From tumor-resistant cardiac fatty acid oxidation to whole-body metabolic reprogramming: Anti-Warburg effect of the high-fat AMRD diet for cancer therapy
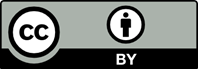
Cancer cells primarily rely on anaerobic glycolysis for energy, a phenomenon known as the Warburg effect, which drives rapid growth, immunosuppression, and tumor microenvironment acidification. In stark contrast, the heart, a tissue remarkably resistant to cancer relies predominantly on fatty acid oxidation (FAO) for its energy demands. This distinctive metabolic resilience inspired the development of a comprehensive therapeutic approach: the Akl metabolic reprogramming diet (AMRD). Unlike conventional strategies that target genetic mutations, AMRD exploits metabolic vulnerabilities, aiming to reprogram cancer cell metabolism from glycolysis to FAO. Central to this approach is the “Akl effect,” a concept proposing that excessive glycolipid accumulation impairs glucose transporter function, forcing cancer cells into a compensatory glycolytic state. By redirecting metabolic pathways toward FAO, AMRD induces metabolic stress, disrupts biosynthetic pathways, and selectively triggers apoptosis in cancer cells. Unlike typical ketogenic diets, AMRD incorporates tailored lipid profiles, moderate protein intake, and controlled carbohydrate levels to maximize metabolic flexibility in healthy tissues while restricting cancer cell metabolism. This dietary strategy has demonstrated potential against glycolysis-dependent cancers like breast cancer, glioblastoma, and non-small cell lung cancer. By increasing reactive oxygen species (ROS) production through enhanced FAO, AMRD imposes oxidative stress that overwhelms cancer cells’ impaired antioxidant defenses, while healthy cells with intact redox homeostasis effectively neutralize ROS. As a precision-based, low-toxicity approach, AMRD represents a paradigm shift in cancer therapy, leveraging the heart’s metabolic resistance to develop a restorative, whole-body strategy against aggressive tumors. This innovative approach has the potential to redefine metabolic cancer therapy.
- Rui L. Energy metabolism in the liver. Compr Physiol. 2014;4(1):177-197. doi: 10.1002/cphy.c130024
- Nguyen BY, Ruiz-Velasco A, Bui T, Collins L, Wang X, Liu W. Mitochondrial function in the heart: the insight into mechanisms and therapeutic potentials. Br J Pharmacol. 2019;176(22):4302-4318. doi: 10.1111/bph.14431
- Lopaschuk GD, Ussher JR, Folmes CD, Jaswal JS, Stanley WC. Myocardial fatty acid metabolism in health and disease. Physiol Rev. 2010;90(1):207-258. doi: 10.1152/physrev.00015.2009
- Liang K. Mitochondrial CPT1A: Insights into structure, function, and basis for drug development. Front Pharmacol. 2023;14:1160440. doi: 10.3389/fphar.2023.1160440
- Chen S, Zou Y, Song C, et al. The role of glycolytic metabolic pathways in cardiovascular disease and potential therapeutic approaches. Basic Res Cardiol. 2023;118:48. doi: 10.1007/s00395-023-01018-w
- Karlstaedt A, Taegtmeyer H. Cardio-onco-metabolism - metabolic vulnerabilities in cancer and the heart. J Mol Cell Cardiol. 2022;171:71-80. doi: 10.1016/j.yjmcc.2022.06.008
