High-throughput fabrication of cell spheroids with 3D acoustic assembly devices
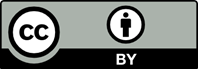
Acoustic cell assembly devices are applied in cell spheroid fabrication attributed to their rapid, label-free and low-cell damage production of size-uniform spheroids. However, the spheroids yield and production efficiency are still insufficient to meet the requirements of several biomedical applications, especially those that require large quantities of cell spheroids, such as high-throughput screening, macro-scale tissue fabrication, and tissue repair. Here, we developed a novel 3D acoustic cell assembly device combined with a gelatin methacrylamide (GelMA) hydrogels for the high-throughput fabrication of cell spheroids. The acoustic device employs three orthogonal piezoelectric transducers that can generate three orthogonal standing bulk acoustic waves to create a 3D dot-array (25 × 25 × 22) of levitated acoustic nodes, enabling large-scale fabrication of cell aggregates (>13,000 per operation). The GelMA hydrogel serves as a supporting scaffold to preserve the structure of cell aggregates after the withdrawal of acoustic fields. As a result, mostly cell aggregates (>90%) mature into spheroids maintaining good cell viability. We further applied these acoustically assembled spheroids to drug testing to explore their potency in drug response. In conclusion, this 3D acoustic cell assembly device may pave the way for the scale-up fabrication of cell spheroids or even organoids, to enable flexible application in various biomedical applications, such as high-throughput screening, disease modeling, tissue engineering, and regenerative medicine.
1. Rodenhizer D, Dean T, D’Arcangelo E, et al., 2018, The current landscape of 3D in vitro tumor models: What cancer hallmarks are accessible for drug discovery?.Adv Healthc Mater, 7(8):e1701174. https://doi.org/10.1002/adhm.201701174
2. Rodoplu D, Matahum JS, Hsu CH, 2022, A microfluidic hanging drop-based spheroid co-culture platform for probing tumor angiogenesis. Lab Chip, 22(7):1275–1285. https://doi.org/10.1039/d1lc01177d
3. Kim W, Gwon Y, Park S, et al., 2023, Therapeutic strategies of three-dimensional stem cell spheroids and organoids for tissue repair and regeneration. Bioact Mater, 19:50–74. https://doi.org/10.1016/j.bioactmat.2022.03.039
4. Lin R, Chou L, Chien CM, et al., 2006, Dynamic analysis of hepatoma spheroid formation: Roles of E-cadherin and beta1-integrin. Cell Tissue Res, 324(3):411–422. https://doi.org/10.1007/s00441-005-0148-2
5. Huang B, Wei X, Chen K, et al., 2023, Bioprinting of hydrogel beads to engineer pancreatic tumor-stroma microtissues for drug screening. Int J Bioprint. 9(3):676. https://doi.org/10.1016/j.jconrel.2017.12.005
6. Li S, Yang K, Chen X, et al., 2021, Simultaneous 2D and 3D cell culture array for multicellular geometry, drug discovery and tumor microenvironment reconstruction. Biofabrication, 13(4):045013. https://doi.org/10.1088/1758-5090/ac1ea8
7. Szűcs D, Fekete Z, Guba M, et al., 2023, Toward better drug development: Threedimensional bioprinting in toxicological research. Int J Bioprint, 9(2):663. https://doi.org/10.18063/ijb.v9i2.663
8. Kim SJ, Kim EM, Yamamoto M, et al., 2020, Engineering multi-cellular spheroids for tissue engineering and regenerative medicine. Adv Healthc Mater, 9(23):2000608. https://doi.org/10.1002/adhm.202000608
9. Banerjee D, Singh YP, Datta P, et al., 2022, Strategies for 3D bioprinting of spheroids: A comprehensive review. Biomaterials, 291:121881. https://doi.org/10.1016/j.biomaterials.2022.121881
10. Zhuang P, Chiang YH, Fernanda MS, et al., 2021, Using spheroids as building blocks towards 3D bioprinting of tumor microenvironment. Int J Bioprint, 7(4):444. http://doi.org/10.18063/ijb.v7i4.444
11. Skylar-Scott MA, Uzel SGM, Nam LL, et al., 2019, Biomanufacturing of organ-specific tissues with high cellular density and embedded vascular channels. Sci Adv, 5(9):eaaw2459. https://doi.org/10.1126/sciadv.aaw2459
12. Ayan B, Heo DN, Zhang Z, et al., 2020, Aspiration-assisted bioprinting for precise positioning of biologics. Sci Adv, 6(10):eaaw5111. https://doi.org/10.1126/sciadv.aaw5111
