A Codispersed Nanosystem of Silver-anchored MoS2 Enhances Antibacterial and Antitumor Properties of Selective Laser Sintered Scaffolds
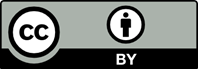
Tumor recurrence and bacterial infection are common problems during bone repair and reconstruction after bone tumor surgery. In this study, silver-anchored MoS2 nanosheets (Ag@PMoS2 ) were synthesized by in situ reduction, then a composite polymer scaffold (Ag@PMoS2 /PGA) with sustained antitumor and antibacterial activity was successfully constructed by selective laser sintering technique. In the Ag@PMoS2 nanostructures, silver nanoparticles (Ag NPs) were sandwiched between adjacent MoS2 nanosheets (MoS2 NSs), which restrained the restacking of the MoS2 NSs. In addition, the MoS2 NSs acted as steric hindrance layers, which prevented the aggregation of Ag NPs. More importantly, MoS2 NSs can provide a barrier layer for Ag NPs, hindering Ag NPs from reacting with the external solution to prevent its quick release. The results showed that Ag@PMoS2 /PGA scaffolds have stronger photothermal effect and antitumor function. Meanwhile, the Ag@PMoS2 /PGA scaffolds also demonstrated slow control of silver ion (Ag+ ) release and more efficient long-term antibacterial ability. Besides, composite scaffolds have been proved to kill the MG-63 cells by inducing apoptosis and inhibit bacterial proliferation by upregulating the level of bacterial reactive oxygen species. This kind of novel bifunctional implants with antitumor and antibacterial properties provides better choice for the artificial bone transplantation after primary bone tumor resection.
1. Yang Q, Yin H, Xu T, et al., 2020, Engineering 2D Mesoporous Silica@MXene-Integrated 3D-Printing Scaffolds for Combinatory Osteosarcoma Therapy and NO-Augmented Bone Regeneration. Small, 16:e1906814. https://doi.org/10.1002/smll.201906814
2. Smeland S, Bielack SS, Whelan J, et al., 2019, Survival and Prognosis with Osteosarcoma: Outcomes in more than 2000 Patients in the EURAMOS-1 (European and American Osteosarcoma Study) cohort. Eur J Cancer, 109:36–50. https://doi.org/10.1016/j.ejca.2018.11.027
3. Dang W, Li T, Li B, et al., 2018, A Bifunctional Scaffold with CuFeSe2 Nanocrystals for Tumor Therapy and Bone Reconstruction. Biomaterials, 160:92–106. https://doi.org/10.1016/j.biomaterials.2017.11.020
4. Feng P, Jia J, Peng S, et al., 2022, Transcrystalline Growth of PLLA on Carbon Fiber Grafted with Nano-SiO2 Towards Boosting Interfacial Bonding in Bone Scaffold. Biomater Res, 26:2. https://doi.org/10.1186/s40824-021-00248-0
5. Zan J, Qian G, Deng F, et al., 2022, Dilemma and Breakthrough of Biodegradable Poly-l-lactic Acid in Bone Tissue Repair. J Mater Res Technol, 2369–87. https://doi.org/10.1016/j.jmrt.2022.01.164
6. Li Y, Yang Y, Huang Z, et al., 2020, Bone Defect Reconstruction with Autologous Bone Inactivated with Liquid Nitrogen after Resection of Primary Limb Malignant Tumors: An Observational Study. Medicine (Baltimore), 99:e20442. https://doi.org/10.1097/MD.0000000000020442
7. Zhu C, He M, Sun D, et al., 2021, 3D-Printed Multifunctional Polyetheretherketone Bone Scaffold for Multimodal Treatment of Osteosarcoma and Osteomyelitis. ACS Appl Mater Interfaces, 13:47327–40. https://doi.org/10.1021/acsami.1c10898
