3D printing of drug-eluting bioactive multifunctional coatings for orthopedic applications
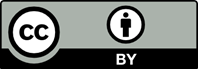
Three-dimensional (3D) printing is implemented for surface modification of titanium alloy substrates with multilayered biofunctional polymeric coatings. Poly(lactic-coglycolic) acid (PLGA) and polycaprolactone (PCL) polymers were embedded with amorphous calcium phosphate (ACP) and vancomycin (VA) therapeutic agents to promote osseointegration and antibacterial activity, respectively. PCL coatings revealed a uniform deposition pattern of the ACP-laden formulation and enhanced cell adhesion on the titanium alloy substrates as compared to the PLGA coatings. Scanning electron microscopy and Fourier-transform infrared spectroscopy confirmed a nanocomposite structure of ACP particles showing strong binding with the polymers. Cell viability data showed comparable MC3T3 osteoblast proliferation on polymeric coatings as equivalent to positive controls. In vitro live/dead assessment indicated higher cell attachments for 10 layers (burst release of ACP) as compared to 20 layers (steady release) for PCL coatings. The PCL coatings loaded with the antibacterial drug VA displayed a tunable release kinetics profile based on the multilayered design and drug content of the coatings. Moreover, the concentration of active VA released from the coatings was above the minimum inhibitory concentration and minimum bactericidal concentration, demonstrating its effectiveness against Staphylococcus aureus bacterial strain. This research provides a basis for developing antibacterial biocompatible coatings to promote osseointegration of orthopedic implants.
1. Bose S, Robertson SF, Bandyopadhyay A, 2018, Surface modification of biomaterials and biomedical devices using additive manufacturing. Acta Biomater, 66: 6–22. https://doi.org/10.1016/J.ACTBIO.2017.11.003
2. Xue P, Li Q, Li Y, et al., 2017, Surface modification of poly(dimethylsiloxane) with polydopamine and hyaluronic acid to enhance hemocompatibility for potential applications in medical implants or devices. ACS Appl Mater Interfaces, 9: 33632–33644. https://doi.org/10.1021/ACSAMI.7B10260
3. Xiao M, Chen YM, Biao MN, et al., 2017, Bio-functionalization of biomedical metals. Mater Sci Eng C Mater Biol Appl, 70: 1057–1070. https://doi.org/10.1016/J.MSEC.2016.06.067
4. Adarkwa E, Kotoka R, Desai S, 2021, 3D printing of polymeric coatings on AZ31 Mg alloy substrate for corrosion protection of biomedical implants. Med Dev Sens, 4: e10167. https://doi.org/10.1002/MDS3.10167
5. Desai S, Shankar MR, 2021, Emerging trends in polymers, composites, and nano biomaterial applications. In: Bio- Materials and Prototyping Applications in Medicine. Germany: Springer. p.19–34. https://doi.org/10.1007/978-3-030-35876-1_2
6. Aldawood FK, Andar A, Desai S, 2021, A comprehensive review of microneedles: Types, materials, processes, characterizations and applications. Polymers (Basel), 13: 2815. https://doi.org/10.3390/POLYM13162815
7. Parupelli SK, Aljohani A, Khanal K, et al., 2019, Direct Jet Printing and Characterization of Calcium Alginate Microcapsules for Biomedical Applications. In: IIE Annual Conference Proceedings. United States: Institute of Industrial and Systems Engineers (IISE). pp.300–305.
8. Marquetti I, Desai S, 2018, Molecular modeling the adsorption behavior of bone morphogenetic protein-2 on hydrophobic and hydrophilic substrates. Chem Phys Lett, 706: 285–294. https://doi.org/10.1016/J.CPLETT.2018.06.015
9. Marquetti I, Desai S, 2018, Adsorption behavior of bone morphogenetic Protein-2 on a graphite substrate for biomedical applications. Am J Eng Appl Sci, 11: 1037–1044. https://doi.org/10.3844/AJEASSP.2018.1037.1044
10. Aljohani A, Desai S, 2018, 3D printing of porous scaffolds for medical applications. Am J Eng Appl Sci, 11: 1076–1085. https://doi.org/10.3844/AJEASSP.2018.1076.1085
11. Biomedical Applications of Titanium and its Alloys. Available from: https://www.tms.org/jom.html [Last accessed on 2022 Mar 27].
