Current materials for 3D-printed flexible medical electrodes
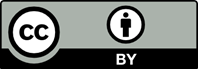
Electrodes serve as essential tools for both acquiring and stimulating electrical signals, pivotal in monitoring human health through electrophysiological signals and playing a significant role in disease management and treatment. Notably, Young’s modulus of flexible electrodes is similar to that of tissues and organs, thereby avoiding tissue or organ damage arising from mechanical mismatch. Thus, flexible electrodes become the fundamental devices for ensuring the stable, long-term acquisition of electrical signals and delivering reversed electrical stimulation to guide disease treatment. Reducing the size of flexible electrodes and increasing the number of electrode channels are significant for improving the sensitivity and accuracy of signal acquisition. In comparison to traditional manufacturing methods, 3D printing technology is able to fabricate products with higher resolution at a much faster speed. It is customizable and provides a novel approach for preparing flexible electrodes. Many conductive materials have been developed and applied to prepare flexible electrodes, and some have been integrated into 3D printing techniques, driving forward the development of 3D-printed flexible electrodes in medical fields. This article reviews recent research advances concerning the combination of these materials with 3D printing technology to prepare flexible electrodes and categorizes the materials into four main groups, namely metallic materials, carbon-based materials, conductive polymers, and other materials. In addition, we outline the future directions regarding the application of 3D-printed flexible electrodes in clinical research and medical translation.
- Metzger SL, Littlejohn KT, Silva AB, et al., 2023, A high-performance neuroprosthesis for speech decoding and avatar control. Nature, 620:1037–1046. https://doi.org/10.1038/s41586-023-06443-4
- Alagapan S, Choi KS, Heisig S, et al., 2023, Cingulate dynamics track depression recovery with deep brain stimulation. Nature, 622:130–138. https://doi.org/10.1038/s41586-023-06541-3
- Jang J, Kim J, Shin H, et al., 2021, Smart contact lens and transparent heat patch for remote monitoring and therapy of chronic ocular surface inflammation using mobiles. Sci Adv, 7: eabf7194. https://doi.org/10.1126/sciadv.abf7194
- Lee GH, Kang H, Chung JW, et al., 2022, Stretchable PPG sensor with light polarization for physical activity-permissible monitoring. Sci Adv, 8: eabm3622. https://doi.org/10.1126/sciadv.abm3622
- Li J, Liu Y, Yuan L, et al., 2022, A tissue-like neurotransmitter sensor for the brain and gut. Nature, 606:94–101. https://doi.org/10.1038/s41586-022-04615-2
- Yang Q, Yang S, Qiu P, et al., 2022, Flexible thermoelectrics based on ductile semiconductors. Science, 377:854–858. https://doi.org/10.1126/science.abq0682
- Hu H, Huang H, Li M, et al., 2023, A wearable cardiac ultrasound imager. Nature, 613:667–675. https://doi.org/10.1038/s41586-022-05498-z
- Flesher SN, Downey JE, Weiss JM, et al., 2021, A brain-computer interface that evokes tactile sensations improves robotic arm control. Science, 372:831–836. https://doi.org/10.1126/science.abd0380
- Pearre BW, Michas C, Tsang JM, et al., 2019, Fast micron-scale 3D printing with a resonant-scanning two-photon microscope. Addit Manuf, 30:100887. https://doi.org/10.1016/j.addma.2019.100887
- Trotter M, Juric D, Bagherian Z, et al., 2020, Inkjet-printing of nanoparticle gold and silver ink on cyclic olefin copolymer for DNA-sensing applications. Sensors, 20:1333. https://doi.org/10.3390/s20051333
- Im J, Trindade GF, Quach TT, et al., 2022, Functionalized gold nanoparticles with a cohesion enhancer for robust flexible electrodes. ACS Appl Nano Mater, 5:6708–6716. https://doi.org/10.1021/acsanm.2c00742
