Functional materials of 3D bioprinting for wound dressings and skin tissue engineering applications: A review
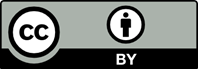
The skin plays an important role in vitamin D synthesis, humoral balance, temperature regulation, and waste excretion. Due to the complexity of the skin, fluids loss, bacterial infection, and other life-threatening secondary complications caused by skin defects often lead to the damage of skin functions. 3D bioprinting technology, as a customized and precise biomanufacturing platform, can manufacture dressings and tissue engineering scaffolds that accurately simulate tissue structure, which is more conducive to wound healing. In recent years, with the development of emerging technologies, an increasing number of 3D-bioprinted wound dressings and skin tissue engineering scaffolds with multiple functions, such as antibacterial, antiinflammatory, antioxidant, hemostatic, and antitumor properties, have significantly improved wound healing and skin treatment. In this article, we review the process of wound healing and summarize the classification of 3D bioprinting technology. Following this, we shift our focus on the functional materials for wound dressing and skin tissue engineering, and also highlight the research progress and development direction of 3D-bioprinted multifunctional wound healing materials.
Moeini A, Pedram P, Makvandi P, et al., 2020, Wound healing and antimicrobial effect of active secondary metabolites in chitosan-based wound dressings: A review. Carbohydr Polym, 233:115839. https://doi.org/10.1016/j.carbpol.2020.115839
Kus KJB, Ruiz ES, 2020, Wound dressings—A practical review. Curr Derm Rep, 9(4):298–308. https://doi.org/10.1007/s13671-020-00319-w
Li R, Liu K, Huang X, et al., 2022, Bioactive materials promote wound healing through modulation of cell behaviors. Adv Sci (Weinh), 9(10):e2105152. https://doi.org/10.1002/advs.202105152
Simoes D, Miguel SP, Ribeiro MP, et al., 2018, Recent advances on antimicrobial wound dressing: A review. Eur J Pharm Biopharm, 127:130–141. https://doi.org/10.1016/j.ejpb.2018.02.022
Zhang K, Wang Y, Wei Q, et al., 2021, Design and fabrication of sodium alginate/carboxymethyl cellulose sodium blend hydrogel for artificial skin. Gels, 7(3):115. https://doi.org/10.3390/gels7030115
Farahani M, Shafiee A, 2021, Wound healing: From passive to smart dressings. Adv Healthc Mater, 10(16):e2100477. https://doi.org/10.1002/adhm.202100477
Wang F, Wang S, Nan L, et al., 2022, Conductive adhesive and antibacterial zwitterionic hydrogel dressing for therapy of full-thickness skin wounds. Front Bioeng Biotechnol, 10:833887. https://doi.org/10.3389/fbioe.2022.833887
Katiyar S, Singh D, Kumari S, et al., 2022, Novel strategies for designing regenerative skin products for accelerated wound healing. 3 Biotech, 12(11):316. https://doi.org/10.1007/s13205-022-03331-y
Da LC, Huang YZ, Xie HQ, 2017, Progress in development of bioderived materials for dermal wound healing. Regen Biomater, 4(5):325–334. https://doi.org/10.1093/rb/rbx025
Xu J, Fang H, Zheng S, et al., 2021, A biological functional hybrid scaffold based on decellularized extracellular matrix/gelatin/chitosan with high biocompatibility and antibacterial activity for skin tissue engineering. Int J Biol Macromol, 187:840–849. https://doi.org/10.1016/j.ijbiomac.2021.07.162
Wang K, Wang J, Li L, et al., 2020, Novel nonreleasing antibacterial hydrogel dressing by a one-pot method. ACS Biomater Sci Eng, 6(2):1259–1268. https://doi.org/10.1021/acsbiomaterials.9b01812
Guo Y, Huang J, Fang Y, et al., 2022, 1D, 2D, and 3D scaffolds promoting angiogenesis for enhanced wound healing. Chem Eng J, 437:134690. https://doi.org/10.1016/j.cej.2022.134690
Brock WD, Bearden W, Tann T, et al., 2003, Autogenous dermis skin grafts in lower eyelid reconstruction. Ophthalmic Plast Reconstr Surg, 19(5):394–397. https://doi.org/10.1097/01.IOP.0000087070.83353.99
Ali JM, Catarino P, Dunning J, et al., 2016, Could sentinel skin transplants have some utility in solid organ transplantation? Transplant Proc, 48(8):2565–2570. https://doi.org/10.1016/j.transproceed.2016.06.040
Shi C, Zhu Y, Su Y, et al., 2006, Stem cells and their applications in skin-cell therapy. Trends Biotechnol, 24(1):48–52. https://doi.org/10.1016/j.tibtech.2005.11.003