13. Chen K, Jiang E, Wei X, et al., 2021, The acoustic droplet printing of functional tumor microenvironments. Lab Chip, 21(8):1604–1612. https://doi.org/10.1039/d1lc00003a
14. Daly AC, Davidson MD, Burdick JA, 2021, 3D bioprinting of high cell-density heterogeneous tissue models through spheroid fusion within self-healing hydrogels. Nat Commun, 12(1):753. https://doi.org/10.1038/s41467-021-21029-2
15. Li L, Chen Y, Wang H, et al., 2021, A high-throughput, open-space and reusable microfluidic chip for combinational drug screening on tumor spheroids. Lab Chip, 21(20):3924–3932. https://doi.org/10.1039/d1lc00525a
16. Singh SK, Abbas S, Saxena AK, et al., 2020, Critical role of three-dimensional tumorsphere size on experimental outcome. BioTechniques, 69(5):333–338. https://doi.org/10.2144/btn-2020-0081
17. Pyo DH, Hong HK, Lee WY, et al., 2020, Patient-derived cancer modeling for precision medicine in colorectal cancer: Beyond the cancer cell line. Cancer Biol Ther, 21(6):495–502. https://doi.org/10.1080/15384047.2020.1738907
18. Brassard JA, Nikolaev M, Hubscher T, et al., 2021, Recapitulating macro-scale tissue self-organization through organoid bioprinting. Nat Mater, 20(1):22–29. https://doi.org/10.1038/s41563-020-00803-5
19. Han SJ, Kwon S, Kim KS, 2021, Challenges of applying multicellular tumor spheroids in preclinical phase. Cancer Cell Int, 21(1):152. https://doi.org/10.1186/s12935-021-01853-8
20. Feng L, Liang S, Zhou Y, et al, 2020, Three-dimensional printing of hydrogel scaffolds with hierarchical structure for scalable stem cell culture. ACS Biomater Sci Eng, 6(5):2995–3004. https://doi.org/10.1021/acsbiomaterials.9b01825
21. Shen H, Cai S, Wu C, et al., 2021, Recent advances in three-dimensional multicellular spheroid culture and future development. Micromachines, 12(1):96. https://doi.org/10.3390/mi12010096
22. Ryu NE, Lee SH, Park H, 2019, Spheroid culture system methods and applications for mesenchymal stem cells. Cells, 8(12):1620. https://doi.org/10.3390/cells8121620
23. Rodrigues T, Kundu B, Silva-Correia J, et al., 2018, Emerging tumor spheroids technologies for 3D in vitro cancer modeling. Pharmacol Therapeut, 184:201–211. https://doi.org/10.1016/j.pharmthera.2017.10.018
24. Foty R, 2011, A simple hanging drop cell culture protocol for generation of 3D spheroids. J Vis Exp, 51:e2720. https://doi.org/10.3791/2720
25. Tung YC, Hsiao AY, Allen SG, et al., 2011, High-throughput 3D spheroid culture and drug testing using a 384 hanging drop array. Analyst, 136(3):473–478. https://doi.org/10.1039/c0an00609b
26. He H, He Q, Xu F, et al., 2019, Dynamic formation of cellular aggregates of chondrocytes and mesenchymal stem cells in spinner flask. Cell Proliferat, 52(4):e12587. https://doi.org/10.1111/cpr.12587
27. Curcio E, Salerno S, Barbieri G, et al., 2007, Mass transfer and metabolic reactions in hepatocyte spheroids cultured in rotating wall gas-permeable membrane system. Biomaterials, 28(36):5487–5497. https://doi.org/10.1016/j.biomaterials.2007.08.033
28. Napolitano AP, Dean DM, Man AJ, et al., 2007, Scaffold-free three-dimensional cell culture utilizing micromolded nonadhesive hydrogels. BioTechniques, 43(4):494, 496–500. https://doi.org/10.2144/000112591
29. Wu Y, Zhou Y, Qin X, et al., 2021, From cell spheroids to vascularized cancer organoids: Microfluidic tumor-on-a-chip models for preclinical drug evaluations. Biomicrofluidics, 15(6):061503. https://doi.org/10.1063/5.0062697
30. Prince E, Kheiri S, Wang Y, et al., 2022, Microfluidic arrays of breast tumor spheroids for drug screening and personalized cancer therapies. Adv Healthc Mater, 11(1):e2101085. https://doi.org/10.1002/adhm.202101085
31. Yin F, Zhang X, Wang L, et al., 2021, HiPSC-derived multi-organoids-on-chip system for safety assessment of antidepressant drugs. Lab Chip, 21(3):571–581. https://doi.org/10.1039/d0lc00921k
32. Albrecht DR, Underhill GH, Wassermann TB, et al., 2006, Probing the role of multicellular organization in three-dimensional microenvironments. Nat Methods, 3(5): 369–375. https://doi.org/10.1038/nmeth873