- Lin Z, Jiang T, Shang J, 2022, The emerging technology of biohybrid micro-robots: A review. Biodes Manuf, 5:107–132. https://doi.org/10.1007/s42242-021-00135-6
- Adewole DO, Struzyna LA, Burrell JC, et al., 2021, Development of optically controlled “living electrodes” with long-projecting axon tracts for a synaptic brain-machine interface. Sci Adv, 7: eaay5347. https://doi.org/10.1126/sciadv.aay5347
- Steinmetz NA, Aydin C, Lebedeva A, et al., 2021, Neuropixels 2.0: A miniaturized high-density probe for stable, long-term brain recordings. Science, 372: eabf4588. https://doi.org/10.1126/science.abf4588
- Guo H, Wei Q, Wu Y, et al., 2023, Enhanced nitrate removal from groundwater using a conductive spacer in flow-electrode capacitive deionization. Chin Chem Lett, 109325. https://doi.org/10.1016/j.cclet.2023.109325
- Zhao ET, Hull JM, Mintz Hemed N, et al., 2023, A CMOS-based highly scalable flexible neural electrode interface. Sci Adv, 9: eadf9524. https://doi.org/10.1126/sciadv.adf9524
- Wang Z, Bai H, Yu W, et al., 2022, Flexible bioelectronic device fabricated by conductive polymer-based living material. Sci Adv, 8: eabo1458. https://doi.org/10.1126/sciadv.abo1458
- Larson NM, Mueller J, Chortos A, et al., 2023, Rotational multimaterial printing of filaments with subvoxel control. Nature, 613:682–688. https://doi.org/10.1038/s41586-022-05490-7
- Zeng C, Faaborg MW, Sherif A, et al., 2022, 3D-printed machines that manipulate microscopic objects using capillary forces. Nature, 611:68–73. https://doi.org/10.1038/s41586-022-05234-7
- Sanders SN, Schloemer TH, Gangishetty MK, et al., 2022, Triplet fusion upconversion nanocapsules for volumetric 3D printing. Nature, 604:474–478. https://doi.org/10.1038/s41586-022-04485-8
- Regehly M, Garmshausen Y, Reuter M, et al., 2020, Xolography for linear volumetric 3D printing. Nature, 588:620–624. https://doi.org/10.1038/s41586-020-3029-7
- Schmidt C, 2021, The rise of the assembloid. Nature, 597: S22–S23. https://doi.org/10.1038/d41586-021-02628-x
- Lawlor KT, Vanslambrouck JM, Higgins JW, et al., 2021, Cellular extrusion bioprinting improves kidney organoid reproducibility and conformation. Nat Mater, 20:260–271. https://doi.org/10.1038/s41563-020-00853-9
- Yi HG, Jeong YH, Kim Y, et al., 2019, A bioprinted human-glioblastoma-on-a-chip for the identification of patient-specific responses to chemoradiotherapy. Nat Biomed Eng, 3:509–519. https://doi.org/10.1038/s41551-019-0363-x
- Wang J, Lu T, Yang M, et al., 2019, Hydrogel 3D printing with the capacitor edge effect. Sci Adv, 5: eaau8769. https://doi.org/10.1126/sciadv.aau8769
- Browne MP, Mills A, 2018, Determining the importance of the electrode support and fabrication method during the initial screening process of an active catalyst for the oxygen evolution reaction. J Mater Chem A, 6:14162–14169. https://doi.org/10.1039/C8TA02908C
- Benck JD, Pinaud BA, Gorlin Y, et al., 2014, Substrate selection for fundamental studies of electrocatalysts and photoelectrodes: Inert potential windows in acidic, neutral, and basic electrolyte. PLoS One, 9: e107942. https://doi.org/10.1371/journal.pone.0107942
- Song Y, Tay RY, Li J, et al., 2023, 3D-printed epifluidic electronic skin for machine learning-powered multimodal health surveillance. Sci Adv, 9: eadi6492. https://doi.org/10.1126/sciadv.adi6492
- Zeng L, Ling S, Du D, et al., 2023, Direct ink writing 3D printing for high‐performance electrochemical energy storage devices: A minireview. Adv Sci (Weinh), 10:2303716. https://doi.org/10.1002/advs.202303716
- Huang H, Liao L, Lin Z, et al., 2023, Direct ink writing of pickering emulsions generates ultralight conducting polymer foams with hierarchical structure and multifunctionality. Small, 19:2301493. https://doi.org/10.1002/smll.202301493
