A Study on Dual-Response Composite Hydrogels Based on Oriented Nanocellulose
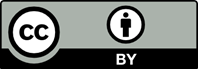
In nature, many biological tissues are composed of oriented structures, which endow tissues with special properties and functions. Although traditional hydrogels can achieve a high level of biomimetic composition, the orderly arrangement of internal structures remains a challenge. Therefore, it is of great significance to synthesize hydrogels with oriented structures easily and quickly. In this study, we first proposed and demonstrated a fabrication process for producing a well-ordered and dual-responsive cellulose nanofibers + hyaluronic acid methacrylate (CN+HAMA) hydrogels through an extrusion-based three-dimensional (3D) printing process. CN in the CN+HAMA hydrogels are directionally aligned after extrusion due to shear stress. In addition, the synthesized hydrogels exhibited responsive behaviors to both temperature and ultraviolet light. Since the temperature-responsiveness is reversible, the hydrogels can transit between the gelation and solution states while retaining their original qualities. Furthermore, the developed well-oriented CN+HAMA hydrogels induced directional cell growth, paving the way for potential applications in ordered biological soft-tissue repair
1. Powers DB, Breeze J, 2019, Avulsive Soft Tissue Injuries. Atlas Oral Maxillofac Surg Clin, 27:135–42. https://doi.org/10.1016/j.cxom.2019.05.005
2. Brown BN, Badylak SF, 2014, Extracellular Matrix as an Inductive Scaffold for Functional Tissue Reconstruction. Transl Res, 163:268–85. https://doi.org/10.1016/j.trsl.2013.11.003
3. Lee KY, Mooney DJ, 2001, Hydrogels for Tissue Engineering. Chem Rev, 101:1869–79.
4. Zhang YS, Khademhosseini A, 2017, Advances in Engineering Hydrogels. Science, 356:eaaf3627.
5. Hoffman AS, 2012, Hydrogels for Biomedical Applications. Adv Drug Deliver Rev, 64:18–23.
6. Tavakolian M, Jafari SM, van de Ven TG, 2020, A Review on Surface-Functionalized Cellulosic Nanostructures as Biocompatible Antibacterial Materials. Nano-Micro Lett, 12:73. https://doi.org/10.1007/s40820-020-0408-4
7. Hai Van H, Makkar P, Padalhin AR, et al., 2020, Preliminary Studies on the in Vivo Performance of Various Kinds of Nanocellulose for Biomedical Applications. J Biomater Appl, 34:942–51. https://doi.org/10.1177/0885328219883478
8. De France KJ, Hoare Tand Cranston E D, 2017, Review of Hydrogels and Aerogels Containing Nanocellulose. Chem Mater, 29:4609–31. https://doi.org/10.1021/acs.chemmater.7b00531
9. Ravanbakhsh H, Bao G, Luo Z, et al., 2021, Composite Inks for Extrusion Printing of Biological and Biomedical Constructs. ACS Biomater Sci Eng, 7:4009–26. https://doi.org/10.1021/acsbiomaterials.0c01158
10. Tashiro K, Kobayashi M, 1991, Theoretical Evaluation of 3-Dimensional Elastic-constants of Native and Regenerated Celluloses-role of Hydrogen-bonds. Polymer, 32:1516–30. https://doi.org/10.1016/0032-3861(91)90435-l
11. Mueller M, Ozturk E, Arlov O, et al., 2017, Alginate Sulfate-Nanocellulose Bioinks for Cartilage Bioprinting Applications. Ann Biomed Eng, 45:210–23. https://doi.org/10.1007/s10439-016-1704-5
12. Chinga-Carrasco G, 2018, Potential and Limitations of Nanocelluloses as Components in Biocomposite Inks for Three-Dimensional Bioprinting and for Biomedical Devices. Biomacromolecules, 19:701–11. https://doi.org/10.1021/acs.biomac.8b00053
13. Yadav S, Majumder A, 2021, Biomimicked Hierarchical 2D and 3D Structures from Natural Templates: Applications in Cell Biology. Biomed Mater, 16:7. https://doi.org/10.1088/1748-605x/ac21a7
14. Hua M, Wu S, Ma Y, et al., 2021, Strong Tough Hydrogels Via the Synergy of Freeze-casting and Salting Out. Nature, 590:594–9. https://doi.org/10.1038/s41586-021-03212-z
15. Zhang H, Cooper AI, 2007, Aligned Porous Structures by Directional Freezing. Adv Mater, 19:1529–33. https://doi.org/10.1002/adma.200700154
16. Liu M, Ishida Y, Ebina Y, et al., 2015, An Anisotropic Hydrogel with Electrostatic Repulsion between Cofacially Aligned Nanosheets. Nature, 517:68–72. https://doi.org/10.1038/nature14060
