Fabrication of large-scale scaffolds with microscale features using light sheet stereolithography
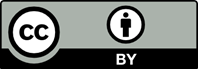
The common characteristics that make scaffolds suitable for human tissue substitutes include high porosity, microscale features, and pores interconnectivity. Too often, however, these characteristics are limiting factors for the scalability of different fabrication approaches, particularly in bioprinting techniques, in which either poor resolution, small areas, or slow processes hinder practical use in certain applications. An excellent example is bioengineered scaffolds for wound dressings, in which microscale pores in large surface-to-volume ratio scaffolds must be manufactured – ideally fast, precise, and cheap, and where conventional printing methods do not readily meet both ends. In this work, we propose an alternative vat photopolymerization technique to fabricate centimeter-scale scaffolds without losing resolution. We used laser beam shaping to first modify the profile of the voxels in 3D printing, resulting in a technology we refer to as light sheet stereolithography (LS-SLA). For proof of concept, we developed a system from commercially available off-the-shelf components to demonstrate strut thicknesses up to 12.8 ± 1.8 μm, tunable pore sizes ranging from 36 μm to 150 μm, and scaffold areas up to 21.4 mm × 20.6 mm printed in a short time. Furthermore, the potential to fabricate more complex and three-dimensional scaffolds was demonstrated with a structure composed of six layers, each rotated by 45° with respect to the previous. Besides the demonstrated high resolution and achievable large scaffold sizes, we found that LS-SLA has great potential for scaling-up of applied oriented technology for tissue engineering applications.
1. O’Brien FJ, 2011, Biomaterials a amp; scaffolds for tissue engineering. Mater Today, 14: 88–95. https://doi.org/10.1016/S1369-7021(11)70058-X
2. Loh QL, Choong C, 2013, Three-dimensional scaffolds for tissue engineering applications: Role of porosity and pore size. Tissue Eng Part B Rev, 19: 485–502. https://doi.org/10.1089/ten.teb.2012.0437
3. O’Brien FJ, Harley BA, Waller MA, et al., 2006, The effect of pore size on permeability and cell attachment in collagen scaffolds for tissue engineering. Technol Heal Care, 15: 3–17. https://doi.org/10.3233/THC-2007-15102
4. Perez RA, Mestres G, 2016, Role of pore size and morphology in musculo-skeletal tissue regeneration. Mater Sci Eng C Mater Biol Appl, 61: 922–939. https://doi.org/10.1016/j.msec.2015.12.087
5. Sobral JM, Caridade SG, Sousa RA, et al., 2011, Three dimensional plotted scaffolds with controlled pore size gradients: Effect of scaffold geometry on mechanical performance and cell seeding efficiency. Acta Biomater, 7: 1009–1018. https://doi.org/10.1016/j.actbio.2010.11.003
6. Sheikholeslam M, Wright ME, Jeschke MG, et al., 2018, Biomaterials for skin substitutes. Adv Healthc Mater, 7: 1700897. https://doi.org/10.1002/adhm.201700897
7. Phelps EA, García AJ, 2010, Engineering more than a cell: Vascularization strategies in tissue engineering. Curr Opin Biotechnol, 21: 704–709. https://doi.org/10.1016/j.copbio.2010.06.005
8. Negut I, Dorcioman G, Grumezescu V, 2020, Scaffolds for wound healing applications. Polymers (Basel), 12: 1–19. https://doi.org/10.3390/polym12092010
9. Gauvin R, Chen YC, Lee JW, et al., 2012, Khademhosseini, Microfabrication of complex porous tissue engineering scaffolds using 3D projection stereolithography. Biomaterials, 33: 3824–3834. https://doi.org/10.1016/j.biomaterials.2012.01.048