- Kotlarz M, Ferreira AM, Gentile P, et al., 2022, Droplet-based bioprinting enables the fabrication of cell-hydrogel-microfibre composite tissue precursors. Biodes Manuf, 5:512–528. https://doi.org/10.1007/s42242-022-00192-5
- Lee J, So H, 2023, 3D-printing-assisted flexible pressure sensor with a concentric circle pattern and high sensitivity for health monitoring. Microsyst Nanoeng, 9:44. https://doi.org/10.1038/s41378-023-00509-z
- Foster CW, Down MP, Zhang Y, et al., 2017, 3D printed graphene based energy storage devices. Sci Rep, 7:42233. https://doi.org/10.1038/srep42233
- Parra-Cabrera C, Achille C, Kuhn S, et al., 2018, 3D printing in chemical engineering and catalytic technology: Structured catalysts, mixers and reactors. Chem Soc Rev, 47:209–230. https://doi.org/10.1039/C7CS00631D
- Browne MP, Redondo E, Pumera M, 2020, 3D printing for electrochemical energy applications. Chem Rev, 120:2783–2810. https://doi.org/10.1021/acs.chemrev.9b00783
- Tanzi MC, Farè S, Candiani G, 2019, Manufacturing technologies. In: Tanzi MC, Farè S, Candiani G, editors. Foundations of Biomaterials Engineering. Ch. 3. Cambridge: Academic Press. p. 137–196. https://doi.org/10.1016/B978-0-08-101034-1.00003-7
- He Q, Wang Z, Wang Y, et al., 2021, Electrospun liquid crystal elastomer microfiber actuator. Sci Robot, 6: eabi9704. https://doi.org/10.1126/scirobotics.abi9704
- Cao F, Guo S, Ma H, et al., 2011, Nickel oxide microfibers immobilized onto electrode by electrospinning and calcination for nonenzymatic glucose sensor and effect of calcination temperature on the performance. Biosens Bioelectron, 26:2756–2760. https://doi.org/10.1016/j.bios.2010.10.013
- Wang X, Xiang H, Song C, et al., 2020, Highly efficient transparent air filter prepared by collecting-electrode-free bipolar electrospinning apparatus. J Hazard Mater, 385:121535. https://doi.org/10.1016/j.jhazmat.2019.121535
- Kim C, An K, Kang M, et al., 2022, Facile fabrication of flexible metal grid transparent electrode using inkjet-printed dot array as sacrificial layer. Sci Rep, 12:1572. https://doi.org/10.1038/s41598-022-05312-w
- Wei Z, Chen H, Yan K, et al., 2014. Inkjet printing and instant chemical transformation of a CH3NH3PbI3/nanocarbon electrode and interface for planar perovskite solar cells. Angew Chem Int Ed Engl, 53:13239–13243. https://doi.org/10.1002/anie.201408638
- Liu Z, Baluchová S, Brocken B, et al., 2023, Inkjet printing-manufactured boron-doped diamond chip electrodes for electrochemical sensing purposes. ACS Appl Mater Interfaces, 15:39915–39925. https://doi.org/10.1021/acsami.3c04824
- Yin J, Zhao D, Liu J, 2019, Trends on physical understanding of bioink printability. Biodes Manuf, 2:50–54. https://doi.org/10.1007/s42242-019-00033-y
- Chen X, Sun M, Jaber F, et al., 2023, A flexible wearable self-supporting hybrid supercapacitor device based on hierarchical nickel cobalt sulfide@C electrode. Sci Rep, 13:15555. https://doi.org/10.1038/s41598-023-42278-9
- Xu Y, Lin Z, Wei W, et al., 2022, Recent progress of electrode materials for flexible perovskite solar cells. Nanomicro Lett, 14:117. https://doi.org/10.1007/s40820-022-00859-9
- Cabrera L, Sadle C, Purcell E, 2019, Neuroethical considerations of high-density electrode arrays. Nat Biomed Eng, 3:586–589. https://doi.org/10.1038/s41551-019-0407-2
- Bennett C, Álvarez-Ciara A, Franklin M, et al., 2021, The complement cascade at the Utah microelectrode-tissue interface. Biomaterials, 268:120583. https://doi.org/10.1016/j.biomaterials.2020.120583
- Soscia DA, Lam D, Tooker AC, et al., 2020, A flexible 3-dimensional microelectrode array for in vitro brain models. Lab Chip, 20:901–911. https://doi.org/10.1039/C9LC01148J
