A conceptual model of the role of infectious agents in autoimmune diseases
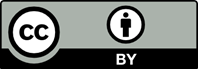
The correlation between microbes and autoimmunity is well established, but many underlying mechanisms remain obscure. Thus, this paper attempts to elucidate the role of infectious agents (bacteria, viruses, etc.) in autoimmune diseases. To offer a concise framework for many relevant research findings, the following general conceptual model is proposed and discussed: autoimmune diseases arise from alterations in cells, tissues, or organs, caused by infectious agents. These alterations evolve with time, beginning as subtle, often undetectable changes. As the alterations become more severe, they can be identified by the immune system, which may subsequently attack the infected cells. This process allows for new explanations of relationships between triggers of autoimmunity and infectious agents, the time lag between infection and autoimmune response, the progressive nature of autoimmune diseases, and the role of virus persistence. It can also offer a new point of view on molecular mimicry and epitope spreading. The roles of genetic predisposition, sex, stress, dietary habits, the “hygiene hypothesis,” and the healing effects of β-interferon also fit into this framework. In addition, the side effects of malignancy treatments using immune checkpoint inhibitors can also be explained. Adhering to the framework, it is concluded that treatments should aim to eliminate the cause of these evolving alterations, namely, the infectious agents. Presumably, they could be based on antibiotics and antiviral drugs. Future research directions are suggested for evaluating the proposed conceptual model.

- Rose N, Mackay I, editors. The Autoimmune Diseases. United States: Elsevier Academic Press; 2020.
- Rose NR, Bona C. Defining criteria for autoimmune diseases (Witebsky’s postulates revisited). Immunol Today. 1993;14:426-430. doi: 10.1016/0167-5699(93)90244-F
- Theofilopoulos A, Kono D, Baccala R. The multiple pathways to autoimmunity. Nat Immunol. 2017;18:716-24. doi: 10.1038/ni.3731.
- Meffre E, O’Connor KC. Impaired B-cell tolerance checkpoints promote the development of autoimmune diseases and pathogenic autoantibodies. Immunol Rev. 2019;292:90-101. doi: 10.1111/imr.12821
- Pisetsky DS. Pathogenesis of autoimmune disease. Nat Rev Nephrol. 2023;19:509-524. doi: 10.1038/s41581-023-00720-1
- Rosenblum MD, Remedios KA, Abbas AK. Mechanisms of human autoimmunity. J Clin Invest. 2015;125(6):216-228. doi: 10.1172/JCI78088.
- Stojanovich, L. Stress and autoimmunity. Autoimmun Rev. 2010;9(5):A271-A276. doi: 10.1016/j.autrev.2009.11.014
- Bluestone JA, Anderson M. Tolerance in the age of immunotherapy. N Engl J Med. 2020;383:1156-1166. doi: 10.1056/NEJMra1911109
- Rałowska-Gmoch W, Koszewicz M, Łabuz-Roszak B, Budrewicz S, Dziadkowiak E. Diagnostic criteria and therapeutic implications of rapid-onset demyelinating polyneuropathies. Exp Mol Pathol. 2024;140:04942. doi: 10.1016/j.yexmp.2024.104942
- Ercolini AM, Miller SD. The role of infections in autoimmune disease. Clin Exp Immunol. 2009;155(1):1-15. doi: 10.1111/j.1365-2249.2008.03834.x
- Fairweather D, Kaya Z, Shellam GR, Lawson CM, Rose NR. From infection to autoimmunity. J Autoimmun. 2001;16(3):175-186. doi: 10.1006/jaut.2000.0492
- Rojas M, Restrepo-Jiménez P, Monsalve DM, et al. Molecular mimicry and autoimmunity. J Autoimmun. 2018;95:100-123. doi: 10.1016/j.jaut.2018.10.012
- Dotta F, Censini S, Van Halteren AG, et al. Coxsackie B4 virus infection of beta cells and natural killer cell insulitis in recent-onset type 1 diabetic patients. Proc Natl Acad Sci U S A. 2007;104:5115-5120. doi: 10.1073/pnas.0700442104