10. Park S, Kim G, Jeon YC, et al., 2009, 3D polycaprolactone scaffolds with controlled pore structure using a rapid prototyping system. J Mater Sci Mater Med, 20: 229–234. https://doi.org/10.1007/s10856-008-3573-4
11. Gleadall A, Visscher D, Yang J, et al., 2018, Review of additive manufactured tissue engineering scaffolds: Relationship between geometry and performance. Burn Trauma, 6: 1–16. https://doi.org/10.1186/s41038-018-0121-4
12. Yannas IV, Lee E, Orgill DP, et al., 1989, Synthesis and characterization of a model extracellular matrix that induces partial regeneration of adult mammalian skin. Proc Natl Acad Sci U S A, 86: 933–937. https://doi.org/10.1073/pnas.86.3.933
13. Zhong SP, Zhang YZ, Lim CT, 2010, Tissue scaffolds for skin wound healing and dermal reconstruction. WIREs Nanomed Nanobiotechnol, 2: 510–525. https://doi.org/10.1002/wnan.100
14. Kus KJ, Ruiz ES, 2020, Wound dressings–a practical review. Curr Dermatol Rep, 9: 298–308. https://doi.org/10.1007/s13671-020-00319-w
15. Dabiri G, Damstetter E, Phillips T, 2016, Choosing a wound dressing based on common wound characteristics. Adv Wound Care, 5: 32–41. https://doi.org/10.1089/wound.2014.0586
16. Lindholm C, Searle R, 2016, Wound management for the 21st century: Combining effectiveness and efficiency. Int Wound J, 13: 5–15. https://doi.org/10.1111/iwj.12623
17. Kröger K, Jöster M, 2018, Prevalence of chronic wounds in different modalities of care in Germany. EWMA J, 19: 45–49.
18. Sen CK, 2021, Human wound and its burden: Updated 2020 compendium of estimates. Adv Wound Care, 10: 281–292. https://doi.org/10.1089/wound.2021.0026
19. Posnett J, Gottrup F, Lundgren H, et al., 2009, The resource impact of wounds on health-care providers in Europe. J Wound Care, 18: 154–154. https://doi.org/10.12968/jowc.2009.18.4.41607
20. Powers JG, Higham C, Broussard K, et al., 2016, Wound healing and treating wounds. J Am Acad Dermatol, 74: 607–625. https://doi.org/10.1016/j.jaad.2015.08.070
21. Nichols E, 2015, Wound assessment Part 1: How to measure a wound. Wound Essent, 10: 51–55.
22. Lucas Y, Niri R, Treuillet S, et al., 2021, Castaneda, Wound size imaging: Ready for smart assessment and monitoring. Adv Wound Care, 10: 641–661. https://doi.org/10.1089/wound.2018.0937
23. Verbelen J, Hoeksema H, Heyneman A, et al., 2014, Aquacel® Ag dressing versus Acticoattm dressing in partial thickness burns: A prospective, randomized, controlled study in 100 patients. Part 1: Burn wound healing. Burns, 40: 416–427. https://doi.org/10.1016/j.burns.2013.07.008
24. Selig HF, Lumenta DB, Giretzlehner M, et al., 2012, The properties of an “ideal” burn wound dressing what do we need in daily clinical practice? Results of a worldwide online survey among burn care specialists. Burns, 38: 960–966. https://doi.org/10.1016/j.burns.2012.04.007
25. Yu H, Chen X, Cai J, et al., 2019, Novel porous three dimensional nanofibrous scaffolds for accelerating wound healing. Chem Eng J, 369: 253–262. https://doi.org/10.1016/j.cej.2019.03.091
26. Shyna S, Shanti Krishna A, Nair PD, et al., 2020, A nonadherent chitosan-polyvinyl alcohol absorbent wound dressing prepared via controlled freeze-dry technology. Int J Biol Macromol, 150: 129–140. https://doi.org/10.1016/j.ijbiomac.2020.01.292