- Conway CR, Olin BD, Aaronson ST, et al., 2021, A prospective, multi-center randomized, controlled, blinded trial of vagus nerve stimulation for difficult to treat depression: A novel design for a novel treatment. Brain Stimul, 14:1666. https://doi.org/10.1016/j.brs.2021.10.247
- Frederick RA, Meliane IY, Joshi-Imre A, et al., 2020, Activated iridium oxide film (AIROF) electrodes for neural tissue stimulation. J Neural Eng, 17:056001. https://doi.org/10.1088/1741-2552/abb9bf
- Musk E, Neuralink, 2019, An integrated brain-machine interface platform with thousands of channels. J Med Internet Res, 21: e16194. https://doi.org/10.2196/16194
- Hui Y, Yao Y, Qian Q, et al., 2022, Three-dimensional printing of soft hydrogel electronics. Nat Electron, 5:893–903. https://doi.org/10.1038/s41928-022-00887-8
- Ma S, Dahiya AS, Dahiya R, 2023, Out-of-plane electronics on flexible substrates using inorganic nanowires grown on high-aspect-ratio printed gold micropillars. Adv Mater, 35: e2210711. https://doi.org/10.1002/adma.202210711
- Saleh MS, Ritchie SM, Nicholas MA, et al., 2022, CMU array: A 3D nanoprinted, fully customizable high-density microelectrode array platform. Sci Adv, 8: eabj4853. https://doi.org/10.1126/sciadv.abj4853
- Brown MA, Zappitelli KM, Singh L, et al., 2023, Direct laser writing of 3D electrodes on flexible substrates. Nat Commun, 14:3610. https://doi.org/10.1038/s41467-023-39152-7
- Kamyshny A, Magdassi S, 2019, Conductive nanomaterials for 2D and 3D printed flexible electronics. Chem Soc Rev, 48:1712–1740. https://doi.org/10.1039/c8cs00738a
- Nayak L, Mohanty S, Nayak SK, et al., 2019, A review on inkjet printing of nanoparticle inks for flexible electronics. J Mater Chem C, 7:8771–8795. https://doi.org/10.1039/C9TC01630A
- Fernandes IJ, Aroche AF, Schuck A, et al., 2020, Silver nanoparticle conductive inks: Synthesis, characterization, and fabrication of inkjet-printed flexible electrodes. Sci Rep, 10:8878. https://doi.org/10.1038/s41598-020-65698-3
- Rahman MK, Lee JS, Kwon KS, 2023, Fine conductive line printing of high viscosity CuO ink using near field electrospinning (NFES). Sci Rep, 13:17668. https://doi.org/10.1038/s41598-023-45083-6
- Han E, Pan Y, Li L, et al., 2023, Bisphenol a detection based on nano gold-doped molecular imprinting electrochemical sensor with enhanced sensitivity. Food Chem, 426:136608. https://doi.org/10.1016/j.foodchem.2023.136608
- Sadeghi M, Shabani-Nooshabadi M, 2022, Use of a nano-porous gold film electrode modified with chitosan/polypyrrole for electrochemical determination of metronidazole in the presence of acetaminophen. Chemosphere, 307:135722. https://doi.org/10.1016/j.chemosphere.2022.135722
- Tuleushova N, Amanova A, Abdellah I, et al., 2023, Radiolysis-assisted direct growth of gold-based electrocatalysts for glycerol oxidation. Nanomaterials (Basel), 13:1713. https://doi.org/10.3390/nano13111713
- Ma B, Xu C, Chi J, et al., 2019, A versatile approach for direct patterning of liquid metal using magnetic field. Adv Funct Mater, 29:1901370. https://doi.org/10.1002/adfm.201901370
- Neumann TV, Dickey MD, 2020, Liquid metal direct write and 3D printing: A review. Adv Mater Technol, 5:2000070. https://doi.org/10.1002/admt.202000070
- Saborio MG, Cai S, Tang J, et al., 2020, Liquid metal droplet and graphene co-fillers for electrically conductive flexible composites. Small, 16: e1903753. https://doi.org/10.1002/smll.201903753
- Dickey MD, 2017, Stretchable and soft electronics using liquid metals. Adv Mater, 29:1606425. https://doi.org/10.1002/adma.201606425
- Wu Q, Zhu F, Wu Z, et al., 2022, Suspension printing of liquid metal in yield-stress fluid for resilient 3D constructs with electromagnetic functions. Npj Flex Electron, 6:50. https://doi.org/10.1038/s41528-022-00184-6