Pajardi G, Rapisarda V, Somalvico F, et al., 2016, Skin substitutes based on allogenic fibroblasts or keratinocytes for chronic wounds not responding to conventional therapy: A retrospective observational study. Int Wound J, 13(1):44–52. https://doi.org/10.1111/iwj.12223
Rastin H, Ramezanpour M, Hassan K, et al., 2021, 3D bioprinting of a cell-laden antibacterial polysaccharide hydrogel composite. Carbohydr Polym, 264:117989. https://doi.org/10.1016/j.carbpol.2021.117989
Yi T, Huang S, Liu G, et al., 2018, Bioreactor synergy with 3D scaffolds: New era for stem cells culture. ACS Appl Bio Mater, 1(2):193–209. https://doi.org/10.1021/acsabm.8b00057
Xue J, Wang X, Wang E, et al., 2019, Bioinspired multifunctional biomaterials with hierarchical microstructure for wound dressing. Acta Biomater, 100:270–279. https://doi.org/10.1016/j.actbio.2019.10.012
Radmanesh S, Shabangiz S, Koupaei N, et al., 2022, 3D printed bio polymeric materials as a new perspective for wound dressing and skin tissue engineering applications: A review. J Polym Res, 29(2):50. https://doi.org/10.1007/s10965-022-02899-6
Aderibigbe BA, Buyana B, 2018, Alginate in wound dressings. Pharmaceutics, 10(2):42. https://doi.org/10.3390/pharmaceutics10020042
Yu P, Zhong W, 2021, Hemostatic materials in wound care. Burns Trauma, 9:tkab019. https://doi.org/10.1093/burnst/tkab019
Pelletier DA, Suresh AK, Holton GA, et al., 2010, Effects of engineered cerium oxide nanoparticles on bacterial growth and viability. Appl Environ Microbiol, 76(24):7981–7989. https://doi.org/10.1128/AEM.00650-10
Yang X, Liu W, Li N, et al., 2017, Design and development of polysaccharide hemostatic materials and their hemostatic mechanism. Biomater Sci, 5(12):2357–2368. https://doi.org/10.1039/c7bm00554g
Wan W, Cai F, Huang J, et al., 2019, A skin-inspired 3D bilayer scaffold enhances granulation tissue formation and anti-infection for diabetic wound healing. J Mater Chem B, 7(18):2954–2961. https://doi.org/10.1039/c8tb03341b
Zhang B, Luo Y, Ma L, et al., 2018, 3D bioprinting: An emerging technology full of opportunities and challenges. Bio-Des Manuf, 1(1):2–13. https://doi.org/10.1007/s42242-018-0004-3
Chen X, Han S, Wu W, et al., 2022, Harnessing 4D printing bioscaffolds for advanced orthopedics. Small, 18(36):e2106824. https://doi.org/10.1002/smll.202106824
Hockaday LA, Kang KH, Colangelo NW, et al., 2012, Rapid 3D printing of anatomically accurate and mechanically heterogeneous aortic valve hydrogel scaffolds. Biofabrication, 4(3):035005. https://doi.org/10.1088/1758-5082/4/3/035005
Zhong H, Huang J, Wu J, et al., 2021, Electrospinning nanofibers to 1D, 2D, and 3D scaffolds and their biomedical applications. Nano Res, 15(2):787–804. https://doi.org/10.1007/s12274-021-3593-7
Song W, Yao B, Zhu D, et al., 2022, 3D-bioprinted microenvironments for sweat gland regeneration. Burns Trauma, 10:tkab044. https://doi.org/10.1093/burnst/tkab044
Liu C, Wang Z, Wei X, et al., 2021, 3D printed hydrogel/PCL core/shell fiber scaffolds with NIR-triggered drug release for cancer therapy and wound healing. Acta Biomater, 131: 314–325. https://doi.org/10.1016/j.actbio.2021.07.011
Zhao C, Chen R, Chen Z, et al., 2021, Bioinspired multifunctional cellulose nanofibril-based in situ liquid wound dressing for multiple synergistic therapy of the postoperative infected wound. ACS Appl Mater Interfaces, 13(43):51578–51591. https://doi.org/10.1021/acsami.1c18221
Gul A, Gallus I, Tegginamath A, et al., 2021, Electrospun antibacterial nanomaterials for wound dressings applications. Membranes (Basel), 11(12):908. https://doi.org/10.3390/membranes11120908
Chouhan D, Dey N, Bhardwaj N, et al., 2019, Emerging and innovative approaches for wound healing and skin regeneration: Current status and advances. Biomaterials, 216:119267. https://doi.org/10.1016/j.biomaterials.2019.119267
Lansdown A, 2002, Calcium a potential central regulator in wound healing in the skin. Wound Repair Regen, 10(5): 271–285.https://doi.org/10.1046/j.1524-475X.2002.10502.x
Homaeigohar S, Boccaccini AR, 2020, Antibacterial biohybrid nanofibers for wound dressings. Acta Biomater, 107:25–49. https://doi.org/10.1016/j.actbio.2020.02.022
Liang Y, He J, Guo B, 2021, Functional hydrogels as wound dressing to enhance wound healing. ACS Nano, 15(8):12687– 12722. https://doi.org/10.1021/acsnano.1c04206
