Development of an alginate–gelatin bioink enhancing osteogenic differentiation by gelatin release
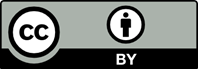
Hydrogels are natural bioink options for cellular printing due to their high-water content and permeable three-dimensional (3D) polymeric structure, which are favorable for cellular anchoring and metabolic activities. To increase the functionality of hydrogels as bioinks, biomimetic components are often incorporated, such as proteins, peptides, and growth factors. In this study, we aimed to enhance the osteogenic activity of a hydrogel formulation by integrating both the release and retention of gelatin so that gelatin serves as both an indirect support for released ink component on cells nearby and a direct support for encapsulated cells inside a printed hydrogel, thereby fulfills two functions. Methacrylate-modified alginate (MAalginate) was chosen as the matrix because it has a low cell adhesion effect due to the absence of ligands. The gelatin-containing MA-alginate hydrogel was fabricated, and gelatin was found to remain in the hydrogel for up to 21 days. The gelatin remaining in the hydrogel had positive effects on encapsulated cells, especially on cell proliferation and osteogenic differentiation. The gelatin released from the hydrogel affected the external cells, showing more favorable osteogenic behavior than the control sample. It was also found that the MA-alginate/gelatin hydrogel could be used as a bioink for printing with high cell viability. Therefore, we anticipate that the alginate-based bioink developed in this study could potentially be used to induce osteogenesis in bone tissue regeneration.
1. Santoni S, Gugliandolo SG, Sponchioni M, et al., 2022, 3D bioprinting: Current status and trends—A guide to the literature and industrial practice. Bio-Design Manuf, 5(1):14–42. https://doi.org/10.1007/s42242-021-00165-0
2. Ahadian S, Khademhosseini A, 2018, A perspective on 3D bioprinting in tissue regeneration. Bio-Design Manuf, 1(3):157–160. https://doi.org/10.1007/s42242-018-0020-3
3. Groll J, Burdick JA, Cho DW, et al., 2018, A definition of bioinks and their distinction from biomaterial inks. Biofabrication, 11(1):013001. https://doi.org/10.1088/1758-5090/aaec52
4. Bian L, 2020, Functional hydrogel bioink, a key challenge of 3D cellular bioprinting. APL Bioeng, 4(3):030401–030401. https://doi.org/10.1063/5.0018548
5. Cui X, Li J, Hartanto Y, et al., 2020, Advances in extrusion 3D bioprinting: A focus on multicomponent hydrogel-based bioinks. Adv Healthc Mater, 9(15):1901648. https://doi.org/10.1002/adhm.201901648
6. Bell JH, Haycock JW, 2012, Next generation nerve guides: Materials, fabrication, growth factors, and cell delivery. Tissue Eng Part B Rev, 18(2):116–128. https://doi.org/10.1089/ten.TEB.2011.0498
7. Kyle S, Jessop ZM, Al-Sabah A, et al., 2017, ‘Printability’ of candidate biomaterials for extrusion based 3D printing: State-of-the-art. Adv Healthc Mater, 6(16):1700264. https://doi.org/10.1002/adhm.201700264
8. Sellaro TL, Ranade A, Faulk DM, et al., 2010, Maintenance of human hepatocyte function in vitro by liver-derived extracellular matrix gels. Tissue Eng Part A, 16(3):1075– 1082. https://doi.org/10.1089/ten.tea.2008.0587
9. Pati F, Jang J, Ha D-H, et al., 2014, Printing three-dimensional tissue analogues with decellularized extracellular matrix bioink. Nat Commun, 5(1):3935. https://doi.org/10.1038/ncomms4935
10. Malda J, Visser J, Melchels FP, et al., 2013, 25th anniversary article: Engineering hydrogels for biofabrication. Adv Mater, 25(36):5011–5028. https://doi.org/10.1002/adma.201302042
11. Ramiah P, du Toit LC, Choonara YE, et al., 2020, Hydrogel-based bioinks for 3D bioprinting in tissue regeneration. Front Mater, 7:76. https://doi.org/10.3389/fmats.2020.00076
12. Fatimi A, Okoro OV, Podstawczyk D, et al., 2022, Natural hydrogel-based bio-inks for 3D bioprinting in tissue engineering: A review. Gels, 8(3):179.