- Liberti MV, Locasale JW. The Warburg effect: How does it benefit cancer cells? Trends Biochem Sci. 2016;41(3):211-218. doi: 10.1016/j.tibs.2015.12.001
- Luo W, Hu H, Chang R, et al. Pyruvate kinase M2 is a PHD3- stimulated coactivator for hypoxia-inducible factor 1. Cell. 2011;145(5):732-744. doi: 10.1016/j.cell.2011.03.054
- Phan LM, Yeung SC, Lee MH. Cancer metabolic reprogramming: Importance, main features, and potentials for precise targeted anti-cancer therapies. Cancer Biol Med. 2014;11(1):1-19. doi: 10.7497/j.issn.2095-3941.2014.01.001
- Moris D, Spartalis M, Spartalis E, et al. The role of reactive oxygen species in the pathophysiology of cardiovascular diseases and the clinical significance of myocardial redox. Ann Transl Med. 2017;5(16):326. doi: 10.21037/atm.2017.06.27
- Navarro C, Ortega Á, Santeliz R, et al. Metabolic reprogramming in cancer cells: Emerging molecular mechanisms and novel therapeutic approaches. Pharmaceutics. 2022;14(6):1303. doi: 10.3390/pharmaceutics14061303
- Nsiah-Sefaa A, McKenzie M. Combined defects in oxidative phosphorylation and fatty acid β-oxidation in mitochondrial disease. Biosci Rep. 2016;36(2):e00313. doi: 10.1042/BSR20150295
- Akl MM, Ahmed A. The Role of pH in cancer biology and its impact on cellular repair, tumor markers, tumor stages, isoenzymes, and therapeutics. Med Commun Health Arch. 2023;1(3):78-87. doi: 10.23958/mcha/vol01/i03/32
- Patra KC, Hay N. The pentose phosphate pathway and cancer. Trends Biochem Sci. 2014;39(8):347-354. doi: 10.1016/j.tibs.2014.06.005
- Chelakkot C, Chelakkot VS, Shin Y, Song K. Modulating glycolysis to improve cancer therapy. Int J Mol Sci. 2023;24(3):2606. doi: 10.3390/ijms24032606
- Goodpaster BH, Sparks LM. Metabolic flexibility in health and disease. Cell Metab. 2017;25(5):1027-1036. doi: 10.1016/j.cmet.2017.04.015
- Carracedo A, Cantley LC, Pandolfi PP. Cancer metabolism: Fatty acid oxidation in the limelight. Nat Rev Cancer. 2013;13(4):227-232. doi: 10.1038/nrc3483
- Wang Y, Liu Z, Han Y, Xu J, Huang W, Li Z. Medium Chain Triglycerides enhances exercise endurance through the increased mitochondrial biogenesis and metabolism. PLoS One. 2018;13(2):e0191182. doi: 10.1371/journal.pone.0191182
- Aufricht C, Neuhofer W, Topley N, Wörnle M. Peritoneal infection and inflammation. Mediat Inflamm. 2012;2012:456985. doi: 10.1155/2012/456985
- Zorov DB, Juhaszova M, Sollott SJ. Mitochondrial reactive oxygen species (ROS) and ROS-induced ROS release. Physiol Rev. 2014;94(3):909-950. doi: 10.1152/physrev.00026.2013
- Zivkovic AM, Telis N, German JB, Hammock BD. Dietary omega-3 fatty acids aid in the modulation of inflammation and metabolic health. Calif Agric. 2011;65(3):106-111. doi: 10.3733/ca.v065n03p106
- Watanabe S, Tsujino S. Applications of medium-chain triglycerides in foods. Front Nutr. 2022;9:802805. doi: 10.3389/fnut.2022.802805
- Wang ZH, Peng WB, Zhang P, Yang XP, Zhou Q. Lactate in the tumour microenvironment: From immune modulation to therapy. EBioMedicine, 2021;73:103627. doi: 10.1016/j.ebiom.2021.103627
- Ward C, Meehan J, Gray ME, et al. The impact of tumour pH on cancer progression: Strategies for clinical intervention. Explor Target Antitumor Ther. 2020;1(2):71-100. doi: 10.37349/etat.2020.00005