- Lee W, Kim H, Kang I, et al., 2022, Universal assembly of liquid metal particles in polymers enables elastic printed circuit board. Science, 378:637–641. https://doi.org/10.1126/science.abo6631
- An L, Jiang H, de Camargo Branco D, et al., 2022, Self-packaged high-resolution liquid metal nano-patterns. Matter, 5:1016–1030. https://doi.org/10.1016/j.matt.2022.01.004
- Parker CJ, Krishnamurthi V, Zuraiqi K, et al., 2023, Synthesis of planet-like liquid metal nanodroplets with promising properties for catalysis. Adv Funct Mater, 2304248. https://doi.org/10.1002/adfm.202304248
- Sheng A, Khuje S, Yu J, et al., 2022, Copper nanoplates for printing flexible high-temperature conductors. ACS Appl Nano Mater, 5:4028–4037. https://doi.org/10.1021/acsanm.2c00019
- Wang F, Gosling JH, Trindade GF, et al., 2021, Inter-flake quantum transport of electrons and holes in inkjet-printed graphene devices. Adv Funct Mater, 31:2007478. https://doi.org/10.1002/adfm.202007478
- E Silva EP, Huang B, Helaehil JV, et al., 2021, In vivo study of conductive 3D printed PCL/MWCNTs scaffolds with electrical stimulation for bone tissue engineering. Biodes Manuf, 4:190–202. https://doi.org/10.1007/s42242-020-00116-1
- Kanoun O, Bouhamed A, Ramalingame R, et al., 2021, Review on conductive polymer/CNTs nanocomposites based flexible and stretchable strain and pressure sensors. Sensors, 21:341. https://doi.org/10.3390/s21020341
- Shi Z, Meng L, Shi X, et al., 2022, Morphological engineering of sensing materials for flexible pressure sensors and artificial intelligence applications. Nanomicro Lett, 14:141. https://doi.org/10.1007/s40820-022-00874-w
- Sun X, Wang C, Chi C, et al., 2018, A highly-sensitive flexible tactile sensor array utilizing piezoresistive carbon nanotube-polydimethylsiloxane composite. J Micromech Microeng, 28:105011. https://doi.org/10.1088/1361-6439/aaceb9
- Zhao T, Li T, Chen L, et al., 2019, Highly sensitive flexible piezoresistive pressure sensor developed using biomimetically textured porous materials. ACS Appl Mater Interfaces, 11:29466–29473. https://doi.org/10.1021/acsami.9b09265
- Park SJ, Kim J, Chu M, et al., 2018, Flexible piezoresistive pressure sensor using wrinkled carbon nanotube thin films for human physiological signals. Adv Mater Technol, 3:1700158. https://doi.org/10.1002/admt.201700158
- Jia J, Huang G, Deng J, et al., 2019, Skin-inspired flexible and high-sensitivity pressure sensors based on rGO films with continuous-gradient wrinkles. Nanoscale, 11:4258–4266. https://doi.org/10.1039/C8NR08503J
- Yu R, Xia T, Wu B, et al., 2020, Highly sensitive flexible piezoresistive sensor with 3D conductive network. ACS Appl Mater Interfaces, 12:35291–35299. https://doi.org/10.1021/acsami.0c09552
- Jeong WY, Choi HE, Kim KS. Based Nanomaterials as Drug Delivery Carriers. SpringerLink. Available from: https:// link.springer.com/chapter/10.1007/978-981-16-4923- 3_6#sec7”graphene [Last accessed on 2023 Sep 11]. https://doi.org/10.1007/978-981-16-4923-3_6
- Manikandan V, Lee NY, 2023, Reduced graphene oxide: Biofabrication and environmental applications. Chemosphere, 311:136934. https://doi.org/10.1016/j.chemosphere.2022.136934
- Chen Y, Li L, Zhang J, et al., 2023, Tailored ionically conductive graphene oxide-encased metal ions for ultrasensitive cadaverine sensor. Chin Chem Lett, 109102. https://doi.org/10.1016/j.cclet.2023.109102
- Yang J, Zhang K, Yu J, et al., 2021, Facile fabrication of robust and reusable PDMS supported graphene dry electrodes for wearable electrocardiogram monitoring. Adv Mater Technol, 6:2100262. https://doi.org/10.1002/admt.202100262
- Qian C, Xiao T, Chen Y, et al., 2022, 3D printed reduced graphene oxide/elastomer resin composite with structural modulated sensitivity for flexible strain sensor. Adv Eng Mater, 24:2101068. https://doi.org/10.1002/adem.202101068