12. Niinomi M, 2002, Recent metallic materials for biomedical applications. Metallurgical Mater Trans A 33: 477–486. https://doi.org/10.1007/S11661-002-0109-2
13. Desai S, Bidanda B, Bártolo P, 2008, Metallic and ceramic biomaterials: Current and future developments. In: Bio- Materials and Prototyping Applications in Medicine. Berlin: Springer. pp.1–14. https://doi.org/10.1007/978-0-387-47683-4_1
14. Gu Y, Chen X, Lee JH, et al., 2012, Inkjet printed antibiotic-and calcium-eluting bioresorbable nanocomposite micropatterns for orthopedic implants. Acta Biomater, 8: 424–431. https://doi.org/10.1016/J.ACTBIO.2011.08.006
15. Benoit MA, Mousset B, Delloye C, et al., 1998, Antibiotic-loaded plaster of Paris implants coated with poly lactide-co-glycolide as a controlled release delivery system for the treatment of bone infections. Int Orthop, 21: 403. https://doi.org/10.1007/S002640050195
16. Dion A, Langman M, Hall G, et al., 2005, Vancomycin release behaviour from amorphous calcium polyphosphate matrices intended for osteomyelitis treatment. Biomaterials, 26: 7276–7285. https://doi.org/10.1016/J.BIOMATERIALS.2005.05.072
17. Tang LH, Zhao CH, Xiong Y, et al., 2009, Preparation, release profiles, and antibacterial properties of vancomycin-loaded poly(D,L-lactic) titanium alloy plates. Orthopedics, 32: 324. https://doi.org/10.3928/01477447-20090501-16
18. Jiang J, Li YF, Fang TL, et al., 2012, Vancomycin-loaded nano-hydroxyapatite pellets to treat MRSA-induced chronic osteomyelitis with bone defect in rabbits. Inflamm Res, 61: 207–215. https://doi.org/10.1007/S00011-011-0402-X/FIGURES/6
19. Soundrapandian C, Datta S, Sa B, 2007, Drug-eluting implants for osteomyelitis. Crit Rev Ther Drug Carrier Syst, 24: 493–545. https://doi.org/10.1615/critrevtherdrugcarriersyst.V24. I6.10
20. Zhao L, Chu PK, Zhang Y, et al., 2009, Antibacterial coatings on titanium implants. J Biomed Mater Res B Appl Biomater, 91: 470–480. https://doi.org/10.1002/JBM.B.31463
21. Hetrick EM, Schoenfisch MH, 2006, Reducing implant-related infections: Active release strategies. Chem Soc Rev, 35: 780–789. https://doi.org/10.1039/B515219B
22. Lewis K, 2007, Persister cells, dormancy and infectious disease. Nat Rev Microbiol, 5: 48–56. https://doi.org/10.1038/NRMICRO1557
23. Dunne WM, 2002, Bacterial adhesion: Seen any good biofilms lately? Clin Microbiol Rev, 15: 155–166. https://doi.org/10.1128/CMR.15.2.155-166.2002
24. Antimicrobial and Wound Healing Properties of Nitric Oxide-Releasing Xerogels and Silica Nanoparticles Carolina Digital Repository, Dissertation or Thesis. p.47429941z. Available from: https://www.cdr.lib.unc.edu/concern/ dissertations/47429941z [Last accessed on 2022 Mar 27].