- Lossius A, Johansen JN, Torkildsen Ø, Vartdal F, Holmøy T. Epstein-barr virus in systemic lupus erythematosus, rheumatoid arthritis and multiple sclerosis-association and causation. Viruses. 2012;4(12):3701-3730. doi: 10.3390/v4123701
- Sher EK, Ćosović A, Džidić-Krivić A, Farhat EK, Pinjić E, Sher F. Covid-19 a triggering factor of autoimmune and multi-inflammatory diseases. Life Sci. 2023;319:121531. doi: 10.1016/j.lfs.2023.121531
- Vellozzi C, Iqbal S, Broder K. Guillain-Barre syndrome, influenza, and influenza vaccination: The epidemiologic evidence. Clin Infect Dis. 2014;58:1149-1155. doi: 10.1093/cid/ciu005
- Polykretis P, Donzelli A, Lindsay JC, et al. Autoimmune inflammatory reactions triggered by the COVID-19 genetic vaccines in terminally differentiated tissues. Autoimmunity. 2023;56(1):2259123. doi: 10.1080/08916934.2023.2259123
- Rojas M, Herrán M, Ramírez-Santana C, et al. Molecular mimicry and autoimmunity in the time of COVID-19. J Autoimmun. 2023;139:103070. doi: 10.1016/j.jaut.2023.103070
- Cardoso F. Sydenham’s chorea. Curr Treat Options Neurol. 2008;10:230-5. doi: 10.1007/s11940-008-0025-x
- Bonthius DJ, Karacay B. Sydenham’s chorea: Not gone and not forgotten. Semin Pediatr Neurol. 2003;10:11-19. doi: 10.1016/S1071-9091(02)00004-9
- Van Toorn R, Weyers HH, Schoeman JF. Distinguishing PANDAS from Sydenham’s chorea: Case report and review of the literature. Eur J Paediatr Neurol. 2004;8:211-216. doi: 10.1016/j.ejpn.2004.03.005
- Axelrad JE, Cadwell KH, Colombel JF, Shah SC. The role of gastrointestinal pathogens in inflammatory bowel disease: A systematic review. Ther Adv Gastroenter. 2021;14:17562848211004493. doi: 10.1177/17562848211004493
- Wang M, Wu L, Weng R, Zheng W, Wu Z, Lv Z. Therapeutic potential of helminths in autoimmune diseases: Helminth-derived immune-regulators and immune balance. Parasitol Res. 2017;116:2065-2074. doi: 10.1007/s00436-017-5544-5
- Shukla SK, Govind Singh G, Ahmad S, Pant P. Infections, genetic and environmental factors in pathogenesis of autoimmune thyroid diseases. Microb Pathog. 2018;116:279-288. doi: 10.1016/j.micpath.2018.01.004
- Von Herrath M, Fujinami R, Whitton J. Microorganisms and autoimmunity: Making the barren field fertile? Nat Rev Microbiol. 2003;1:151-157. doi: 10.1038/nrmicro754
- Domingues TD, Malato J, Grabowska AD, et al. Association analysis between symptomology and herpesvirus IgG antibody concentrations in myalgic encephalomyelitis/ chronic fatigue syndrome (ME/CFS) and multiple sclerosis. Heliyon. 2023;9(7):e18250. doi: 10.1016/j.heliyon.2023.e18250
- Libbey JE, Cusick MF, Fujinami RS. Role of pathogens in multiple sclerosis. Int Rev Immunol. 2014;33:266-283. doi: 10.3109/08830185.2013.823422
- Willison HJ, Jacobs BC, Van Doorn PA. Guillain-Barré syndrome. Lancet. 2016;388(10045):717-727. doi: 10.1016/S0140-6736(16)00339-1
- Maślińska M. The role of Epstein-Barr virus infection in primary Sjögren’s syndrome. Curr Opin Rheumatol. 2019;31(5):475-483. doi: 10.1097/BOR.0000000000000622
- Wang Y, Dou H, Liu G, et al. Hepatitis C virus infection and the risk of Sjögren or sicca syndrome: A meta-analysis. Microbiol Immunol. 2014;58:675-687. doi: 10.1111/1348-0421.12202
- Battistini C, Ballan R, Herkenhoff ME, Saad SM, Sun J. Vitamin D modulates intestinal microbiota in inflammatory bowel diseases. Int J Mol Sci. 2021;22(1):362. doi: 10.3390/ijms22010362
- Shim CH, Cho S, Shin YM, Choi JM. Emerging role of bystander T cell activation in autoimmune diseases. BMB Rep. 2022;55(2):57-64. doi: 10.5483/BMBRep.2022.55.2.183
- Fujinami RS, Von Herrath MG, Christen U, Whitton JL. Molecular mimicry, bystander activation, or viral persistence: Infections and autoimmune disease. Clin Microbiol Rev. 2006;19(1):80-94. doi: 10.1128/cmr.19.1.80-94.2006