Wang F, Gao Y, Li H, et al., 2022, Effect of natural-based biological hydrogels combined with growth factors on skin wound healing. Nanotechnol Rev, 11(1):2493–2512. https://doi.org/10.1515/ntrev-2022-0122
Wang H, Xu Z, Zhao M, et al., 2021, Advances of hydrogel dressings in diabetic wounds. Biomater Sci, 9(5):1530–1546. https://doi.org/10.1039/d0bm01747g
Brown MS, Ashley B, Koh A, 2018, Wearable technology for chronic wound monitoring: Current dressings, advancements, and future prospects. Front Bioeng Biotechnol, 6:47. https://doi.org/10.3389/fbioe.2018.00047
Tang N, Zheng Y, Cui D, et al., 2021, Multifunctional dressing for wound diagnosis and rehabilitation. Adv Healthc Mater, 10(22):e2101292. https://doi.org/10.1002/adhm.202101292
Hao R, Cui Z, Zhang X, et al., 2021, Rational design and preparation of functional hydrogels for skin wound healing. Front Chem, 9:839055. https://doi.org/10.3389/fchem.2021.839055
Sharifi S, Hajipour MJ, Gould L, et al., 2021, Nanomedicine in healing chronic wounds: Opportunities and Challenges. Mol Pharm, 18(2):550–575. https://doi.org/10.1021/acs.molpharmaceut.0c00346
Mao H, Yang L, Zhu H, et al., 2020, Recent advances and challenges in materials for 3D bioprinting. Prog Nat Sci Mater Int, 30(5):618–634. https://doi.org/10.1016/j.pnsc.2020.09.015
Bishop ES, Mostafa S, Pakvasa M, et al., 2017, 3-D bioprinting technologies in tissue engineering and regenerative medicine: Current and future trends. Genes Dis, 4(4):185–195. https://doi.org/10.1016/j.gendis.2017.10.002
Askari M, Afzali Naniz M, Kouhi M, et al., 2021, Recent progress in extrusion 3D bioprinting of hydrogel biomaterials for tissue regeneration: A comprehensive review with focus on advanced fabrication techniques. Biomater Sci, 9(3):535–573. https://doi.org/10.1039/d0bm00973c
Vedakumari WS, Ayaz N, Karthick AS, et al., 2017, Quercetin impregnated chitosan-fibrin composite scaffolds as potential wound dressing materials—Fabrication, characterization and in vivo analysis. Eur J Pharm Sci, 97:106–112. https://doi.org/10.1016/j.ejps.2016.11.012
Li Y, Zhang W, Niu J, et al., 2012, Mechanism of photogenerated reactive oxygen species and correlation with the antibacterial properties of engineered metal-oxide nanoparticles. ACS Nano, 6(6):5164–5173. https://doi.org/10.1021/nn300934k
Yu H, Gong W, Mei J, et al., 2022, The efficacy of a paeoniflorin-sodium alginate-gelatin skin scaffold for the treatment of diabetic wound: An in vivo study in a rat model. Biomed Pharmacother, 151:113165. https://doi.org/10.1016/j.biopha.2022.113165
Seon GM, Lee MH, Koo MA, et al., 2021, A collagen-AS/ epsilonPLL bilayered artificial substitute regulates anti-inflammation and infection for initial inflamed wound healing. Biomater Sci, 9(20):6865–6878. https://doi.org/10.1039/d1bm01071a
Sadidi H, Hooshmand S, Ahmadabadi A, et al., 2020, Cerium oxide nanoparticles (nanoceria): Hopes in soft tissue engineering. Molecules, 25(19):4559. https://doi.org/10.3390/molecules25194559
Liang Y, Chen B, Li M, et al., 2020, injectable antimicrobial conductive hydrogels for wound disinfection and infectious wound healing. Biomacromolecules, 21(5):1841–1852. https://doi.org/10.1021/acs.biomac.9b01732
Tang Q, Plank TN, Zhu T, et al., 2019, Self-assembly of metallo-nucleoside hydrogels for injectable materials that promote wound closure. ACS Appl Mater Interfaces, 11(22):19743–19750. https://doi.org/10.1021/acsami.9b02265
Xu Z, Han S, Gu Z, et al., 2020, advances and impact of antioxidant hydrogel in chronic wound healing. Adv Healthc Mater, 9(5):e1901502. https://doi.org/10.1002/adhm.201901502
Tang P, Han L, Li P, et al., 2019, Mussel-inspired electroactive and antioxidative scaffolds with incorporation of polydopamine-reduced graphene oxide for enhancing skin wound healing. ACS Appl Mater Interfaces, 11(8):7703– 7714. https://doi.org/10.1021/acsami.8b18931
Nguyen TTT, Ghosh C, Hwang SG, et al., 2013, Characteristics of curcumin-loaded poly (lactic acid) nanofibers for wound healing. J Mater Sci, 48(20):7125–7133. https://doi.org/10.1007/s10853-013-7527-y
Liu L, Hu E, Yu K, et al., 2021, Recent advances in materials for hemostatic management. Biomater Sci, 9(22):7343–7378. https://doi.org/10.1039/d1bm01293b
Du X, Wu L, Yan H, et al., 2021, Microchannelled alkylated chitosan sponge to treat noncompressible hemorrhages and facilitate wound healing. Nat Commun, 12(1):4733. https://doi.org/10.1038/s41467-021-24972-2