27. Boekema B.K.H., Vlig M, Olde Damink L, et al., 2014, Ulrich, effect of pore size and cross-linking of a novel collagen-elastin dermal substitute on wound healing. J Mater Sci Mater Med, 25: 423–433. https://doi.org/10.1007/s10856-013-5075-2
28. Ng S, 2020, Freeze‐dried wafers for wound healing. In: Ther. Dressings Wound Health. Cupertino, Hoboken: Apple, Wiley, pp137–155. https://doi.org/10.1002/9781119433316.ch7
29. Dias JR, Granja PL, Bártolo PJ, 2016, Advances in electrospun skin substitutes. Prog Mater Sci, 84: 314–334. https://doi.org/10.1016/j.pmatsci.2016.09.006
30. Cui T, Yu J, Li Q, et al., 2020, Large‐scale fabrication of robust artificial skins from a biodegradable sealant‐loaded nanofiber scaffold to skin tissue via microfluidic blow spinning. Adv Mater, 32: 2000982. https://doi.org/10.1002/adma.202000982
31. Pereira RF, Barrias CC, Granja PL, et al., 2013, Advanced biofabrication strategies for skin regeneration and repair. Nanomedicine, 8: 603–621. https://doi.org/10.2217/nnm.13.50
32. Chartrain NA, Williams CB, Whittington AR, 2018, A review on fabricating tissue scaffolds using vat photopolymerization. Acta Biomate, 74: 90–111. https://doi.org/10.1016/j.actbio.2018.05.010
33. Mostafavi A, Samandari M, Karvar M, et al., 2021, Colloidal multiscale porous adhesive (bio)inks facilitate scaffold integration. Appl Phys Rev, 8: 041415. https://doi.org/10.1063/5.0062823
34. Jiang T, Munguia-Lopez JG, Flores-Torres S, et al., 2019, Extrusion bioprinting of soft materials: An emerging technique for biological model fabrication Appl Phys Rev, 6: 011310. https://doi.org/10.1063/1.5059393
35. Li X, Liu B, Pei B, et al., 2020, Inkjet Bioprinting of biomaterials. Chem Rev, 120: 10793–10833. https://doi.org/10.1021/acs.chemrev.0c00008
36. Ng WL, Lee JM, Zhou M, et al., 2020, Vat polymerization based bioprinting—process, materials, applications and regulatory challenges. Biofabrication, 12: 022001. https://doi.org/10.1088/1758-5090/ab6034
37. Ge Q, Li Z, Wang Z, et al., 2020, Projection micro stereolithography based 3D printing and its applications. Int J Extrem Manuf, 2: 022004. https://doi.org/10.1088/2631-7990/ab8d9a.
38. Lee MP, Cooper G.J.T, Hinkley T, et al., 2015, Development of a 3D printer using scanning projection stereolithography. Sci Rep, 5: 9875. https://doi.org/10.1038/srep09875
39. Ahn D, Stevens LM, Zhou K, et al., 2020, Rapid high-resolution visible light 3D printing. ACS Cent Sci, 6: 1555–1563. https://doi.org/10.1021/acscentsci.0c00929
40. Sun C, Fang N, Wu DM, et al., 2005, Projection microstereolithography using digital micro-mirror dynamic mask. Sens Actuators A Phys, 121: 113–120. https://doi.org/10.1016/j.sna.2004.12.011
41. Behroodi E, Latifi H, Najafi F, 2019, A compact LEDbased projection microstereolithography for producing 3D microstructures. Sci Rep, 9: 19692. https://doi.org/10.1038/s41598-019-56044-3
42. Liu Y, Nolte DD, Pyrak-Nolte LJ, 2010, Large-format fabrication by two-photon polymerization in SU-8. Appl Phys A, 100: 181–191. https://doi.org/10.1007/s00339-010-5735-8
43. Gong H, Bickham BP, Woolley AT, et al., 2017, Custom 3D printer and resin for 18 μm × 20 μm microfluidic flow channels. Lab Chip, 17: 2899–2909. https://doi.org/10.1039/C7LC00644F
44. Ricci D, Nava M, Zandrini T, et al., 2017, Scaling-Up Techniques for the nanofabrication of cell culture substrates via two-photon polymerization for industrial-scale expansion of stem cells. Materials (Basel), 10: 66. https://doi.org/10.3390/ma10010066