17. Kuo JC, Huang HW, Tung SW, et al., 2014, A Hydrogel-based Intravascular Microgripper Manipulated using Magnetic Fields. Sensor Actuat Phys, 211:121–30. https://doi.org/10.1016/j.sna.2014.02.028
18. Shikinaka K, Koizumi Y, Kaneda K, et al., 2013, Straininduced Reversible Isotropic-anisotropic Structural Transition of Imogolite Hydrogels. Polymer, 54:2489–92. https://doi.org/10.1016/j.polymer.2013.03.028
19. Shao JJ, Raidongia K, Koltonow AR, et al., 2015, Self-assembled Two-dimensional Nanofluidic Proton Channels with High Thermal Stability. Nat Commun, 6:7602–2. https://doi.org/10.1038/ncomms8602
20. Guo J, Li Q, Zhang R, et al., 2022, Loose Pre-cross-linking Mediating Cellulose Self-assembly for 3D Printing Strong and Tough Biomimetic Scaffolds. Biomacromolecules, 23:877-88. https://doi.org/10.1021/acs.biomac.1c01330.s002
21. Ye D, Yang P, Lei X, et al., 2018, Robust Anisotropic Cellulose Hydrogels Fabricated via Strong Self-aggregation Forces for Cardiomyocytes Unidirectional Growth. Chem Mater, 30:5175–83. https://doi.org/10.1021/acs.chemmater.8b01799
22. Zou J, Wu S, Chen J, et al., 2019, Highly Efficient and Environmentally Friendly Fabrication of Robust, Programmable, and Biocompatible Anisotropic, All-Cellulose, Wrinkle-Patterned Hydrogels for Cell Alignment. Adv Mater, 31:1904762. https://doi.org/10.1002/adma.201904762
23. Kong W, Wang C, Jia C, et al., 2021, Muscle-inspired Highly Anisotropic, Strong, Ion-Conductive Hydrogels. Adv Mater, 33:1801934–41. https://doi.org/10.1002/adma.201801934
24. Ma T, Lv L, Ouyang C, et al., 2021, Rheological Behavior and Particle Alignment of Cellulose Nanocrystal and its Composite Hydrogels During 3D Printing. Carbohyd Polym, 253:117217. https://doi.org/10.1016/j.carbpol.2020.117217
25. Fourmann O, Hausmann MK, Neels A, et al., 2021, 3D Printing of Shape-morphing and Antibacterial Anisotropic Nanocellulose Hydrogels. Carbohydr Polym, 259:117716–27. https://doi.org/10.1016/j.carbpol.2021.117716
26. Collins MN, Birkinshaw C, 2013, Hyaluronic Acid Based scaffolds for Tissue Engineering a Review. Carbohyd Polym, 92:1262–79. https://doi.org/10.1016/j.carbpol.2012.10.028
27. Gwak MA, Hong BM, Seok JM, et al., 2021, Effect of Tannic Acid on the Mechanical and Adhesive Properties of Catechol modified Hyaluronic Acid Hydrogels. Int J Biol Macromol, 191:699–705. https://doi.org/10.1016/j.ijbiomac.2021.09.123
28. Shin J, Lee JS, Lee C, et al., 2015, Tissue Adhesive Catechol-Modified Hyaluronic Acid Hydrogel for Effective, Minimally Invasive Cell Therapy. Adv Funct Mater, 25:3814–24. https://doi.org/10.1002/adfm.201500006
29. Xavier Acasigua GA, de Olyveira GM, Manzine Costa LM, et al., 2014, Novel Chemically Modified Bacterial Cellulose Nanocomposite as Potential Biomaterial for Stem Cell Therapy Applications. Curr Stem Cell Res T, 9:117–23. https://doi.org/10.2174/1574888x08666131124135654
30. Fan Y, Yue Z, Lucarelli E, et al., 2020, Hybrid Printing Using Cellulose Nanocrystals Reinforced GelMA/HAMA Hydrogels for Improved Structural Integration. Adv Healthc Mater, 9:2001410. https://doi.org/10.1002/adhm.202001410
31. Zhao H, Zhang Y, Liu Y, et al., 2021, In Situ Forming Cellulose Nanofibril-Reinforced Hyaluronic Acid Hydrogel for Cartilage Regeneration. Biomacromolecules, 22:5097–107. https://doi.org/10.1021/acs.biomac.1c01063
32. Sun W, Starly B, Daly AC, et al., 2020, The Bioprinting Roadmap. Biofabrication, 12:022002. https://doi.org/10.1088/1758-5090/ab5158
33. Arab W, Kahin K, Khan Z, et al., 2019, Exploring Nanofibrous Self-assembling Peptide Hydrogels using Mouse Myoblast Cells for Three-dimensional Bioprinting and Tissue Engineering Applications. Int J Bioprinting, 5:74–82. https://doi.org/10.18063/ijb.v5i2.198
34. Lin C, Wang Y, Huang Z, et al., 2021, Advances in Filament Structure of 3D Bioprinted Biodegradable Bone Repair Scaffolds. Int J Bioprinting, 7:43–64. https://doi.org/10.18063/ijb.v7i4.426
35. Zhang X, Liu Y, Zuo Q, et al., 2021, 3D Bioprinting of Biomimetic Bilayered Scaffold Consisting of Decellularized Extracellular Matrix and Silk Fibroin for Osteochondral Repair. Int J Bioprinting, 7:85–98.