- Fang Y, Wang C, Liu Z, et al., 2023, 3D printed conductive multiscale nerve guidance conduit with hierarchical fibers for peripheral nerve regeneration. Adv Sci (Weinh), 10: e2205744. https://doi.org/10.1002/advs.202205744
- Su T, Wang Y, Zhu Q, et al., 2023, Multiple conductive network for KTi2(PO4)3 anode based on MXene as a binder for high-performance potassium storage. Chin Chem Lett, 109191. https://doi.org/10.1016/j.cclet.2023.109191
- Wang F, Yang C, Duan C, et al., 2014, An organ-like titanium carbide material (MXene) with multilayer structure encapsulating hemoglobin for a mediator-free biosensor. J Electrochem Soc, 162: B16. https://doi.org/10.1149/2.0371501jes
- Amara U, Rashid S, Mahmood K, et al., 2022, Insight into prognostics, diagnostics, and management strategies for SARS CoV-2. RSC Adv, 12:8059–8094. https://doi.org/10.1039/D1RA07988C
- Dos Santos CC, Lucena GN, Pinto GC, et al., 2021, Advances and current challenges in non-invasive wearable sensors and wearable biosensors-a mini-review. Med Devices Sens, 4: e10130. https://doi.org/10.1002/mds3.10130
- Wang G, Park JM, Kang T, et al., 2023, Anion storage of MXenes. Small Methods, 7: e2201440. https://doi.org/10.1002/smtd.202201440
- Elbadawi M, Ong JJ, Pollard TD, et al., 2021, Additive manufacturable materials for electrochemical biosensor electrodes. Adv Funct Mater, 31:2006407. https://doi.org/10.1002/adfm.202006407
- Chen L, Eriksson A, Weström S, et al., 2022, Ultra-sensitive monitoring of leukemia patients using superRCA mutation detection assays. Nat Commun, 13:4033. https://doi.org/10.1038/s41467-022-31397-y
- Cui T, Qiao Y, Li D, et al., 2023, Multifunctional, breathable MXene-PU mesh electronic skin for wearable intelligent 12-lead ECG monitoring system. Chem Eng J, 455:140690. https://doi.org/10.1016/j.cej.2022.140690
- Li L, Meng J, Bao X, et al., 2023, Direct-ink-write 3D printing of programmable micro-supercapacitors from MXene-regulating conducting polymer inks. Adv Energy Mater, 13:2203683. https://doi.org/10.1002/aenm.202203683
- Bubniene US, Ratautaite V, Ramanavicius A, et al., 2022, Conducting polymers for the design of tactile sensors. Polymers (Basel), 14:2984. https://doi.org/10.3390/polym14152984
- Gaikar PS, Kadu KS, Tehare KK, et al., 2022, Recent developments in polypyrrole/manganese oxide-based nanocomposites for thin film electrodes in supercapacitors: A minireview. Nanoscale Adv, 4:5245–5252. https://doi.org/10.1039/d2na00654e
- Chu X, Chen G, Xiao X, et al., 2021, Air-stable conductive polymer ink for printed wearable micro-supercapacitors. Small, 17: e2100956. https://doi.org/10.1002/smll.202100956
- Gonzalez G, Nelson AC, Holman AR, et al., 2023, Conductive electrospun polymer improves stem cell-derived cardiomyocyte function and maturation. Biomaterials, 302:122363. https://doi.org/10.1016/j.biomaterials.2023.122363
- Lim T, Kim M, Akbarian A, et al., 2022, Conductive polymer enabled biostable liquid metal electrodes for bioelectronic applications. Adv Healthc Mater, 11: e2102382. https://doi.org/10.1002/adhm.202102382
- Tseng CP, Liu F, Zhang X, et al., 2022, Solution-deposited and patternable conductive polymer thin-film electrodes for microbial bioelectronics. Adv Mater, 34: e2109442. https://doi.org/10.1002/adma.202109442
- Kayser LV, Lipomi DJ, 2019, Stretchable conductive polymers and composites based on PEDOT and PEDOT:PSS. Adv Mater, 31: e1806133. https://doi.org/10.1002/adma.201806133
- O’Neill SJ, Huang Z, Ahmed MH, et al., 2023, Tissue-mimetic supramolecular polymer networks for bioelectronics. Adv Mater, 35: e2207634. https://doi.org/10.1002/adma.202207634
- Yang G, Zhang YM, Cai Y, et al., 2020, Advances in nanomaterials for electrochromic devices. Chem Soc Rev, 49:8687–8720. https://doi.org/10.1039/D0CS00317D
