells. Nat Biotechnol, 25(8): 911–920.
https://doi.org/10.1038/nbt1323
Kaplan RN, Riba RD, Zacharoulis S, et al., 2005, VEGFR1- positive haematopoietic bone marrow progenitors initiate the pre-metastatic niche. Nature, 438(7069): 820–827.
https://doi.org/10.1038/nature04186
Matsubara T, Kanto T, Kuroda S, et al., 2013, TIE2-expressing monocytes as a diagnostic marker for hepatocellular carcinoma correlates with angiogenesis. Hepatology, 57(4): 1416–1425.
https://doi.org/10.1002/hep.25965
Harney AS, Arwert EN, Entenberg D, et al., 2015, Real-time imaging reveals local, transient vascular permeability, and tumor cell intravasation stimulated by TIE2hi macrophage-derived VEGFA. Cancer Discov, 5(9): 932–943.
https://doi.org/10.1158/2159-8290.CD-15-0012
Lu P, Weaver VM, Werb Z, 2012, The extracellular matrix: A dynamic niche in cancer progression. J Cell Biol, 196(4): 395–406.
https://doi.org/10.1083/jcb.201102147
Naba A, Clauser KR, Hoersch S, et al., 2012, The matrisome: In silico definition and in vivo characterization by proteomics of normal and tumor extracellular matrices. Mol Cell Proteomics, 11(4): M111.014647.
https://doi.org/10.1074/mcp.M111.014647
Kessenbrock K, Plaks V, Werb Z, 2010, Matrix metalloproteinases: Regulators of the tumor microenvironment. Cell, 141(1): 52–67.
https://doi.org/10.1016/j.cell.2010.03.015
Mason SD, Joyce JA, 2011, Proteolytic networks in cancer. Trends Cell Biol, 21(4): 228–237.
https://doi.org/10.1016/j.tcb.2010.12.002
Afik R, Zigmond E, Vugman M, et al., 2016, Tumor macrophages are pivotal constructors of tumor collagenous matrix. J Exp Med, 213(11): 2315–2331.
https://doi.org/10.1084/jem.20151193
Madsen DH, Jürgensen HJ, Siersbæk MS, et al., 2017, Tumor-associated macrophages derived from circulating inflammatory monocytes degrade collagen through cellular uptake. Cell Rep, 21(13): 3662–3671.
https://doi.org/10.1016/j.celrep.2017.12.011
Madsen DH, Leonard D, Masedunskas A, et al., 2013, M2-like macrophages are responsible for collagen degradation through a mannose receptor-mediated pathway. J Cell Biol, 202(6): 951–966.
https://doi.org/10.1083/jcb.201301081
Guilliams M, Ginhoux F, Jakubzick C, et al., 2014, Dendritic cells, monocytes and macrophages: A unified nomenclature based on ontogeny. Nat Rev Immunol, 14(8): 571–578.
https://doi.org/10.1038/nri3712
DeNardo DG, Ruffell B, 2019, Macrophages as regulators of tumour immunity and immunotherapy. Nat Rev Immunol, 19(6): 369–382.
https://doi.org/10.1038/s41577-019-0127-6
Zhu Y, Herndon JM, Sojka DK, et al., 2017, Tissue-resident macrophages in pancreatic ductal adenocarcinoma originate from embryonic hematopoiesis and promote tumor progression. Immunity, 47(2): 323–338.e6.
https://doi.org/10.1016/j.immuni.2017.07.014
Sica A, Bronte V, 2007, Altered macrophage differentiation and immune dysfunction in tumor development. J Clin Investig, 117(5): 1155–1166.
https://doi.org/10.1172/JCI31422
Yin M, Li X, Tan S, et al., 2016, Tumor-associated macrophages drive spheroid formation during early transcoelomic metastasis of ovarian cancer. J Clin Investig, 126(11): 4157–4173.