25. Schairer DO, Chouake JS, Nosanchuk JD, et al., 2012, The potential of nitric oxide releasing therapies as antimicrobial agents. Virulence, 3: 271. https://doi.org/10.4161/VIRU.20328
26. Desai S, Perkins J, Harrison BS, et al., 2010, Understanding release kinetics of biopolymer drug delivery microcapsules for biomedical applications. Mater Sci Eng B, 168: 127–131. https://doi.org/10.1016/J.MSEB.2009.11.006
27. Marquetti I, Desai S, 2022, An atomistic investigation of adsorption of bone morphogenetic Protein-2 on gold with nanoscale topographies. Surfaces, 5: 176–185. https://doi.org/10.3390/SURFACES5010010
28. Marquetti I, Desai S, 2022, Nanoscale topographical effects on the adsorption behavior of bone morphogenetic Protein-2 on graphite. Int J Mol Sci, 23: 2432. https://doi.org/10.3390/IJMS23052432
29. Marquetti I, Desai S, 2019, Orientation effects on the nanoscale adsorption behavior of bone morphogenetic Protein-2 on hydrophilic silicon dioxide. RSC Adv, 9: 906– 916. https://doi.org/10.1039/C8RA09165J
30. Desai S, Bidanda B, Bártolo PJ, 2021, Emerging trends in the applications of metallic and ceramic biomaterials. In: Bio- Materials and Prototyping Applications in Medicine. Berlin: Springer. pp.1-17. https://doi.org/10.1007/978-3-030-35876-1_1
31. Laser Fabrication of Polymeric Microneedles for Transdermal Drug Delivery DOE PAGES. Available from: https://www.par.nsf.gov/biblio/10297495 [Last accessed on 2022 Apr 08].
32. Rodrigues J, Desai S, 2019, The nanoscale leidenfrost effect. Nanoscale, 11: 12139–12151. https://doi.org/10.1039/C9NR01386E
33. Gaikwad A, Desai S, 2021, Molecular dynamics investigation of the deformation mechanism of gold with variations in mold profiles during nanoimprinting. Materials (Basel), 14: 2548. https://doi.org/10.3390/MA14102548
34. Desai SS, Marquetti I, Desai S, 2016, Molecular Modeling of Biochemical Cues Using High Performance Computing Molecular Modeling of biochemical cues using High Performance GPU. Available from: https://www. researchgate.net/publication/316656312 [Last accessed 2022 Apr 07].
35. Cordeiro J, Desai S, 2017, The effect of water droplet size, temperature, and impingement velocity on gold wettability at the nanoscale. J Micro Nano Manuf. 25: 031008. https://doi.org/10.1115/1.4036891/369684
36. Neut D, Kluin OS, Crielaard BJ, et al., 2009, A biodegradable antibiotic delivery system based on poly-(trimethylene carbonate) for the treatment of osteomyelitis. Acta Orthop, 80: 514. https://doi.org/10.3109/17453670903350040
37. Price JS, Tencer AF, Arm DM, et al., Controlled release of antibiotics from coated orthopedic implants. J Biomed Mater Res, 30: 281–286. https://doi.org/10.1002/(SICI)1097-4636(199603)30:3
38. Lucke M, Wildemann B, Sadoni S, et al., 2005, Systemic versus local application of gentamicin in prophylaxis of implant-related osteomyelitis in a rat model. Bone, 36: 770–778. https://doi.org/10.1016/J.BONE.2005.01.008
39. Sánchez E, Baro M, Soriano I, et al., 2001, In vivo-in vitro study of biodegradable and osteointegrable gentamicin bone implants. Eur J Pharm Biopharm, 52: 151–158. https://doi.org/10.1016/S0939- 6411(01)00169-2
40. Perkins J, Xu Z, Roy A, et al., 2014, Polymeric Coatings for Biodegradable Implants. Montreal, CANADA: AES-ATEMA 17th International Conference Advances and Trends in Engineering Materials and their Applications.
41. Perkins J, Desai S, Xu Z, et al., 2012, Controlling Corrosion Mechanism of Biodegradable Magnesium Alloys for Implant Applications. In: IIE Annual Conference Proceedings. United States: Institute of Industrial and Systems Engineers (IISE). p.1.
42. Perkins J, Desai S, Wagner W, et al., 2011, Biomanufacturing: Direct-writing of Controlled Release Coatings for Cardiovascular (Stents) Applications. In IIE Annual Conference Proceedings. United States: Institute of Industrial and Systems Engineers (IISE). p. 1.