Futalan CM, Kan C-C, Dalida ML, et al., 2011, Comparative and competitive adsorption of copper, lead, and nickel using chitosan immobilized on bentonite. Carbohydr Polym, 83(2):528–536. https://doi.org/10.1016/j.carbpol.2010.08.013
Biranje SS, Sun J, Cheng L, et al., 2022, Development of cellulose nanofibril/casein-based 3D composite hemostasis scaffold for potential wound-healing application. ACS Appl Mater Interfaces, 14(3):3792–3808. https://doi.org/10.1021/acsami.1c21039
Gao D, Wang Z, Wu Z, et al., 2020, 3D-printing of solvent exchange deposition modeling (SEDM) for a bilayered flexible skin substitute of poly (lactide-co-glycolide) with bioorthogonally engineered EGF. Mater Sci Eng C Mater Biol Appl, 112:110942. https://doi.org/10.1016/j.msec.2020.110942
Lee WH, Ren H, Wu J, et al., 2016, Electrochemically modulated nitric oxide release from flexible silicone rubber patch: Antimicrobial activity for potential wound healing applications. ACS Biomater Sci Eng, 2(9):1432–1435. https://doi.org/10.1021/acsbiomaterials.6b00360
Sajadimajd S, Bahramsoltani R, Iranpanah A, et al., 2020, Advances on natural polyphenols as anticancer agents for skin cancer. Pharmacol Res, 151:104584. https://doi.org/10.1016/j.phrs.2019.104584
Bal-Ozturk A, Ozkahraman B, Ozbas Z, et al., 2021, Advancements and future directions in the antibacterial wound dressings—A review. J Biomed Mater Res B Appl Biomater, 109(5):703–716. https://doi.org/10.1002/jbm.b.34736
Fang H, Wang J, Li L, et al., 2019, A novel high-strength poly(ionic liquid)/PVA hydrogel dressing for antibacterial applications. Chem Eng J, 365:153–164. https://doi.org/10.1016/j.cej.2019.02.030
Zheng L, Li S, Luo J, et al., 2020, Latest advances on bacterial cellulose-based antibacterial materials as wound dressings. Front Bioeng Biotechnol, 8:593768. https://doi.org/10.3389/fbioe.2020.593768
Thill A, Zeyons O, Spalla O, et al., 2006, Cytotoxicity of CeO2 nanoparticles for Escherichia coli. Physico-chemical insight of the cytotoxicity mechanism. Environ Sci Technol, 40(19):6151–6156. https://doi.org/10.1021/es060999b
Guo Z, Zhang Z, Zhang N, et al., 2022, A mg(2+)/ polydopamine composite hydrogel for the acceleration of infected wound healing. Bioact Mater, 15:203–213. https://doi.org/10.1016/j.bioactmat.2021.11.036
Subramanian AK, Prabhakar R, Vikram NR, et al., 2022, In vitro anti-inflammatory activity of silymarin/ hydroxyapatite/chitosan nanocomposites and its cytotoxic effect using brine shrimp lethality assay. J Popul Ther Clin Pharmacol, 28(2):e71–e77. https://doi.org/10.47750/jptcp.2022.874
Kim H, Kim BH, Huh BK, et al., 2017, Surgical suture releasing macrophage-targeted drug-loaded nanoparticles for an enhanced anti-inflammatory effect. Biomater Sci, 5(8):1670–1677. https://doi.org/10.1039/c7bm00345e
He L, Hong G, Zhou L, et al., 2019, Asiaticoside, a component of centella asiatica attenuates RANKL-induced osteoclastogenesis via NFATc1 and NF-kappaB signaling pathways. J Cell Physiol, 234(4):4267–4276. https://doi.org/10.1002/jcp.27195
Talikowska M, Fu X, Lisak G, 2019, Application of conducting polymers to wound care and skin tissue engineering: A review. Biosens Bioelectron, 135:50–63. https://doi.org/10.1016/j.bios.2019.04.001
Guo B, Ma PX, 2018, Conducting polymers for tissue engineering. Biomacromolecules, 19(6):1764–1782. https://doi.org/10.1021/acs.biomac.8b00276
Zhou L, Zheng H, Wang S, et al., 2020, Biodegradable conductive multifunctional branched poly(glycerol-amino acid)-based scaffolds for tumor/infection-impaired skin multimodal therapy. Biomaterials, 262:120300. https://doi.org/10.1016/j.biomaterials.2020.120300
Ou Q, Zhang S, Fu C, et al., 2021, More natural more better: Triple natural anti-oxidant puerarin/ferulic acid/ polydopamine incorporated hydrogel for wound healing. J Nanobiotechnology, 19(1):237. https://doi.org/10.1186/s12951-021-00973-7
Ren Y, Zhang D, He Y, et al., 2021, Injectable and antioxidative HT/QGA hydrogel for potential application in wound healing. Gels, 7(4):204. https://doi.org/10.3390/gels7040204
Zhao X, Wu H, Guo B, et al., 2017, Antibacterial anti-oxidant electroactive injectable hydrogel as self-healing wound dressing with hemostasis and adhesiveness for cutaneous wound healing. Biomaterials, 122:34–47.https://doi.org/10.1016/j.biomaterials.2017.01.011