- Li XG, Wang HY, Huang MR, 2007, Synthesis, film-forming, and electronic properties of o-phenylenediamine copolymers displaying an uncommon tricolor. Macromolecules, 40:1489–1496. https://doi.org/10.1021/ma062463g
- Huang X, Chen J, Xie H, et al., 2022, Inkjet printing of 2D polyaniline for fabricating flexible and patterned electrochromic devices. Sci China Mater, 65:2217–2226. https://doi.org/10.1007/s40843-022-2037-4
- Shirakawa H, 2001, The discovery of polyacetylene film: The dawning of an era of conducting polymers (nobel lecture). Angew Chem Int Ed Engl, 40:2574–2580. https://doi.org/10.1002/1521-3773(20010716)40::14<2574::AID-ANIE2574>3.0.CO;2-N
- Luppi BT, Muralidharan AV, Ostermann N, et al., 2022, Redox-active heteroatom-functionalized polyacetylenes. Angew Chem Int Ed Engl, 61: e202114586. https://doi.org/10.1002/anie.202114586
- Miao Z, Gonsales SD, Ehm C, et al., 2021, Cyclic polyacetylene. Nat Chem, 13:792–799. https://doi.org/10.1038/s41557-021-00713-2
- Yang J, Cui N, Han D, et al., 2022, A simple strategy for constructing hierarchical composite electrodes of PPy-posttreated 3D-printed carbon aerogel with ultrahigh areal capacitance over 8000 mF cm-2. Adv Mater Technol, 7:2101325. https://doi.org/10.1002/admt.202101325
- Li Q, Tang R, Zhou H, et al., 2023, A high-performance and flexible electrode film based on bacterial cellulose/polypyrrole/nitrogen-doped graphene for supercapacitors. Carbohydr Polym, 311:120754. https://doi.org/10.1016/j.carbpol.2023.120754
- Shi X, Sun L, Li X, et al., 2022, High-performance flexible supercapacitor enabled by polypyrrole-coated NiCoP@ CNT electrode for wearable devices. J Colloid Interface Sci, 606:135–147. https://doi.org/10.1016/j.jcis.2021.08.016
- Zotti G, Vercelli B, Berlin A, 2008, Monolayers and multilayers of conjugated polymers as nanosized electronic components. Acc Chem Res, 41:1098–1109. https://doi.org/10.1021/ar8000102
- Ren SB, Ma W, Zhang C, et al., 2020, Exploiting polythiophenyl-triazine-based conjugated microporous polymer with superior lithium-storage performance. ChemSusChem, 13:2295–2302. https://doi.org/10.1002/cssc.202000200
- Memon MA, Bai W, Sun J, et al., 2016, Conjunction of conducting polymer nanostructures with macroporous structured graphene thin films for high-performance flexible supercapacitors. ACS Appl Mater Interfaces, 8:11711–11719. https://doi.org/10.1021/acsami.6b01879
- Liu Y, Li J, Song S, et al., 2020, Morphing electronics enable neuromodulation in growing tissue. Nat Biotechnol, 38:1031–1036. https://doi.org/10.1038/s41587-020-0495-2
- Fan X, Nie W, Tsai H, et al., 2019, PEDOT:PSS for flexible and stretchable electronics: Modifications, strategies, and applications. Adv Sci (Weinh), 6:1900813. https://doi.org/10.1002/advs.201900813
- Lipomi DJ, Vosgueritchian M, Tee BC, et al., 2011, Skin-like pressure and strain sensors based on transparent elastic films of carbon nanotubes. Nat Nanotechnol, 6:788–792. https://doi.org/10.1038/nnano.2011.184
- Nurazizah ES, Aprilia A, Risdiana R, et al., 2023, Different roles between PEDOT:PSS as counter electrode and PEDOT:Carrageenan as electrolyte in dye-sensitized solar cell applications: A systematic literature review. Polymers (Basel), 15:2725. https://doi.org/10.3390/polym15122725
- Sun Z, He Y, Xiong B, et al., 2021, Performance-enhancing approaches for PEDOT:PSS-Si hybrid solar cells. Angew Chem Int Ed Engl, 60:5036–5055. https://doi.org/10.1002/anie.201910629
- Yuk H, Lu B, Lin S, et al., 2020, 3D printing of conducting polymers. Nat Commun, 11:1604. https://doi.org/10.1038/s41467-020-15316-7
- Tomaskovic-Crook E, Zhang P, Ahtiainen A, et al., 2019, Human neural tissues from neural stem cells using conductive biogel and printed polymer microelectrode arrays for 3D electrical stimulation. Adv Healthc Mater, 8: e1900425. https://doi.org/10.1002/adhm.201900425