36. Zheng C, Attarilar S, Li K, et al., 2021, 3D-printed HA15-loaded Beta-Tricalcium Phosphate/Poly (Lactic-co-glycolic acid) Bone Tissue Scaffold Promotes Bone Regeneration in Rabbit Radial Defects. Int J Bioprinting, 7:100–11. https://doi.org/10.18063/ijb.v7i1.317
37. Guo Z, Mi S, Sun W, 2019, A Facile Strategy for Preparing Tough, Self-Healing Double-Network Hyaluronic Acid Hydrogels Inspired by Mussel Cuticles. Macromol Mater Eng, 304(4):1800715–22. https://doi.org/10.1002/mame.201800715
38. Li Y, Yu P, Wen J, et al., 2022, Nanozyme-based Stretchable Hydrogel of Low Hysteresis with Antibacterial and Antioxidant Dual Functions for Closely Fitting and Wound Healing in Movable Parts. Adv Funct Mater, 32:2110720–33. https://doi.org/10.1002/adfm.202110720
39. Zhang J, Wu J, Yu J, et al., 2017, Application of Ionic Liquids for Dissolving Cellulose and Fabricating Cellulose-based Materials: State of the Art and Future Trends. Mater Chem Front, 1:1273–90. https://doi.org/10.1039/c6qm00348f
40. Mouser VH, Abbadessa A, Levato R, et al., 2017, Development of a Thermosensitive HAMA-containing Bio-ink for the Fabrication of Composite Cartilage Repair Constructs. Biofabrication, 9:015026. https://doi.org/10.1088/1758-5090/aa6265
41. Siqueira G, Kokkinis D, Libanori R, et al., 2017, Cellulose Nanocrystal Inks for 3D Printing of Textured Cellular Architectures. Adv Funct Mater, 27:6926–37. https://doi.org/10.1002/adfm.201604619
42. Hoo SP, Sarvi F, Li WH, et al., 2013, Thermo responsive Cellulosic Hydrogels with Cell-Releasing Behavior. ACS Appl Mater Inter, 5:5592–600. https://doi.org/10.1021/am4009133
43. Zhang Y, Gao C, Li X, et al., 2014, Thermosensitive Methyl Cellulose-based Injectable Hydrogels for Post-operation Anti-adhesion. Carbohydr Polym, 101:171–8. https://doi.org/10.1016/j.carbpol.2013.09.001
44. Omidinia-Anarkoli A, Boesveld S, Tuvshindorj U, et al., 2017, An Injectable Hybrid Hydrogel with Oriented Short Fibers Induces Unidirectional Growth of Functional Nerve Cells. Small, 13:70191. https://doi.org/10.1002/smll.201770191
45. Kim J, Bae WG, Kim YJ, et al., 2017, Directional Matrix Nanotopography with Varied Sizes for Engineering Wound Healing. Adv Healthc Mater, 6:1700297. https://doi.org/10.1002/adhm.201700297
46. Zhou F, Yuan L, Mei Y, et al., 2011, Effects of contact guidance and gravity on L929 cell orientation. Chinese Sci Bull, 56:977–81. https://doi.org/10.1007/s11434-010-4173-5