13. Gopinathan J, Noh I, 2018, Recent trends in bioinks for 3D printing. Biomater Res, 22(1):11. https://doi.org/10.1186/s40824-018-0122-1
14. Kim D, Kim M, Lee J, et al., 2022, Review on multicomponent hydrogel bioinks based on natural biomaterials for bioprinting 3D liver tissues. Front Bioeng Biotechnol, 10:764682. https://doi.org/10.3389/fbioe.2022.764682
15. Paxton N, Smolan W, Böck T, et al., 2017, Proposal to assess printability of bioinks for extrusion-based bioprinting and evaluation of rheological properties governing bioprintability. Biofabrication, 9(4):044107. https://doi.org/10.1088/1758-5090/aa8dd8
16. Rastogi P, Kandasubramanian B, 2019, Review of alginate-based hydrogel bioprinting for application in tissue engineering. Biofabrication, 11(4):042001. https://doi.org/10.1088/1758-5090/ab331e
17. Lee KY, Mooney DJ, 2012, Alginate: Properties and biomedical applications. Prog Polym Sci, 37(1):106–126. https://doi.org/10.1016/j.progpolymsci.2011.06.003
18. Sardelli L, Tunesi M, Briatico-Vangosa F, et al., 2021, 3D-reactive printing of engineered alginate inks. Soft Matter, 17(35):8105–8117. https://doi.org/10.1039/D1SM00604E
19. Piras CC, Smith DK, 2020, Multicomponent polysaccharide alginate-based bioinks. J Mater Chem B, 8(36):8171–8188. https://doi.org/10.1039/D0TB01005G
20. Andersen T, Auk-Emblem P, Dornish M, 2015, 3D cell culture in alginate hydrogels. Microarrays (Basel, Switzerland), 4(2):133–161. https://doi.org/10.3390/microarrays4020133
21. Neves MI, Moroni L, Barrias CC, 2020, Modulating alginate hydrogels for improved biological performance as cellular 3D microenvironments. Front Bioeng Biotechnol, 8:665. https://doi.org/10.3389/fbioe.2020.00665
22. Tang-Quan KR, Xi Y, Hochman-Mendez C, et al., 2020, Gelatin promotes cell retention within decellularized heart extracellular matrix vasculature and parenchyma. Cell Mol Bioeng, 13(6):633–645. https://doi.org/10.1007/s12195-020-00634-z
23. Alipal J, Mohd Pu’ad NAS, Lee TC, et al., 2021, A review of gelatin: Properties, sources, process, applications, and commercialisation. Mater Today Proc, 42:240–250. https://doi.org/10.1016/j.matpr.2020.12.922
24. Wu S-C, Chang W-H, Dong G-C, et al., 2011, Cell adhesion and proliferation enhancement by gelatin nanofiber scaffolds. J Bioact Compat Polym, 26(6):565–577. https://doi.org/10.1177/0883911511423563
25. Bello AB, Kim D, Kim D, et al., 2020, Engineering and functionalization of gelatin biomaterials: From cell culture to medical applications. Tissue Eng Part B Rev, 26(2):164–180. https://doi.org/10.1089/ten.TEB.2019.0256
26. Klotz BJ, Gawlitta D, Rosenberg AJWP, et al., 2016, Gelatin-methacryloyl hydrogels: Towards biofabrication-based tissue repair. Trends Biotechnol, 34(5):394–407. https://doi.org/10.1016/j.tibtech.2016.01.002
27. Contessi Negrini N, Celikkin N, Tarsini P, et al., 2020, Three-dimensional printing of chemically crosslinked gelatin hydrogels for adipose tissue engineering. Biofabrication, 12(2):025001. https://doi.org/10.1088/1758-5090/ab56f9
28. Ehrmann A, 2021, Non-toxic crosslinking of electrospun gelatin nanofibers for tissue engineering and biomedicine—A review. Polymers, 13(12):1973.
29. Othman SA, Soon CF, Ma NL, et al., 2021, Alginate-gelatin bioink for bioprinting of hela spheroids in alginate-gelatin hexagon shaped scaffolds. Polym Bull, 78(11):6115–6135. https://doi.org/10.1007/s00289-020-03421-y
30. Gao T, Gillispie GJ, Copus JS, et al., 2018, Optimization of gelatin–alginate composite bioink printability using rheological parameters: A systematic approach. Biofabrication, 10(3):034106. https://doi.org/10.1088/1758-5090/aacdc7
31. Li Z, Huang S, Liu Y, et al., 2018, Tuning alginate-gelatin bioink properties by varying solvent and their impact on stem cell behavior. Sci Rep, 8(1):8020. https://doi.org/10.1038/s41598-018-26407-3
32. Kim AY, Kim Y, Lee SH, et al., 2017, Effect of gelatin on osteogenic cell sheet formation using canine adipose-derived mesenchymal stem cells. Cell Transplant, 26(1):115–123. https://doi.org/10.3727/096368916x693338
33. Hersel U, Dahmen C, Kessler H, 2003, RGD modified polymers: Biomaterials for stimulated cell adhesion and beyond. Biomaterials, 24(24):4385–4415. https://doi.org/10.1016/S0142-9612(03)00343-0
34. Chung JHY, Naficy S, Yue Z, et al., 2013, Bio-ink properties and printability for extrusion printing living cells. Biomater Sci, 1(7):763-773. https://doi.org/10.1039/c3bm00012e
35. Li X, Wang X, Wang X, et al., 2018, 3D bioprinted rat Schwann cell-laden structures with shape flexibility and enhanced nerve growth factor expression. 3 Biotech, 8(8):342. https://doi.org/10.1007/s13205-018-1341-9
36. He Y, Yang F, Zhao H, et al., 2016, Research on the printability of hydrogels in 3D bioprinting. Sci Rep, 6:29977. https://doi.org/10.1038/srep29977
37. Łabowska MB, Cierluk K, Jankowska AM, et al., 2021, A review on the adaption of alginate-gelatin hydrogels for 3D cultures and bioprinting. Materials, 14(4):858. https://doi.org/10.3390/ma14040858
38. Wu S-C, Chang W-H, Dong G-C, et al., 2011, Cell adhesion and proliferation enhancement by gelatin nanofiber scaffolds. J Bioact Compat Polym, 26(6):565–577. https://doi.org/10.1177/0883911511423563
39. Rosellini E, Cristallini C, Barbani N, et al., 2009, Preparation and characterization of alginate/gelatin blend films for cardiac tissue engineering. J Biomed Mater Res Part A, 91A(2):447–453. https://doi.org/10.1002/jbm.a.32216
40. Hoch E, Schuh C, Hirth T, et al., 2012, Stiff gelatin hydrogels can be photo-chemically synthesized from low viscous gelatin solutions using molecularly functionalized gelatin with a high degree of methacrylation. J Mater Sci Mater Med, 23(11):2607–2617. https://doi.org/10.1007/s10856-012-4731-2