- Frazer IH. Autoimmunity and persistent viral infection: Two sides of the same coin? J Autoimmun. 2008;31(3):216-218. doi: 10.1016/j.jaut.2008.04.014
- Smatti MK, Cyprian FS, Nasrallah GK, Al Thani AA, Almishal RO, Yassine HM. Viruses and autoimmunity: A review on the potential interaction and molecular mechanisms. Viruses. 2019;11(8):762. doi: 10.3390/v11080762
- Oldstone MBA. Molecular mimicry, microbial infection, and autoimmune disease: Evolution of the concept, in molecular mimicry: Infection-inducing autoimmune disease. In: Oldstone, MBA, editor. Current Topics in Microbiology and Immunology. Berlin, Heidelberg: Springer; 2005. p. 296. doi: 10.1007/3-540-30791-5_1
- Stewart L, Edgar JDM, Blakely G, Patric S. Antigenic mimicry of ubiquitin by the gut bacterium Bacteroides fragilis: A potential link with autoimmune disease. Clin Exp Immunol. 2018;194(2):153-165. doi: 10.1111/cei.13195
- Suliman BA. Potential clinical implications of molecular mimicry-induced autoimmunity. Immun Inflamm Dis. 2024;12:e1178. doi: 10.1002/iid3.1178
- Maguire C, Wang C, Ramasamy A, et al. Molecular mimicry as a mechanism of viral immune evasion and autoimmunity. Nat Commun. 2024;15:9403. doi: 10.1038/s41467-024-53658-8
- Würzner, R. Evasion of pathogens by avoiding recognition or eradication by complement, in part via molecular mimicry. Mol Immunol. 1999;36(4-5):249-260. doi: 10.1016/S0161-5890(99)00049-8
- Vanderlugt CJ, Miller SD. Epitope spreading. Curr Opin Immunol. 1996;8:831-836. doi: 10.1016/S0952-7915(96)80012-4
- Venkatesha SH, Durai M, Moudgil KD. Epitope spreading in autoimmune diseases. In: Mahroum N, Watad A, Shoenfeld Y, editors. Infection and Autoimmunity. 3rd ed., Ch. 5. Academic Press; 2024. p61-89. doi: 10.1016/B978-0-323-99130-8.00038-6
- Cornaby C, Gibbons L, Mayhew V, Sloan CS, Welling A, Poole BD. B cell epitope spreading: Mechanisms and contribution to autoimmune diseases. Immunol Lett. 2015;163(1):56-68. doi: 10.1016/j.imlet.2014.11.001
- Long H, Yin H, Wang L, Gershwin ME, Lu Q. The critical role of epigenetics in systemic lupus erythematosus and autoimmunity. J Autoimmun. 2016;74:118-138. doi: 10.1016/j.jaut.2016.06.020
- Kahaly GJ, Hansen MP. Type 1 diabetes associated autoimmunity. Autoimmun Rev. 2016;15:644-648. doi: 10.1016/j.autrev.2016.02.017
- Ma WT, Chang CH, Gershwin ME, Lian ZX. Development of autoantibodies precedes clinical manifestations of autoimmune diseases: A comprehensive review. J Autoimmun. 2017;83:95-112. doi: 10.1016/j.jaut.2017.07.003
- Criswell LA, Pfeiffer KA, Lum RF, et al. Analysis of families in the multiple autoimmune disease genetics consortium (MADGC) collection: The PTPN22 620W allele associates with multiple autoimmune phenotypes. Am J Hum Genet. 2005;76(4):561-571. doi: 10.1086/429096
- Li J, Lin SY, Lv YB, Tang HM, Peng F. Association study of MMP-9 -1562C/T gene polymorphism with susceptibility to multiple autoimmune diseases: A meta-analysis. Arch Med Res. 2017;48(1):105-112. doi: 10.1016/j.arcmed.2017.01.001
- Owen KA, Price A, Ainsworth H, et al. Analysis of trans-ancestral SLE risk loci identifies unique biologic networks and drug targets in African and European ancestries. Am J Hum Genet. 2020;107(5):864-881. doi: 10.1016/j.ajhg.2020.09.007
- Fairweather D, Beeler DJ, McCabe EJ, Lieberman SM. Mechanisms underlying sex differences in autoimmunity. J Clin Invest. 2024;134(18):e180076. doi: 10.1172/JCI180076
- Fischinger S, Boudreau CM, Butler AL, Streeck H, Alter G. Sex differences in vaccine-induced humoral immunity. Semin Immunopathol. 2019;41:239-249. doi: 10.1007/s00281-018-0726-5