https://doi.org/10.1172/JCI87252
Richards DM, Hettinger J, Feuerer M, 2013, Monocytes and macrophages in cancer: development and functions. Cancer Microenviron, 6(2): 179–191.
https://doi.org/10.1007/s12307-012-0123-x
Martinez FO, Helming L, Gordon S, 2009, Alternative activation of macrophages: An immunologic functional perspective. Annu Rev Immunol, 27: 451–483.
https://doi.org/10.1146/annurev.immunol.021908.132532
Mantovani A, Sozzani S, Locati M, et al., 2002, Macrophage polarization: Tumor-associated macrophages as a paradigm for polarized M2 mononuclear phagocytes. Trends Immunol, 23(11): 549–555.
https://doi.org/10.1016/s1471-4906(02)02302-5
Minopoli M, Sarno S, Di Carluccio G, et al., 2020, Inhibiting monocyte recruitment to prevent the pro-tumoral activity of tumor-associated macrophages in chondrosarcoma. Cells, 9(4): 1062.
https://doi.org/10.3390/cells9041062
Murray PJ, Allen JE, Biswas SK, et al., 2014, Macrophage activation and polarization: nomenclature and experimental guidelines. Immunity, 41(1): 14–20.
https://doi.org/10.1016/j.immuni.2014.06.008
Azizi E, Carr AJ, Plitas G, et al., 2018, Single-cell map of diverse immune phenotypes in the breast tumor microenvironment. Cell, 174()5: 1293–1308.e36.
https://doi.org/10.1016/j.cell.2018.05.060
Vitale I, Shema E, Loi S, et al., 2021, Intratumoral heterogeneity in cancer progression and response to immunotherapy. Nat Med, 27(2): 212–224.
https://doi.org/10.1038/s41591-021-01233-9
Singhal S, Stadanlick J, Annunziata MJ, et al., 2019, Human tumor-associated monocytes/macrophages and their regulation of T cell responses in early-stage lung cancer. Sci Transl Med, 11(479): eaat1500.
https://doi.org/10.1126/scitranslmed.aat1500
Laviron M, Boissonnas A, 2019, Ontogeny of tumor-associated macrophages. Front Immunol, 10: 1799.
Müller S, Kohanbash G, Liu SJ, et al., 2017, Single-cell profiling of human gliomas reveals macrophage ontogeny as a basis for regional differences in macrophage activation in the tumor microenvironment. Genome Biol, 18: 234.
Bowman RL, Klemm F, Akkari L, et al., 2016, Macrophage ontogeny underlies differences in tumor-specific education in brain malignancies. Cell Rep, 17(9): 2445–2459.
https://doi.org/10.1016/j.celrep.2016.10.052
Balkwill F, Mantovani A, 2001, Inflammation and cancer: Back to virchow? Lancet, 357(9255): 539–545.
https://doi.org/10.1016/S0140-6736(00)04046-0
Kumar V, Patel S, Tcyganov E, et al., 2016, The nature of Myeloid-Derived suppressor cells in the tumor microenvironment. Trends Immunol, 37(3): 208–220.
https://doi.org/10.1016/j.it.2016.01.004
Kumar V, Cheng P, Condamine T, et al., 2016, CD45 phosphatase inhibits STAT3 transcription factor activity in myeloid cells and promotes tumor-associated macrophage differentiation. Immunity, 44(2): 303–315.
https://doi.org/10.1016/j.immuni.2016.01.014
Laoui D, Keirsse J, Morias Y, et al., 2016, The tumour microenvironment harbours ontogenically distinct dendritic cell populations with opposing effects on tumour immunity. Nat Commun, 7: 13720.
https://doi.org/10.1038/ncomms13720
Veglia F, Gabrilovich DI, 2017, Dendritic cells in cancer: The role revisited. Curr Opin Immunol, 45: 43–51.
https://doi.org/10.1016/j.coi.2017.01.002
Broz ML, Binnewies M, Boldajipour B, et al., 2014, Dissecting the tumor myeloid compartment reveals rare activating antigen-presenting cells critical for T cell immunity. Cancer Cell, 26(5): 638–652.