Dominguez-Robles J, Martin NK, Fong ML, et al., 2019, Antioxidant PLA composites containing lignin for 3D Printing applications: A potential material for healthcare applications. Pharmaceutics, 11(4):165. https://doi.org/10.3390/pharmaceutics11040165
Comino-Sanz IM, Lopez-Franco MD, Castro B, et al., 2021, The role of antioxidants on wound healing: A review of the current evidence. J Clin Med, 10(16):3558. https://doi.org/10.3390/jcm10163558
Viana-Mendieta P, Sanchez ML, Benavides J, 2022, Rational selection of bioactive principles for wound healing applications: Growth factors and antioxidants. Int Wound J, 19(1):100–113. https://doi.org/10.1111/iwj.13602
Huang Y, Zhao X, Zhang Z, et al., 2020, Degradable gelatin-based IPN cryogel hemostat for rapidly stopping deep noncompressible hemorrhage and simultaneously improving wound healing. Chem Mater, 32(15):6595–6610. https://doi.org/10.1021/acs.chemmater.0c02030
Sung YK, Lee DR, Chung DJ, 2021, Advances in the development of hemostatic biomaterials for medical application. Biomater Res, 25(1):37. https://doi.org/10.1186/s40824-021-00239-1
Hu Z, Zhang DY, Lu ST, et al., 2018, Chitosan-based composite materials for prospective hemostatic applications. Mar Drugs, 16(8):273. https://doi.org/10.3390/md16080273
Biranje SS, Sun J, Shi Y, et al., 2021, Polysaccharide-based hemostats: recent developments, challenges, and future perspectives. Cellulose, 28(14):8899–8937. https://doi.org/10.1007/s10570-021-04132-x
Zhong Y, Hu H, Min N, et al., 2021, Application and outlook of topical hemostatic materials: A narrative review. Ann Transl Med, 9(7):577. https://doi.org/10.21037/atm-20-7160
Duarte AP, Coelho JF, Bordado JC, et al., 2012, Surgical adhesives: Systematic review of the main types and development forecast. Prog Polym Sci, 37(8):1031–1050. https://doi.org/10.1016/j.progpolymsci.2011.12.003
Zheng Y, Ma W, Yang Z, et al., 2022, An ultralong hydroxyapatite nanowire aerogel for rapid hemostasis and wound healing. Chem Eng J, 430:132912. https://doi.org/10.1016/j.cej.2021.132912
Zhou LY, Fu J, He Y, 2020, A review of 3D printing technologies for soft polymer materials. Adv Funct Mater, 30(28):2000187. https://doi.org/10.1002/adfm.202000187
Yang Y, Gao W, 2019, Wearable and flexible electronics for continuous molecular monitoring. Chem Soc Rev, 48(6):1465–1491. https://doi.org/10.1039/c7cs00730b
Shintake J, Cacucciolo V, Floreano D, et al., 2018, Soft robotic grippers. Adv Mater, 30(29):1707035. https://doi.org/10.1002/adma.201707035
Zhu C, Ninh C, Bettinger CJ, 2014, Photoreconfigurable polymers for biomedical applications: Chemistry and macromolecular engineering. Biomacromolecules, 15(10):3474–3494. https://doi.org/10.1021/bm500990z
Kim TH, An DB, Oh SH, et al., 2015, Creating stiffness gradient polyvinyl alcohol hydrogel using a simple gradual freezing-thawing method to investigate stem cell differentiation behaviors. Biomaterials, 40:51–60. https://doi.org/10.1016/j.biomaterials.2014.11.017
Guvendiren M, Molde J, Soares RM, et al., 2016, Designing biomaterials for 3D printing. ACS Biomater Sci Eng, 2(10):1679–1693. https://doi.org/10.1021/acsbiomaterials.6b00121
Wang P, Wang C, Liu C, 2021, Antitumor effects of dioscin in A431 cells via adjusting ATM/p53-mediated cell apoptosis, DNA damage and migration. Oncol Lett, 21(1):59. https://doi.org/10.3892/ol.2020.12321
Jain S, Chandra V, Kumar Jain P, et al., 2019, Comprehensive review on current developments of quinoline-based anticancer agents. Arab J Chem, 12(8):4920–4946. https://doi.org/10.1016/j.arabjc.2016.10.009
Bagheri B, Zarrintaj P, Surwase SS, et al., 2019, Self-gelling electroactive hydrogels based on chitosan-aniline oligomers/ agarose for neural tissue engineering with on-demand drug release. Colloids Surf B Biointerfaces, 184:110549. https://doi.org/10.1016/j.colsurfb.2019.110549
Qiu M, Wang D, Liang W, et al., 2018, Novel concept of the smart NIR-light-controlled drug release of black phosphorus nanostructure for cancer therapy. Proc Natl Acad Sci U S A, 115(3):501–506. https://doi.org/10.1073/pnas.1714421115
Ghofrani A, Taghavi L, Khalilivavdareh B, et al., 2022, Additive manufacturing and advanced functionalities of cardiac patches: A review. Eur Polym J, 174:111332. https://doi.org/10.1016/j.eurpolymj.2022.111332