45. Trautmann A, Rüth M, Lemke HD, et al., 2018, Two photon polymerization based large scaffolds for adhesion and proliferation studies of human primary fibroblasts. Opt Laser Technol, 106: 474–480. https://doi.org/10.1016/j.optlastec.2018.05.008
46. Weisgrab G, Guillaume O, Guo Z, et al., 2020, Ovsianikov, 3D printing of large-scale and highly porous biodegradable tissue engineering scaffolds from poly(trimethylenecarbonate) using two-photon-polymerization. Biofabrication, 12: 045036. https://doi.org/10.1088/1758-5090/abb539
47. Stender B, Hilbert F, Dupuis Y, et al., 2019, Manufacturing strategies for scalable high-precision 3D printing of structures from the micro to the macro range. Adv Opt Technol, 8: 225–231. https://doi.org/10.1515/aot-2019-0022
48. Zhu W, Tringale KR, Woller SA, et al., 2018, Rapid continuous 3D printing of customizable peripheral nerve guidance conduits. Mater Today, 21: 951–959. https://doi.org/10.1016/j.mattod.2018.04.001
49. Ren M, Lu W, Shao Q, et al., 2021, Aberration-free large-area stitch-free 3D nano-printing based on binary holography. Opt Express, 29: 44250. https://doi.org/10.1364/OE.446503
50. What are Cylinder Lenses? Barrington: Edmund Optics, (n.d.), Available from: https://www.edmundoptics.com/knowledgecenter/ application-notes/optics/what-are-cylinder-lenses [Last accessed on 2022 Jul 13].
51. Goodman JW, 1996, Introduction to Fourier Optics. 2nd ed. New York: McGraw-Hill.
52. Jacobs PF, 1992, Rapid Prototyping and Manufacturing. 1st ed. Southfield: Society of Manufacturing Engineers.
53. Sigma-Aldrich.Com, 3-(Trimethoxysilyl)propyl methacrylate Product Information, (n.d.), 6514. Available from: https://www.sigmaaldrich.com/deepweb/assets/sigmaaldrich/ product/documents/139/774/m6514pis.pdf [Last accessed on 2022 May 19].
54. Wang Z, Abdulla R, Parker B, et al., 2015, A simple and high resolution stereolithography-based 3D bioprinting system using visible light crosslinkable bioinks. Biofabrication, 7: 045009. https://doi.org/10.1088/1758-5090/7/4/045009
55. Zhang R, Larsen NB, 2017, Stereolithographic hydrogel printing of 3D culture chips with biofunctionalized complex 3D perfusion networks. Lab Chip, 17: 4273–4282. https://doi.org/10.1039/c7lc00926g
56. Texas Instruments, (n.d.), DLP Getting Started. DLP Products. Dallas: TI.com. Available from: https://www.ti.com/dlp-chip/getting-started.html#choose_DLP [Last accessed on 2022 Mar 16].
57. Texas Instruments, (n.d.), UV Products (< 400 nm). Dallas: TI.com. Available from: https://www.ti.com/dlp-chip/3dprinting- direct-imaging/ultraviolet/overview.html [Last accessed on 2022 Jun 29].
58. Special Optics. Scanning Lens Theory. New Jersey: Special Optics. Available from: https://www.specialoptics.com/support/resources [Last accessed on 2022 Jul 13].
59. Bumstead JR, Park JJ, Rosen IA, et al., 2018, Designing a large field-of-view two-photon microscope using optical invariant analysis. Neurophotonics, 5: 025001. https://doi.org/10.1117/1.nph.5.2.025001
60. Optics S, Production of Optical Components of Highest Quality-ƒ-theta Lenses. Available from: https://www.silloptics.de/en/products/laser-optics/f-theta-lenses-1 [Last accessed on 2022 Jul 13].
61. Optics S, (n.d.), Stock F-Theta Lenses. Available from: https://www.shanghai-optics.com/stock-optics/stock-f-theta-lenses [Last accessed on 2022 Jul 13].
62. Mondschein RJ, Kanitkar A, Williams CB, et al., 2017, Polymer structure-property requirements for stereolithographic 3D printing of soft tissue engineering scaffolds. Biomaterials, 140: 170–188. https://doi.org/10.1016/j.biomaterials.2017.06.005