8. Li K, Cai K, Ran Q, et al., 2019, Biomimetic Triphase Composite Scaffolds with Antibacterial and Anti-Tumor Potentials for Bone Repair. Mater Lett, 256:126590. https://doi.org/10.1016/j.matlet.2019.126590
9. Wang L, Yang Q, Huo M, et al., 2021, Engineering Single-Atomic Iron-Catalyst-Integrated 3D-Printed Bioscaffolds for Osteosarcoma Destruction with Antibacterial and Bone Defect Regeneration Bioactivity. Adv Mater, 33:e2100150. https://doi.org/10.1002/adma.202100150
10. He M, Zhu C, Xu H, et al., 2020, Conducting Polyetheretherketone Nanocomposites with an Electrophoretically Deposited Bioactive Coating for Bone Tissue Regeneration and Multimodal Therapeutic Applications. ACS Appl Mater Interfaces, 12(51): 56924-56934. https://doi.org/10.1021/acsami.0c20145
11. Chen SC, Lin CY, Cheng TL, et al., 2017, 6‐Mercaptopurine‐Induced Fluorescence Quenching of Monolayer MoS2 Nanodots: Applications to Glutathione Sensing, Cellular Imaging, and Glutathione‐Stimulated Drug Delivery. Adv Funct Mater, 27:1702452. https://doi.org/10.1002/adfm.201702452
12. Gao Q, Zhang X, Yin W, et al., 2018, Functionalized MoS2 Nanovehicle with Near-Infrared Laser-Mediated Nitric Oxide Release and Photothermal Activities for Advanced Bacteria-Infected Wound Therapy. Small, 14:e1802290. https://doi.org/10.1002/smll.201802290
13. Yu J, Yin W, Zheng X, et al., 2015, Smart MoS2/Fe3O4 Nanotheranostic for Magnetically Targeted Photothermal Therapy Guided by Magnetic Resonance/Photoacoustic Imaging. Theranostics, 5:931–45. https://doi.org/10.7150/thno.11802
14. Cheng L, Wang X, Gong F, et al., 2020, 2D Nanomaterials for Cancer Theranostic Applications. Adv Mater, 32:e1902333. https://doi.org/10.1002/adma.201902333
15. Cai S, Yan J, Xiong H, et al., 2020, Aptamer-Functionalized Molybdenum Disulfide Nanosheets for Tumor Cell Targeting and Lysosomal Acidic Environment/NIR Laser Responsive Drug Delivery to Realize Synergetic Chemo-Photothermal Therapeutic Effects. Int J Pharm, 590:119948. https://doi.org/10.1016/j.ijpharm.2020.119948
16. Fu S, Zhang Y, Qin G, et al., 2021, Antibacterial Effect of TiAg Alloy Motivated by Ag-Containing Phases. Mater Sci Eng C Mater Biol Appl, 128:112266. https://doi.org/10.1016/j.msec.2021.112266
17. Liu A, Wang CZ, Chu C, et al., 2018, Adsorption Performance toward Organic Pollutants, Odour Control and Anti-Microbial Activities of One Ag-Based Coordination Polymer. 6:4961–9. https://doi.org/10.1016/j.jece.2018.07.035
18. Gao C, Yao M, Peng S, et al., 2021, Pre-Oxidation Induced In Situ Interface Strengthening in Biodegradable Zn/nano SiC Composites Prepared by Selective Laser Melting. J Adv Res, 38:143–55. https://doi.org/10.1016/j.jare.2021.09.014
19. Feng P, Kong Y, Liu M, et al., 2021, Dispersion Strategies for Low-Dimensional Nanomaterials and Their Application in Biopolymer Implants. 100127. Mater Today, 2021:100127. https://doi.org/10.1016/j.mtnano.2021.100127
20. Shuai C, Guo W, Wu P, et al., 2018, A Graphene Oxide-Ag Co-Dispersing Nanosystem: Dual Synergistic Effects on Antibacterial Activities and Mechanical Properties of Polymer Scaffolds. Chem Eng J, 347:322–33. https://doi.org/10.1016/j.cej.2018.04.092