- Raine C, Austin K, Giles I. Mechanisms determining the amelioration of rheumatoid arthritis in pregnancy: A systematic review. Semin Arthritis Rheum. 2020;50(6):1357-1369. doi: 10.1016/j.semarthrit.2020.03.006
- Lerner A, Matthias T. Changes in intestinal tight junction permeability associated with industrial food additives explain the rising incidence of autoimmune disease. Autoimmun Rev. 2015;14:479-489. doi: 10.1016/j.autrev.2015.01.009
- Murdaca G, Tonacci A, Negrini S, et al. Emerging role of vitamin D in autoimmune diseases: An update on evidence and therapeutic implications. Autoimmun Rev. 2019;18(9):102350. doi: 10.1016/j.autrev.2019.102350
- Guggenmos J, Schubart AS, Ogg S, et al. Antibody cross-reactivity between myelin oligodendrocyte glycoprotein and the milk protein butyrophilin in multiple sclerosis. J Immunol. 2004;172:661-668. doi: 10.4049/jimmunol.172.1.661
- Armelagos GJ, Brown PJ, Turner B. Evolutionary, historical and political economic perspectives on health and disease. Soc Sci Med. 2005;61(4):755-765. doi: 10.1016/j.socscimed.2004.08.066
- Okada H, Kuhn C, Feillet H, Bach JF. The ‘hygiene hypothesis’ for autoimmune and allergic diseases: An update. Clin Exp Immunol. 2010;160(1):1-9. doi: 10.1111/j.1365-2249.2010.04139.x
- Murdaca G, Greco M, Borro M, Gangemi S. Hygiene hypothesis and autoimmune diseases: A narrative review of clinical evidences and mechanisms. Autoimmun Rev. 2021;20(7):102845. doi: 10.1016/j.autrev.2021.102845
- Pruett SB. Stress and the immune system. Pathophysiology. 2003;9(3):133-153. doi: 10.1016/S0928-4680(03)00003-8
- Jacobs R, Pawlak CR, Mikeska E, et al. Systemic lupus erythematosus and rheumatoid arthritis patients differ from healthy controls in their cytokine pattern after stress exposure. Rheumatology. 2001;40(8):868-875. doi: 10.1093/rheumatology/40.8.868
- Mitsonis CI, Potagas C, Zervas I, Sfagos K. The effects of stressful life events on the course of multiple sclerosis: A review. Int J Neurosci. 2009;119(3):315-335. doi: 10.1080/00207450802480192
- Song H, Fang F, Tomasson G, et al. Association of stress-related disorders with subsequent autoimmune disease. JAMA. 2018;319(23):2388-2400. doi: 10.1001/jama.2018.7028
- Dhib-Jalbut S, Marks S. Interferon-beta mechanisms of action in multiple sclerosis. Neurology. 2010;74(1 Suppl):S17-S24. doi: 10.1212/WNL.0b013e3181c97d99
- Hojati Z, Kay M, Dehghanian F. Mechanism of action of interferon beta in treatment of multiple sclerosis. In: Minagar A, editor. Multiple Sclerosis. Ch. 15. United States: Academic Press; 2016. p. 365-392. doi: 10.1016/B978-0-12-800763-1.00015-4
- Zhu YX, Li ZY, Yu ZL, et al. The underlying mechanism and therapeutic potential of IFNs in viral-associated cancers. Life Sci. 2025;361:123301. doi: 10.1016/j.lfs.2024.123301
- Xiao X, Chang C. Diagnosis and classification of drug-induced autoimmunity (DIA). J Autoimmun. 2014;48-49:66-72. doi: 10.1016/j.jaut.2014.01.005
- Graus F, Dalmau J. Paraneoplastic neurological syndromes in the era of immune-checkpoint inhibitors. Nat Rev Clin Oncol. 2019;16:535-548. doi: 10.1038/s41571-019-0194-4
- Möhn N, Beutel G, Gutzmer R, et al. Neurological immune related adverse events associated with nivolumab, ipilimumab, and pembrolizumab therapy-review of the literature and future outlook. J Clin Med. 2019;8(11):1777. doi: 10.3390/jcm8111777
- Cano-Cruz LG, Barrera-Vargas A, Mateos-Soria A, Soto- Perez-de-Celis E, Merayo-Chalico J. Rheumatological adverse events of cancer therapy with immune checkpoint inhibitors. Arch Med Res. 2022;53(2):113-121. doi: 10.1016/j.arcmed.2021.09.004
- Zhai Y, Moosavi R, Chen M. Immune checkpoints, a novel class of therapeutic targets for autoimmune diseases. Front Immunol. 2021;12:645699. doi: 10.3389/fimmu.2021.645699