Han S, Wu J, 2022, Three-dimensional (3D) scaffolds as powerful weapons for tumor immunotherapy. Bioact Mater, 17:300–319. https://doi.org/10.1016/j.bioactmat.2022.01.020
Bhar B, Chouhan D, Pai N, et al., 2021, Harnessing multifaceted next-generation technologies for improved skin wound healing. ACS Appl Biol Mater, 4(11):7738–7763. https://doi.org/10.1021/acsabm.1c00880
Lee M, Rizzo R, Surman F, et al., 2020, Guiding lights: Tissue bioprinting using photoactivated materials. Chem Rev, 120(19):10950–11027. https://doi.org/10.1021/acs.chemrev.0c00077
Long J, Etxeberria AE, Nand AV, et al., 2019, A 3D printed chitosan-pectin hydrogel wound dressing for lidocaine hydrochloride delivery. Mater Sci Eng C Mater Biol Appl, 104:109873. https://doi.org/10.1016/j.msec.2019.109873
Park J, Wetzel I, Dreau D, et al., 2018, 3D miniaturization of human organs for drug discovery. Adv Healthc Mater, 7(2):1700551. https://doi.org/10.1002/adhm.201700551
Gudapati H, Dey M, Ozbolat I, 2016, A comprehensive review on droplet-based bioprinting: Past, present and future. Biomaterials, 102:20–42. https://doi.org/10.1016/j.biomaterials.2016.06.012
Gao C, Lu C, Jian Z, et al., 2021, 3D bioprinting for fabricating artificial skin tissue. Colloids Surf B Biointerfaces, 208:112041. https://doi.org/10.1016/j.colsurfb.2021.112041
Kim JH, Yoo JJ, Lee SJ, 2016, Three-dimensional cell-based bioprinting for soft tissue regeneration. Tissue Eng Regen Med, 13(6):647–662. https://doi.org/10.1007/s13770-016-0133-8
Derakhshanfar S, Mbeleck R, Xu K, et al., 2018, 3D bioprinting for biomedical devices and tissue engineering: A review of recent trends and advances. Bioact Mater, 3(2):144–156. https://doi.org/10.1016/j.bioactmat.2017.11.008
Wang Y, Yuan X, Yao B, et al., 2022, Tailoring bioinks of extrusion-based bioprinting for cutaneous wound healing. Bioact Mater, 17:178–194. https://doi.org/10.1016/j.bioactmat.2022.01.024
Fairag R, Rosenzweig DH, Ramirez-Garcialuna JL, et al., 2019, Three-dimensional printed polylactic acid scaffolds promote bone-like matrix deposition in vitro. ACS Appl Mater Interfaces, 11(17):15306–15315. https://doi.org/10.1021/acsami.9b02502
Tan SH, Ngo ZH, Sci DB, et al., 2022, Recent advances in the design of three-dimensional and bioprinted scaffolds for full-thickness wound healing. Tissue Eng. Part B, Rev, 28(1):160–181. https://doi.org/10.1089/ten.TEB.2020.0339
Guillotin B, Souquet A, Catros S, et al., 2010, Laser assisted bioprinting of engineered tissue with high cell density and microscale organization. Biomaterials, 31(28):7250–7256. https://doi.org/10.1016/j.biomaterials.2010.05.055
Zhu W, Ma X, Gou M, et al., 2016, 3D printing of functional biomaterials for tissue engineering. Curr Opin Biotechnol, 40:103–112. https://doi.org/10.1016/j.copbio.2016.03.014
Song Y, Chen X, Zhou J, et al., 2022, Research on performance of passive heat supply tower based on the back propagation neural network. Energy, 250:123762. https://doi.org/10.1016/j.energy.2022.123762
Xu J, Zheng S, Hu X, et al., 2020, Advances in the research of bioinks based on natural collagen, polysaccharide and their derivatives for skin 3D bioprinting. Polymers, 12(6):1237. https://doi.org/10.3390/polym12061237
Chen A, Qu C, Shi Y, et al., 2020, Manufacturing strategies for solid electrolyte in batteries. Front Energy Res, 8:571440. https://doi.org/10.3389/fenrg.2020.571440
Antezana PE, Municoy S, Alvarez-Echazu MI, et al., 2022, The 3D bioprinted scaffolds for wound healing. Pharmaceutics, 14(2):464. https://doi.org/10.3390/pharmaceutics14020464
Augustine R, 2018, Skin bioprinting: A novel approach for creating artificial skin from synthetic and natural building blocks. Prog Biomater, 7(2):77–92. https://doi.org/10.1007/s40204-018-0087-0
Cui X, Dean D, Ruggeri ZM, et al., 2010, Cell damage evaluation of thermal inkjet printed Chinese hamster ovary cells. Biotechnol Bioeng, 106(6):963–969. https://doi.org/10.1002/bit.22762
Ostrovidov S, Salehi S, Costantini M, et al., 2019, 3D bioprinting in skeletal muscle tissue engineering. Small, 15(24):e1805530. https://doi.org/10.1002/smll.201805530
Gao G, Cui X, 2016, Three-dimensional bioprinting in tissue engineering and regenerative medicine. Biotechnol Lett, 38(2):203–211. https://doi.org/10.1007/s10529-015-1975-1
Boland T, Xu T, Damon B, et al., 2006, Application of inkjet printing to tissue engineering. Biotechnol J, 1(9):910–917.