43. Jiang T, Munguia-Lopez JG, Flores-Torres S, et al., 2019, Extrusion bioprinting of soft materials: An emerging technique for biological model fabrication. Appl Phys Rev, 6: 011310. https://doi.org/10.1063/1.5059393
44. Zhuang P, Ng WL, An J, et al., 2019, Layer-by-layer ultraviolet assisted extrusion-based (UAE) bioprinting of hydrogel constructs with high aspect ratio for soft tissue engineering applications. PLoS One, 14: e0216776. https://doi.org/10.1371/JOURNAL.PONE.0216776
45. Li X, Liu B, Pei B, et al., Inkjet bioprinting of biomaterials. Chem Rev, 120: 10793–10833. https://doi.org/10.1021/acs.chemrev.0c00008/asset/images/ large/cr0C00008_0028.JPEG
46. Ng WL, Huang X, Shkolnikov V, et al., 2022, Controlling droplet impact velocity and droplet volume: Key factors to achieving high cell viability in sub-nanoliter droplet-based bioprinting. Int J Bioprint, 8: 424. https://doi.org/10.18063/IJB.V8I1.424
47. Li W, Mille LS, Robledo JA, et al., 2020, Recent advances in formulating and processing biomaterial inks for vat polymerization-based 3D printing. Adv Healthc Mater, 9: 2000156. https://doi.org/10.1002/ADHM.202000156
48. Cooley P, Wallace D, Antohe B, 2016, Applicatons of ink-jet printing technology to BioMEMS and microfluidic systems. J Assoc Lab Auto, 7: 33–39. https://doi./org/10.1016/S1535-5535-04-00214-X
49. Fuller SB, Wilhelm EJ, Jacobson JM, 2002, Ink-jet printed nanoparticle microelectromechanical systems. J Microelectromech Syst, 11: 54–60.
50. Hebner TR, Wu CC, Marcy D, et al., 2022, Ink-jet Printing of Doped Polymers for Organic Light Emitting Devices. Available from: https://www.ojps.aip.org/aplo/aplcr.jsp [Last accessed on 2022 Mar 27].
51. Wallace D, 2005, Ink-jet Printing as a Tool in Manufacturing and Instrumentation. Nanolithography and Patterning Techniques in Microelectronics. Netherlands: Elsevier. p.267.
52. Perkins J, Hong Y, Ye SH, et al., 2014, Direct writing of bio-functional coatings for cardiovascular applications. J Biomed Mater Res Part A, 102: 4290–4300. https://doi.org/10.1002/JBM.A.35105
53. Perkins J, Xu Z, Smith C, et al., 2015, Direct writing of polymeric coatings on magnesium alloy for tracheal stent applications. Ann Biomed Eng, 43: 1158–1165. https://doi.org/10.1007/S10439-014-1169-3/figures/6
54. Kazemzadeh-Narbat M, Noordin S, Masri BA, et al., 2012, Drug release and bone growth studies of antimicrobial peptide-loaded calcium phosphate coating on titanium. J Biomed Mater Res Part B Appl Biomater, 100B: 1344–1352. https://doi.org/10.1002/JBM.B.32701
55. Le Ray AM, Chiffoleau S, Iooss P, et al., 2003, Vancomycin encapsulation in biodegradable poly(ε-caprolactone) microparticles for bone implantation. Influence of the formulation process on size, drug loading, in vitro release and cytocompatibility. Biomaterials, 24: 443–449. https://doi.org/10.1016/S0142-9612(02)00357-5
56. Kim HW, Knowles JC, Kim HE, 2005, Hydroxyapatite porous scaffold engineered with biological polymer hybrid coating for antibiotic Vancomycin release. J Mater Sci Mater Med, 16: 189–195. https://doi.org/10.1007/S10856-005-6679-Y
57. De Gans BJ, Duineveld PC, Schubert US, 2004, Inkjet printing of polymers: State of the art and future developments. Adv Mater, 16: 203–213. https://doi.org/10.1002/ADMA.200300385
58. Adamczyk M, Brate EM, Chiappetta EG, et al., 1997, Development of a quantitative vancomycin immunoassay for the Abbott AxSYM analyzer. Ther Drug Monit, 19: 571. https://doi.org/10.1097/00007691-199710000-00106
59. Lawson MC, Bowman CN, Anseth KS, 2007, Vancomycin derivative photopolymerized to titanium kills S. epidermidis. Clin Orthop Rel Res, 461: 96–105. https://doi.org/10.1097/BLO.0B013E3180986706
60. Monzón M, Oteiza C, Leiva J, et al., 2001, Synergy of different antibiotic combinations in biofilms of Staphylococcus epidermidis. J Antimicrob Chemother, 48: 793–801. https://doi.org/10.1093/JAC/48.6.793
61. Xie Z, Liu X, Jia W, et al., 2009, Treatment of osteomyelitis and repair of bone defect by degradable bioactive borate glass releasing vancomycin. J Control Rel, 139: 118–126. https://doi.org/10.1016/J.JCONREL.2009.06.012
62. Headquarters, AST, 1976, Adhesion Measurement of Thin Films, Thick Fi Lms, an D Bulk Coatings. Vol. 2. PA: Astm Special Technical Publication. p. 4.