- Kappen J, Skorupa M, Krukiewicz K, 2022, Conducting polymers as versatile tools for the electrochemical detection of cancer biomarkers. Biosensors (Basel), 13:31. https://doi.org/10.3390/bios13010031
- Yuk H, Lu B, Zhao X, 2019, Hydrogel bioelectronics. Chem Soc Rev, 48:1642–1667. https://doi.org/10.1039/C8CS00595H
- Xie X, Xu Z, Yu X, et al., 2023, Liquid-in-liquid printing of 3D and mechanically tunable conductive hydrogels. Nat Commun, 14:4289. https://doi.org/10.1038/s41467-023-40004-7
- Zhou T, Yuk H, Hu F, et al., 2023, 3D printable high-performance conducting polymer hydrogel for all-hydrogel bioelectronic interfaces. Nat Mater, 22:895–902. https://doi.org/10.1038/s41563-023-01569-2
- Wong J, Gong AT, Defnet PA, et al., 2019, 3D Printing ionogel auxetic frameworks for stretchable sensors. Adv Mater Technol, 4:1900452. https://doi.org/10.1002/admt.201900452
- Zhou LY, Fu J, He Y, 2020, A review of 3D printing technologies for soft polymer materials. Adv Funct Mater, 30:2000187. https://doi.org/10.1002/adfm.202000187
- Cheng J, Wang R, Sun Z, et al., 2022, Centrifugal multimaterial 3D printing of multifunctional heterogeneous objects. Nat Commun, 13:7931. https://doi.org/10.1038/s41467-022-35622-6
- Ma X, Yu J, Hu Y, et al., 2023, Ionic liquid/poly(ionic liquid)-based electrolytes for lithium batteries. Ind Chem Mater, 1:39–59. https://doi.org/10.1039/D2IM00051B
- Fan X, Liu S, Jia Z, et al., 2023, Ionogels: Recent advances in design, material properties and emerging biomedical applications. Chem Soc Rev, 52:2497–2527. https://doi.org/10.1039/D2CS00652A
- Luo Z, Li W, Yan J, et al., 2022, Roles of ionic liquids in adjusting nature of ionogels: A mini review. Adv Funct Mater, 32:2203988. https://doi.org/10.1002/adfm.202203988
- Huang J, Yu Z, Wu P, 2023, 3D printing of ionogels with complementary functionalities enabled by self-regulating ink. Adv Sci, 10:2302891. https://doi.org/10.1002/advs.202302891
- Wang M, Lai Z, Jin X, et al., 2021, Multifunctional liquid-free ionic conductive elastomer fabricated by liquid metal induced polymerization. Adv Funct Mater, 31:2101957. https://doi.org/10.1002/adfm.202101957
- Yiming B, Han Y, Han Z, et al., 2021, A mechanically robust and versatile liquid-free ionic conductive elastomer. Adv Mater, 33: e2006111. https://doi.org/10.1002/adma.202006111
- Cao Y, Tan YJ, Li S, et al., 2019, Self-healing electronic skins for aquatic environments. Nat Electron, 2:75–82. https://doi.org/10.1038/s41928-019-0206-5
- Wang M, Zhang P, Shamsi M, et al., 2022, Tough and stretchable ionogels by in situ phase separation. Nat Mater, 21:359–365. https://doi.org/10.1038/s41563-022-01195-4
- Yu Z, Wu P, 2021, Underwater communication and optical camouflage ionogels. Adv Mater, 33: e2008479. https://doi.org/10.1002/adma.202008479
- Yao Y, Hui Y, Wang Z, et al., 2023, Granular ionogel particle inks for 3D printed tough and stretchable ionotronics. Research (Wash D C), 6:0104. https://doi.org/10.34133/research.0104
- Zhang M, Yu R, Tao X, et al., 2023, Mechanically robust and highly conductive ionogels for soft ionotronics. Adv Funct Mater, 33:2208083. https://doi.org/10.1002/adfm.202208083
- Xiong X, Chen Y, Wang Z, et al., 2023, Polymerizable rotaxane hydrogels for three-dimensional printing fabrication of wearable sensors. Nat Commun, 14:1331. https://doi.org/10.1038/s41467-023-36920-3
- Xiao J, Gao Y, 2017, The manufacture of 3D printing of medical grade TPU. Prog Addit Manuf, 2:117–123. https://doi.org/10.1007/s40964-017-0023-1
- Hu X, Chen Y, Xu W, et al., 2023, 3D-printed thermoplastic polyurethane electrodes for customizable, flexible lithium-ion batteries with an ultra-long lifetime. Small, 19: e2301604. https://doi.org/10.1002/smll.202301604