https://doi.org/10.1002/biot.200600081
Saunders RE, Derby B, 2014, Inkjet printing biomaterials for tissue engineering: Bioprinting. Int Mater Rev, 59(8): 430–448. https://doi.org/10.1179/1743280414y.0000000040
Gu Z, Fu J, Lin H, et al., 2020, Development of 3D bioprinting: From printing methods to biomedical applications. Asian J Pharm Sci, 15(5):529–557. https://doi.org/10.1016/j.ajps.2019.11.003
Decante G, Costa JB, Silva-Correia J, et al., 2021, Engineering bioinks for 3D bioprinting. Biofabrication, 13(3):032001. https://doi.org/10.1088/1758-5090/abec2c
He P, Zhao J, Zhang J, et al., 2018, Bioprinting of skin constructs for wound healing. Burns Trauma, 6:5. https://doi.org/10.1186/s41038-017-0104-x
Hakimi N, Cheng R, Leng L, et al., 2018, Handheld skin printer: In situ formation of planar biomaterials and tissues. Lab Chip, 18(10):1440–1451. https://doi.org/10.1039/c7lc01236e
Aljghami ME, Saboor S, Amini-Nik S, 2019, Emerging innovative wound dressings. Ann Biomed Eng, 47(3):659–675. https://doi.org/10.1007/s10439-018-02186-w
Wang X, Qi J, Zhang W, et al., 2021, 3D-printed antioxidant antibacterial carboxymethyl cellulose/epsilon-polylysine hydrogel promoted skin wound repair. Int J Biol Macromol, 187:91–104. https://doi.org/10.1016/j.ijbiomac.2021.07.115
Deng X, Gould M, Ali MA, 2022, A review of current advancements for wound healing: Biomaterial applications and medical devices. J Biomed Mater Res B Appl Biomater, 110(11):2542–2573. https://doi.org/10.1002/jbm.b.35086
Zhao H, Xu J, Yuan H, et al., 2022, 3D printing of artificial skin patches with bioactive and optically active polymer materials for anti-infection and augmenting wound repair. Mater Horiz, 9(1):342–349. https://doi.org/10.1039/d1mh00508a
Yang Z, Ren X, Liu Y, 2021, N-halamine modified ceria nanoparticles: Antibacterial response and accelerated wound healing application via a 3D printed scaffold. Compos Part B Eng, 227:109390. https://doi.org/10.1016/j.compositesb.2021.109390
He X, Yang S, Liu C, et al., 2020, Integrated wound recognition in bandages for intelligent treatment. Adv Healthc Mater, 9(22):e2000941. https://doi.org/10.1002/adhm.202000941
Wang C, Jiang X, Kim HJ, et al., 2022, Flexible patch with printable and antibacterial conductive hydrogel electrodes for accelerated wound healing. Biomaterials, 285:121479. https://doi.org/10.1016/j.biomaterials.2022.121479
Lei Q, He D, Ding L, et al., 2022, Microneedle patches integrated with biomineralized melanin nanoparticles for simultaneous skin tumor photothermal therapy and wound healing. Adv Funct Mater, 32(22):2113269. https://doi.org/10.1002/adfm.202113269
Alizadehgiashi M, Nemr CR, Chekini M, et al., 2021, Multifunctional 3D-printed wound dressings. ACS Nano, 15(7):12375–12387. https://doi.org/10.1021/acsnano.1c04499
Cereceres S, Lan Z, Bryan L, et al., 2019, Bactericidal activity of 3D-printed hydrogel dressing loaded with gallium maltolate. APL Bioeng, 3(2):026102. https://doi.org/10.1063/1.5088801
Wu Z, Hong Y, 2019, Combination of the silver-ethylene interaction and 3D printing to develop antibacterial superporous hydrogels for wound management. ACS Appl Mater Interfaces, 11(37):33734–33747. https://doi.org/10.1021/acsami.9b14090
Yang Z, Ren X, Liu Y, 2021, Multifunctional 3D printed porous GelMA/xanthan gum based dressing with biofilm control and wound healing activity. Mater Sci Eng C Mater Biol Appl, 131:112493. https://doi.org/10.1016/j.msec.2021.112493
Krishnan KA, Thomas S, 2019, Recent advances on herb-derived constituents-incorporated wound-Dressing materials: A review. Polym Adv Technol, 30(4):823–838. https://doi.org/10.1002/pat.4540
Dhivya S, Padma VV, Santhini E, 2015, Wound dressings—A review. Biomedicine (Taipei), 5(4):22. https://doi.org/10.7603/s40681-015-0022-9
Zhu J, Jiang G, Song G, et al., 2019, Incorporation of ZnO/Bioactive glass nanoparticles into alginate/chitosan composite hydrogels for wound closure. ACS Appl Biol Mater, 2(11):5042–5052. https://doi.org/10.1021/acsabm.9b00727
Teoh JH, Mozhi A, Sunil V, et al., 2021, 3D printing personalized, photocrosslinkable hydrogel wound dressings for the treatment of thermal burns. Adv Funct Mater, 31(48):2105932. https://doi.org/10.1002/adfm.202105932
Vargas AJ, Harris CC, 2016, Biomarker development in the precision medicine era: Lung cancer as a case study. Nat Rev Cancer, 16(8):525–537. https://doi.org/10.1038/nrc.2016.56
Arnedos M, Vicier C, Loi S, et al., 2015, Precision medicine for metastatic breast cancer—Limitations and solutions. Nat Rev Clin Oncol, 12(12):693–704. https://doi.org/10.1038/nrclinonc.2015.123