63. ASTM International, 2017, ASTM D3359-17 Standard Test Methods for Rating Adhesion by Tape Test. United States: ASTM International. pp.1–9.
64. Page B, Page M, Noel C, 1993, A new fluorometric assay for cytotoxicity measurements in vitro. Int J Oncol, 3: 473–476. https://doi.org/10.3892/IJO.3.3.473
65. Adams CS, Antoci Jr V, Harrison G, et al., 2009, Controlled release of vancomycin from thin sol‐gel films on implant surfaces successfully controls osteomyelitis. J Orthop Res, 27: 701–709.
66. Li B, Brown KV, Wenke JC, et al., 2010, Sustained release of vancomycin from polyurethane scaffolds inhibits infection of bone wounds in a rat femoral segmental defect model. J Control Release, 145: 221–230. https://doi.org/10.1016/J.JCONREL.2010.04.002
67. Ruiz JC, Alvarez-Lorenzo C, Taboada P, et al., 2008, Polypropylene grafted with smart polymers (PNIPAAm/ PAAc) for loading and controlled release of vancomycin. Eur J Pharm Biopharm, 70: 467–477. https://doi.org/10.1016/J.EJPB.2008.05.020
68. Meejoo S, Maneeprakorn W, Winotai P, 2006, Phase and thermal stability of nanocrystalline hydroxyapatite prepared via microwave heating. Thermochimica Acta, 447: 115–120. https://doi.org/10.1016/J.TCA.2006.04.013
69. Roy A, Jhunjhunwala S, Bayer E, et al., 2016, Porous calcium phosphate-poly (lactic-co-glycolic) acid composite bone cement: A viable tunable drug delivery system. Mater Sci Eng C Mater Biol Appl, 59: 92–101. https://doi.org/10.1016/J.MSEC.2015.09.081
70. Dorozhkin S v. Amorphous calcium (ortho)phosphates. Acta Biomaterialia. 2010;6(12):4457-4475. doi:10.1016/J. ACTBIO.2010.06.031
71. Habibovic P, Barralet JE, 2011, Bioinorganics and biomaterials: Bone repair. Acta Biomater, 7: 3013–3026. https://doi.org/10.1016/J.ACTBIO.2011.03.027
72. Hoppe A, Güldal NS, Boccaccini AR, 2011, A review of the biological response to ionic dissolution products from bioactive glasses and glass-ceramics. Biomaterials, 32: 2757–2774. https://doi.org/10.1016/J.BIOMATERIALS.2011.01.004
73. Lee SH, Lee JE, Baek WY, et al., 2004, Regional delivery of vancomycin using pluronic F-127 to inhibit methicillin resistant Staphylococcus aureus (MRSA) growth in chronic otitis media in vitro and in vivo. J Controlled Release, 96: 1–7. https://doi.org/10.1016/J.JCONREL.2003.12.029
74. Ginebra MP, Traykova T, Planell JA, 2006, Calcium phosphate cements as bone drug delivery systems: A review. J Control Release, 113: 102–110. https://doi.org/10.1016/J.JCONREL.2006.04.007
75. Edin ML, Miclau T, Lester GE, et al., 1996, Effect of cefazolin and vancomycin on osteoblasts in vitro. Clin Orthop Relat Res, 333: 245–251.