33. Tocchio A, Durmus NG, Sridhar K, et al., 2018, Magnetically guided self-assembly and coding of 3D living architectures. Adv Mater, 30(4):1705034. https://doi.org/10.1002/adma.201705034
34. Parfenov VA, Koudan EV, Bulanova EA, et al., 2018, Scaffold-free, label-free and nozzle-free biofabrication technology using magnetic levitational assembly. Biofabrication, 10(3):034104. https://doi.org/10.1088/1758-5090/aac900
35. Souza GR, Molina JR, Raphael RM, et al., 2010, Three-dimensional tissue culture based on magnetic cell levitation. Nat Nanotechnol, 5(4):291–296. https://doi.org/10.1038/nnano.2010.23
36. Chen K, Wu M, Guo F, et al., 2016, Rapid formation of size-controllable multicellular spheroids via 3D acoustic tweezers. Lab Chip, 16(14):2636–2643. https://doi.org/10.1039/c6lc00444j
37. Olofsson K, Carannante V, Ohlin M, et al., 2018, Acoustic formation of multicellular tumor spheroids enabling on-chip functional and structural imaging. Lab Chip, 18(16): 2466–2476. https://doi.org/10.1039/c8lc00537k
38. Chen B, Wu Y, Ao Z, et al., 2019, High-throughput acoustofluidic fabrication of tumor spheroids. Lab Chip, 19(10):1755–1763. https://doi.org/10.1039/c9lc00135b
39. Armstrong JPK, Maynard SA, Pence IJ, et al., 2019, Spatiotemporal quantification of acoustic cell patterning using Voronoï tessellation. Lab Chip, 19(4):562–573. https://doi.org/10.1039/C8LC01108G
40. Bouyer C, Chen P, Guven S, et al., 2016, A bio-acoustic levitational (BAL) assembly method for engineering of multilayered, 3D brain-like constructs, using human embryonic stem cell derived neuro-progenitors. Adv Mater, 28(1):161–167. https://doi.org/10.1002/adma.201503916
41. Sriphutkiat Y, Kasetsirikul S, Zhou YF, 2018, Formation of cell spheroids using standing surface acoustic wave (SSAW). Int J Bioprint, 4(1):130. http://dx.doi.org/10.18063/IJB.v4i1.130
42. Wu Z, Chen B, Wu Y, et al., 2021, Scaffold-free generation of heterotypic cell spheroids using acoustofluidics. Lab Chip, 21(18):3498–3508. https://doi.org/10.1039/d1lc00496d
43. Wu Y, Ao Z, Bin C, et al., 2018, Acoustic assembly of cell spheroids in disposable capillaries. Nanotechnology, 29(50):504006. https://doi.org/10.1088/1361-6528/aae4f1
44. Hu X, Zhao S, Luo Z, et al., 2020, On-chip hydrogel arrays individually encapsulating acoustic formed multicellular aggregates for high throughput drug testing. Lab Chip, 20(12):2228–2236. https://doi.org/10.1039/d0lc00255k
45. Chansoria P, Narayanan LK, Schuchard K, et al., 2019, Ultrasound-assisted biofabrication and bioprinting of preferentially aligned three-dimensional cellular constructs. Biofabrication, 11:035015. https://doi.org/10.1088/1758-5090/ab15cf
46. Wu Z, Jiang H, Zhang L, et al., 2019, The acoustofluidic focusing and separation of rare tumor cells using transparent lithium niobate transducers. Lab Chip, 19(23): 3922–3930. https://doi.org/10.1039/c9lc00874h
47. Jeger-Madiot N, Arakelian L, Setterblad N, et al., 2021, Self-organization and culture of mesenchymal stem cell spheroids in acoustic levitation. Sci Rep, 11(1):8355. https://doi.org/10.1038/s41598-021-87459-6
48. Cai H, Ao Z, Hu L, et al., 2020, Acoustofluidic assembly of 3D neurospheroids to model Alzheimer’s disease. Analyst, 145(19):6243–6253. https://doi.org/10.1039/d0an01373k
49. Ying G, Jiang N, Yu C, et al., 2018, Three-dimensional bioprinting of gelatin methacryloyl (GelMA). Bio-Des Manuf, 1(4):215–224. https://doi.org/10.1007/s42242-018-0028-8
50. Wei X, Huang B, Chen K, et al., 2022, Dot extrusion bioprinting of spatially controlled heterogenous tumor models. Mater Design, 223:111152. https://doi.org/10.1016/j.matdes.2022.111152
51. Xu J, Ji L, Liang Y, et al., 2020, CircRNA-SORE mediates sorafenib resistance in hepatocellular carcinoma by stabilizing YBX1. Signal Transduct Tar, 5:298. https://doi.org/10.1038/s41392-020-00375-5
52. Nishikawa H, Nishijima N, Enomoto H, et al., 2017, Prognostic significance of sarcopenia in patients with hepatocellular carcinoma undergoing sorafenib therapy. Oncol Lett, 14(2):1637–1647. https://doi.org/10.3892/ol.2017.6287