Afghah F, Ullah M, Seyyed Monfared Zanjani J, et al., 2020, 3D printing of silver-doped polycaprolactone-poly(propylene succinate) composite scaffolds for skin tissue engineering. Biomed Mater, 15(3):035015. https://doi.org/10.1088/1748-605X/ab7417
Xia S, Weng T, Jin R, et al., 2022, Curcumin-incorporated 3D bioprinting gelatin methacryloyl hydrogel reduces reactive oxygen species-induced adipose-derived stem cell apoptosis and improves implanting survival in diabetic wounds. Burns Trauma, 10:tkac001. https://doi.org/10.1093/burnst/tkac001
Li T, Ma H, Ma H, et al., 2019, Mussel-inspired nanostructures potentiate the immunomodulatory properties and angiogenesis of mesenchymal stem cells. ACS Appl Mater Interfaces, 11(19):17134–17146. https://doi.org/10.1021/acsami.8b22017
Ma H, Zhou Q, Chang J, et al., 2019, Grape seed-inspired smart hydrogel scaffolds for melanoma therapy and wound healing. ACS Nano, 13(4):4302–4311. https://doi.org/10.1021/acsnano.8b09496
Bergonzi C, Bianchera A, Remaggi G, et al., 2021, Biocompatible 3D printed chitosan-based scaffolds containing α-tocopherol showing antioxidant and antimicrobial activity. Appl Sci, 11(16):7253. https://doi.org/10.3390/app11167253
Ma C, Jiang L, Wang Y, et al., 2019, 3D printing of conductive tissue engineering scaffolds containing polypyrrole nanoparticles with different morphologies and concentrations. Materials (Basel), 12(15):2491. https://doi.org/10.3390/ma12152491
Xu J, Fang H, Su Y, et al., 2022, A 3D bioprinted decellularized extracellular matrix/gelatin/quaternized chitosan scaffold assembling with poly(ionic liquid)s for skin tissue engineering. Int J Biol Macromol, 220:1253–1266. https://doi.org/10.1016/j.ijbiomac.2022.08.149
Chang P, Li S, Sun Q, et al., 2022, Large full-thickness wounded skin regeneration using 3D-printed elastic scaffold with minimal functional unit of skin. J Tissue Eng, 13:20417314211063022. https://doi.org/10.1177/20417314211063022
Elemoso A, Shalunov G, Balakhovsky YM, et al., 2020, 3D Bioprinting: The roller coaster ride to commercialization. Int J Bioprint, 6(3):301. https://doi.org/10.18063/ijb.v6i3.301
Zhang Y, Wang B, Hu J, et al., 2021, 3D composite bioprinting for fabrication of artificial biological tissues. Int J Bioprint, 7(1):299. https://doi.org/10.18063/ijb.v7i1.299
Kharaziha M, Baidya A, Annabi N, 2021, Rational design of immunomodulatory hydrogels for chronic wound healing. Adv Mater, 33(39):e2100176. https://doi.org/10.1002/adma.202100176
Wang Y, Niu W, Qu X, et al., 2022, Bioactive anti-inflammatory thermocatalytic nanometal-polyphenol polypeptide scaffolds for MRSA-infection/tumor postsurgical tissue repair. ACS Appl Mater Interfaces, 14(4):4946–4958. https://doi.org/10.1021/acsami.1c21082
Xi Y, Ge J, Wang M, et al., 2020, Bioactive anti-inflammatory, antibacterial, antioxidative silicon-based nanofibrous dressing enables cutaneous tumor photothermo-chemo therapy and infection-induced wound healing. ACS Nano, 14(3):2904–2916. https://doi.org/10.1021/acsnano.9b07173
Piola B, Sabbatini M, Gino S, et al., 2022, 3D bioprinting of gelatin-xanthan gum composite hydrogels for growth of human skin cells. Int J Mol Sci, 23(1):539. https://doi.org/10.3390/ijms23010539
Tsegay F, Elsherif M, Butt H, 2022, Smart 3D printed hydrogel skin wound bandages: A review. Polymers, 14(5):1012. https://doi.org/10.3390/polym14051012
Gopinathan J, Noh I, 2018, Recent trends in bioinks for 3D printing. Biomater Res, 22:11. https://doi.org/10.1186/s40824-018-0122-1
Dey M, Ozbolat IT, 2020, 3D bioprinting of cells, tissues and organs. Sci Rep, 10(1):14023. https://doi.org/10.1038/s41598-020-70086-y
Parak A, Pradeep P, Du Toit LC, et al., 2019, Functionalizing bioinks for 3D bioprinting applications. Drug Discov Today, 24(1):198–205. https://doi.org/10.1016/j.drudis.2018.09.012
Xie Z, Gao M, Lobo AO, et al., 2020, 3D bioprinting in tissue engineering for medical applications: The classic and the hybrid. Polymers, 12(8):1717. https://doi.org/10.3390/polym12081717
Agarwala S, 2016, A perspective on 3D bioprinting technology: Present and future. Am J Eng Appl Sci, 9(4):985–990. https://doi.org/10.3844/ajeassp.2016.985.990
Daikuara LY, Chen X, Yue Z, et al., 2021, 3D bioprinting constructs to facilitate skin regeneration. Adv Funct Mater, 32(3):2105080. https://doi.org/10.1002/adfm.202105080
Pati F, Gantelius J, Svahn HA, 2016, 3D bioprinting of tissue/ organ models. Angew Chem Int Ed Engl, 55(15):4650–4665. https://doi.org/10.1002/anie.201505062
Jang KS, Park SJ, Choi JJ, et al., 2021, Therapeutic efficacy of artificial skin produced by 3D bioprinting. Materials (Basel), 14(18):5177. https://doi.org/10.3390/ma14185177