- Wang Z, Jiang Q, Dong C. Metabolic reprogramming in triple-negative breast cancer. Cancer Biol Med. 2020;17(1):44-59. doi: 10.20892/j.issn.2095-3941.2019.0210
- Stine ZE, Schug ZT, Salvino JM, et al. Targeting cancer metabolism in the era of precision oncology. Nat Rev Drug Discov. 2022;21:141-162. doi: 10.1038/s41573-021-00339-6
- Dal Bello S, Valdemarin F, Martinuzzi D, Filippi F, Gigli GL, Valente M. Ketogenic diet in the treatment of gliomas and glioblastomas. Nutrients. 2022;14(18):3851. doi: 10.3390/nu14183851
- Yang J, Shay C, Saba NF, Teng Y. Cancer metabolism and carcinogenesis. Exp Hematol Oncol. 2024;13(1):10. doi: 10.1186/s40164-024-00482-x
- Kolwicz SC Jr., Purohit S, Tian R. Cardiac metabolism and its interactions with contraction, growth, and survival of cardiomyocytes. Circul Res. 2013;113(5):603-616. doi: 10.1161/CIRCRESAHA.113.302095
- Phan LM, Yeung SC, Lee MH. Cancer metabolic reprogramming: Importance, main features, and potentials for precise targeted anti-cancer therapies. Cancer Biol Med. 2014;11(1):1-19. doi: 10.7497/j.issn.2095-3941.2014.01.001
- Dong L, He J, Luo L, Wang K. Targeting the interplay of autophagy and ROS for cancer therapy: An updated overview on phytochemicals. Pharmaceuticals (Basel, Switzerland). 2023;16(1):92. doi: 10.3390/ph16010092
- Jomova K, Alomar SY, Alwasel SH, Nepovimova E, Kuca K, Valko M. Several lines of antioxidant defense against oxidative stress: Antioxidant enzymes, nanomaterials with multiple enzyme-mimicking activities, and low-molecular-weight antioxidants. Arch Toxicol. 2024;98(5):1323-1367. doi: 10.1007/s00204-024-03696-4
- Akl MM, Ahmed A. Exploring the Interplay between the Warburg effect and glucolipotoxicity in cancer development: A novel perspective on cancer etiology. Adv Pharm Bull. 2024;14(3):705-713. doi: 10.34172/apb.2024.049
- Mafi S, Mansoori B, Taeb S, et al. mTOR-Mediated regulation of immune responses in cancer and tumor microenvironment. Front Immunol. 2022;12:774103. doi: 10.3389/fimmu.2021.774103
- Chen Q, Kovilakath A, Allegood J, et al. Endoplasmic reticulum stress and mitochondrial dysfunction during aging: Role of sphingolipids. Biochim Biophys Acta Mol Cell Biol Lipids. 2023;1868(10):159366. doi: 10.1016/j.bbalip.2023.159366
- Glaviano A, Foo AS, Lam HY, et al. PI3K/AKT/mTOR signaling transduction pathway and targeted therapies in cancer. Mol Cancer. 2023;22(1):138. doi: 10.1186/s12943-023-01827-6
- Payen VL, Mina E, Van Hée VF, Porporato PE, Sonveaux P. Monocarboxylate transporters in cancer. Mol Metab. 2020;33:48-66. doi: 10.1016/j.molmet.2019.07.006
- Puchalska P, Crawford PA. Metabolic and signaling roles of ketone bodies in health and disease. Ann Rev Nutr. 2021;41:49-77. doi: 10.1146/annurev-nutr-111120-111518
- Mohammadifard N, Haghighatdoost F, Rahimlou M, et al. The effect of ketogenic diet on shared risk factors of cardiovascular disease and cancer. Nutrients. 2022;14(17):3499. doi: 10.3390/nu14173499
- Smith RL, Soeters MR, Wüst RC, Houtkooper RH. Metabolic flexibility as an adaptation to energy resources and requirements in health and disease. Endocr Rev. 2018;39(4):489-517. doi: 10.1210/er.2017-00211
- Batch JT, Lamsal SP, Adkins M, Sultan S, Ramirez MN. Advantages and disadvantages of the ketogenic diet: A review article. Cureus. 2020;12(8):e9639. doi: 10.7759/cureus.9639