21. Yang M, Shuai Y, Yang Y, et al., 2022, In situ Grown Rare Earth Lanthanum on Carbon Nanofibre for Interfacial Reinforcement in Zn Implants. Virtual Phys Prototyp, 2022:1-18. https://doi.org/10.1080/17452759.2022.2053929
22. Ali I, Hussain R, Louis H, et al., 2021, In situ Reduced Graphene-Based Aerogels Embedded with Gold Nanoparticles for Real-Time Humidity Sensing And Toxic Dyes Elimination. Mikrochim Acta, 188:10. https://doi.org/10.1007/s00604-020-04658-0
23. Xu P, Liang J, Cao X, et al., 2016, Facile Synthesis of Monodisperse of Hollow Mesoporous SiO2 Nanoparticles and In-Situ Growth of Ag Nanoparticles for Antibacterial. J Colloid Interface Sci, 474:114–8. https://doi.org/10.1016/j.jcis.2016.04.009
24. Qi F, Zeng Z, Yao J, et al., 2021, Constructing Core-Shell Structured BaTiO3@ Carbon Boosts Piezoelectric Activity and Cell Response of Polymer Scaffolds. Mater Sci Eng C, 126:112129. https://doi.org/10.1016/j.msec.2021.112129
25. Hu Y, Dan W, Xiong S, et al., 2017, Development of Collagen/Polydopamine Complexed Matrix as Mechanically Enhanced and Highly Biocompatible Semi-Natural Tissue Engineering Scaffold. Acta Biomater, 47:135–48. https://doi.org/10.1016/j.actbio.2016.10.017
26. Chen T, Liu Z, Zhang K, et al., 2021, Mussel-Inspired Ag NPs Immobilized on Melamine Sponge for Reduction of 4-Nitrophenol, Antibacterial Applications and Its Superhydrophobic Derivative for Oil-Water Separation. ACS Appl Mater Interfaces, 13:50539–51. https://doi.org/10.1021/acsami.1c14544
27. Yang Y, Yang M, He C, et al., 2021, Rare Earth Improves Strength and Creep Resistance of Additively Manufactured Zn Implants. Compos Part B Eng, 216:108882. https://doi.org/10.1016/j.compositesb.2021.108882
28. Yang Y, Lu C, Shen L, et al., 2021, In-Situ Deposition of Apatite Layer to Protect Mg-Based Composite Fabricated Via Laser Additive Manufacturing. J Magn Alloys, in press. https://doi.org/10.1016/j.jma.2021.04.009
29. Qian G, Zhang L, Wang G, et al., 2021, 3D Printed Zn-doped Mesoporous Silica-incorporated Poly-L-lactic Acid Scaffolds for Bone Repair. Int J Bioprint, 7:346. https://doi.org/10.18063/ijb.v7i2.346
30. Feng P, Wu P, Gao C, et al., 2018, A Multimaterial Scaffold with Tunable Properties: Toward Bone Tissue Repair. Adv Sci (Weinh), 5:1700817. https://doi.org/10.1002/advs.201700817
31. Qi F, Liao R, Shuai Y, et al., 2022, A Conductive Network Enhances Nerve Cell Response. Addit Manuf, 52:102694. https://doi.org/10.1016/j.addma.2022.102694
32. Qi F, Gao X, Shuai Y, et al., 2022, Magnetic-Driven Wireless Electrical Stimulation in a Scaffold. Compos Part B Eng, 237:109864. https://doi.org/10.1016/j.compositesb.2022.109864
33. Wang H, Zeng X, Pang L, et al., 2020, Integrative Treatment of Anti-Tumor/Bone Repair by Combination of MoS2 Nanosheets with 3D Printed Bioactive Borosilicate Glass Scaffolds. Nanoscale, 396:125081. https://doi.org/10.1016/j.cej.2020.125081
34. Zhang Z, Zhang J, Zhang B, et al., 2013, Mussel-Inspired Functionalization of Graphene for Synthesizing Ag-Polydopamine-Graphene Nanosheets as Antibacterial Materials. Nanoscale, 5:118–23. https://doi.org/10.1039/c2nr32092d
35. Yuwen L, Sun Y, Tan G, et al., 2018, MoS2@polydopamine-Ag Nanosheets with Enhanced Antibacterial Activity for Effective Treatment of Staphylococcus aureus Biofilms and Wound Infection. Nanoscale, 10:16711–20. https://doi.org/10.1039/c8nr04111c