https://doi.org/10.1016/j.ccell.2014.09.007
He YF, Wang CQ, Yu Y, et al., 2015, Tie2-expressing monocytes are associated with identification and prognoses of hepatitis B virus related hepatocellular carcinoma after resection. PLoS One, 10(11): e0143657.
https://doi.org/10.1371/journal.pone.0143657
Awad RM, De Vlaeminck Y, Maebe J, et al., 2018, Turn back the TIMe: Targeting tumor infiltrating myeloid cells to revert cancer progression. Front Immunol, 9: 1977.
https://doi.org/10.3389/fimmu.2018.01977
Nywening TM, Wang-Gillam A, Sanford DE, et al., 2016, Targeting tumour-associated macrophages with CCR2 inhibition in combination with FOLFIRINOX in patients with borderline resectable and locally advanced pancreatic cancer: A single-centre, open-label, dose-finding, non-randomised, phase 1b trial. Lancet Oncol, 17(5): 651–662.
https://doi.org/10.1016/S1470-2045(16)00078-4
Bonapace L, Coissieux MM, Wyckoff J, et al., 2014, Cessation of CCL2 inhibition accelerates breast cancer metastasis by promoting angiogenesis. Nature, 515(7525): 130–133.
https://doi.org/10.1038/nature13862
Steggerda SM, Bennett MK, Chen J, et al., 2017, Inhibition of arginase by CB-1158 blocks myeloid cell-mediated immune suppression in the tumor microenvironment. J Immunother. Cancer, 5(1): 101.
https://doi.org/10.1186/s40425-017-0308-4
Nefedova Y, Fishman M, Sherman S, et al., 2007, Mechanism of all-trans retinoic acid effect on tumor-associated myeloid-derived suppressor cells. Cancer Res, 67(22): 11021–11028.
https://doi.org/10.1158/0008-5472.CAN-07-2593
Vincent J, Mignot G, Chalmin F, et al, 2010, 5-fluorouracil selectively kills tumor-associated myeloid-derived suppressor cells resulting in enhanced T cell-dependent antitumor immunity. Cancer Res, 70(8): 3052–3061.
https://doi.org/10.1158/0008-5472.CAN-09-3690
Dempke WC, Fenchel K, Uciechowski P, et al., 2017, Second-and third-generation drugs for immuno-oncology treatment-the more the better? Eur J Cancer, 74: 55–72.
https://doi.org/10.1016/j.ejca.2017.01.001
Mellman I, Coukos G, Dranoff G, 2011, Cancer immunotherapy comes of age. Nature, 480(7378): 480–489.
https://doi.org/10.1038/nature10673
Drake CG, Jaffee E, Pardoll DM, 2006, Mechanisms of immune evasion by tumors. Adv Immunol, 90: 51–81.
https://doi.org/10.1016/S0065-2776(06)90002-9
van der Leun AM, Thommen DS, Schumacher TN, 2020, CD8 T cell states in human cancer: Insights from single-cell analysis. Nat Rev Cancer, 20(4): 218–232.
https://doi.org/10.1038/s41568-019-0235-4
Ribas A, Wolchok JD, 2018, Cancer immunotherapy using checkpoint blockade. Science (New York), 359(6382): 1350– 1355.
https://doi.org/10.1126/science.aar4060
Keir ME, Butte MJ, Freeman GJ, et al., 2008, PD-1 and its ligands in tolerance and immunity. Annu Rev Immunol, 26: 677–704.
https://doi.org/10.1146/annurev.immunol.26.021607.090331
Topalian SL, Drake CG, Pardoll DM, 2015, Immune checkpoint blockade: A common denominator approach to cancer therapy. Cancer Cell, 27(4): 450–461.
https://doi.org/10.1016/j.ccell.2015.03.001
Schetters ST, Rodriguez E, Kruijssen LJ, et al., 2020, Monocyte-derived APCs are central to the response of PD1 checkpoint blockade and provide a therapeutic target for combination therapy. J Immunother Cancer, 8(2): e000588.