76. Antoci V Jr., Adams CS, Hickok NJ, et al., 2007, Antibiotics for local delivery systems cause skeletal cell toxicity in vitro. Clin Orthop Rel Res, 462: 200–206. https://doi.org/10.1097/BLO.0B013E31811FF866
77. Haleem AA, Rouse MS, Lewallen DG, et al., 2004, Gentamicin and vancomycin do not impair experimental fracture healing. Clin Orthop Rel Res,427: 22–24. https://doi.org/10.1097/01.BLO.0000144477.43661.59
78. McLaren JS, White LJ, Cox HC, et al., 2014, A biodegradable antibiotic-impregnated scaffold to prevent osteomyelitis in a contaminated in vivo bone defect model. Eur Cells Mater, 27: 332–349. https://doi.org/10.22203/ECM.V027A24
79. Mason EO, Lamberth LB, Hammerman WA, et al., 2009, Vancomycin MICs for Staphylococcus aureus vary by detection method and have subtly increased in a pediatric population since 2005. J Clin Microbiol, 47: 1628–1630. https://doi.org/10.1128/JCM.00407-09
80. Moise PA, North D, Steenbergen JN, et al., 2009, Susceptibility relationship between vancomycin and daptomycin in Staphylococcus aureus: Facts and assumptions. Lancet Infect Dis, 9: 617–624. https://doi.org/10.1016/S1473-3099(09)70200-2
81. Peña J, Corrales T, Izquierdo-Barba I, et al., 2006, Long term degradation of poly(ɛ-caprolactone) films in biologically related fluids. Polym Degradation Stability, 91: 1424–1432. https://doi.org/10.1016/J.POLYMDEGRADSTAB.2005.10.016
82. Yeh ML, Chen KH, Chen HD, et al., 2013, Prolonged antibiotic release by PLGA encapsulation on titanium alloy. J Med Biol Eng, 2013 33(1): p 5.
83. Kim HW, Lee EJ, Jun IK, et al., 2005, Degradation and drug release of phosphate glass/polycaprolactone biological composites for hard-tissue regeneration. J Biomed Mater Res Part B Appl Biomater, 75B: 34–41. https://doi.org/10.1002/JBM.B.30223
84. Fang T, Wen J, Zhou J, et al., 2012, Poly (ε-caprolactone) coating delays vancomycin delivery from porous chitosan/ β-tricalcium phosphate composites. J Biomed Mater Res Part B Appl Biomater, 100B: 1803–1811. https://doi.org/10.1002/JBM.B.32747
85. Uskokovic̈ V, Desai TA, 2013, Phase composition control of calcium phosphate nanoparticles for tunable drug delivery kinetics and treatment of osteomyelitis. II. Antibacterial and osteoblastic response. J Biomed Mater Res A, 101: 1427–1436. https://doi.org/10.1002/JBM.A.34437
86. Kirkham J, Brookes SJ, Shore RC, et al., 2002, Physico-chemical properties of crystal surfaces in matrix–mineral interactions during mammalian biomineralisation. Curr Opin Colloid Interface Sci, 7: 124–132.
87. Dos Santos EA, Farina M, Soares GA, et al., 2008, Surface energy of hydroxyapatite and β-tricalcium phosphate ceramics driving serum protein adsorption and osteoblast adhesion. J Mater Sci Mater Med, 19: 2307–2316. https://doi.org/10.1007/S10856-007-3347-4/FIGURES/7
88. Mueller B, Zacharias M, Rezwan K, 2010, Bovine serum albumin and lysozyme adsorption on calcium phosphate particles. Adv Eng Mater, 12: B53–B61. https://doi.org/10.1002/ADEM.200980024
89. Combes C, Rey C, 2010, Amorphous calcium phosphates: Synthesis, properties and uses in biomaterials. Acta Biomater, 6: 3362–3378. https://doi.org/10.1016/J.ACTBIO.2010.02.017