https://doi.org/10.1136/jitc-2020-000588
Hugo W, Zaretsky JM, Sun L, et al., 2016, Genomic and transcriptomic features of response to anti-PD-1 therapy in metastatic melanoma. Cell, 165(1): 35–44.
https://doi.org/10.1016/j.cell.2016.02.065
Hamid O, Robert C, Daud A, et al., 2013, Safety and tumor responses with lambrolizumab (anti-PD-1) in melanoma. N Engl J Med, 369(2): 134–144.
https://doi.org/10.1056/NEJMoa1305133
Das S, Camphausen K, Shankavaram U, 2020, Cancer-specific immune prognostic signature in solid tumors and its relation to immune checkpoint therapies. Cancers, 12(9): 2476.
https://doi.org/10.3390/cancers12092476
Marcovecchio PM, Thomas G, Salek-Ardakani S, 2021, CXCL9-expressing tumor-associated macrophages: New players in the fight against cancer. J Immunother Cancer, 9(2): e002045.
https://doi.org/10.1136/jitc-2020-002045
Huber V, Di Guardo L, Lalli L, et al., 2021, Back to simplicity: A four-marker blood cell score to quantify prognostically relevant myeloid cells in melanoma patients. J Immunother Cancer, 9(2): e001167.
https://doi.org/10.1136/jitc-2020-001167
Gordon SR, Maute RL, Dulken BW, et al., 2017, PD-1 expression by tumour-associated macrophages inhibits phagocytosis and tumour immunity. Nature, 545(7655): 495–499.
https://doi.org/10.1038/nature22396
Zou W, Wolchok JD, Chen L, 2016, PD-L1 (B7-H1) and PD-1 pathway blockade for cancer therapy: Mechanisms, response biomarkers, and combinations. Sci Transl Med, 8(328): 328–324.
https://doi.org/10.1126/scitranslmed.aad7118
Liu M, Zhou J, Liu X, et al., 2020, Targeting monocyte-intrinsic enhancer reprogramming improves immunotherapy efficacy in hepatocellular carcinoma. Gut, 69(2): 365–379.
https://doi.org/10.1136/gutjnl-2018-317257
Escobar G, Barbarossa L, Barbiera G, et al., 2018, Interferon gene therapy reprograms the leukemia microenvironment inducing protective immunity to multiple tumor antigens. Nat Commun, 9: 2896.
Gubin MM, Esaulova E, Ward JP, et al., 2018, High-dimensional analysis delineates myeloid and lymphoid compartment remodeling during successful immune-checkpoint cancer therapy. Cell, 175(4): 1443.
https://doi.org/10.1016/j.cell.2018.09.030
Yin M, Guo Y, Hu R, et al., 2020, Potent BRD4 inhibitor suppresses cancer cell-macrophage interaction. Nat Commun, 11(1): 1833.
https://doi.org/10.1038/s41467-020-15290-0
June CH, Sadelain M, 2018, Chimeric antigen receptor therapy. N Engl J Med, 379(1): 64–73.
https://doi.org/10.1056/NEJMra1706169
Green DS, Nunes AT, David-Ocampo V, et al., 2018, A phase 1 trial of autologous monocytes stimulated ex vivo with sylatron (peginterferon alfa-2b) and actimmune (interferon gamma-1b) for intra-peritoneal administration in recurrent ovarian cancer. J Transl Med, 16(1): 196.
https://doi.org/10.1186/s12967-018-1569-5
Liu Y, Cao X, 2015, The origin and function of tumor-associated macrophages. Cell Mol Immunol, 12: 1–4.
https://doi.org/10.1038/cmi.2014.83
Noy R, Pollard JW, 2014, Tumor-associated macrophages: From mechanisms to therapy. Immunity, 41(1): 49–61.
https://doi.org/10.1016/j.immuni.2014.06.010