36. Xie Y, Yan B, Xu H, et al., 2014, Highly Regenerable Mussel-Inspired Fe(3)O(4)@polydopamine- Ag Core-Shell Microspheres as Catalyst and Adsorbent for Methylene Blue Removal. ACS Appl Mater Interfaces, 6:8845–52. https://doi.org/10.1021/am501632f
37. Shuai C, Xu Y, Feng P, et al., 2019, Antibacterial Polymer Scaffold Based on Mesoporous Bioactive Glass Loaded with In Situ Grown Silver. Chem Eng J, 374:304–15. https://doi.org/10.1016/j.cej.2019.03.273
38. Shuai C, Liu G, Yang Y, et al., 2020, A Strawberry-Like Ag-Decorated Barium Titanate Enhances Piezoelectric and Antibacterial Activities of Polymer Scaffold. Nano Energy, 74:104825. https://doi.org/10.1016/j.nanoen.2020.104825
39. Zeng G, Huang L, Huang Q, et al., 2018, Rapid Synthesis of MoS2-PDA-Ag Nanocomposites as Heterogeneous Catalysts and Antimicrobial Agents via Microwave Irradiation. Appl Surf Sci, 459:588–95. https://doi.org/10.1016/j.apsusc.2018.07.144
40. Loh QL, Choong C, 2013, Three-Dimensional Scaffolds for Tissue Engineering Applications: Role of Porosity and Pore Size. Tissue Eng Part B Rev, 19:485–502. https://doi.org/10.1089/ten.TEB.2012.0437
41. Wang C, Shuai Y, Yang Y, et al., 2022, Amorphous Magnesium Alloy with High Corrosion Resistance Fabricated by Laser Powder Bed Fusion. J Alloys Compd, 897:163247. https://doi.org/10.1016/j.jallcom.2021.163247
42. Yang Y, Cheng Y, Yang M, et al., 2022, Semi coherent Strengthens Graphene/Zinc Scaffolds. Mater Today Nano, 17:100163. https://doi.org/10.1016/j.mtnano.2021.100163
43. Shuai C, Yu L, Feng P, et al., 2022, Construction of a Stereocomplex between Poly(D-lactide) Grafted Hydroxyapatite and Poly(L-lactide): Toward a Bioactive Composite Scaffold with Enhanced Interfacial Bonding. J Mater Chem B, 10:214–23. https://doi.org/10.1039/d1tb02111g
44. Zeng J, Xu L, Luo X, et al., 2021, A Novel Design of SiH/CeO2(111) Van Der Waals Type-II Heterojunction for Water Splitting. Phys Chem Chem Phys, 23:2812–8. https://doi.org/10.1039/d0cp05238h
45. Feng P, Kong Y, Yu L, et al., 2019, Molybdenum Disulfide Nanosheets Embedded with Nanodiamond Particles: Co-Dispersion Nanostructures as Reinforcements for Polymer Scaffolds. Appl Mater Today, 17:216–26. https://doi.org/10.1016/j.apmt.2019.08.005
46. Wu S, Butt HJ, 2016, Near-Infrared-Sensitive Materials Based on Upconverting Nanoparticles. Adv Mater, 28:1208–26. https://doi.org/10.1002/adma.201502843
47. Tian B, Wang C, Zhang S, et al., 2011, Photothermally Enhanced Photodynamic Therapy Delivered by Nano-Graphene Oxide. ACS Nano, 5:7000–9. https://doi.org/10.1021/nn201560b
48. Rengan AK, Bukhari AB, Pradhan A, et al., 2015, In Vivo Analysis of Biodegradable Liposome Gold Nanoparticles as Efficient Agents for Photothermal Therapy of Cancer. Nano Lett, 15:842–8. https://doi.org/10.1021/nl5045378
49. Tong L, Zhao Y, Huff TB, et al., 2007, Gold Nanorods Mediate Tumor Cell Death by Compromising Membrane Integrity. Adv Mater, 19:3136–41. https://doi.org/10.1002/adma.200701974
50. Xing Y, Kang T, Luo X, et al., 2019, Coral-shaped Au Nanostructures for Selective Apoptosis Induction during Photothermal Therapy. J Mater Chem B, 7:6224–6231. https://doi.org/10.1039/c9tb01503e
51. D’Arcy MS, 2019, Cell Death: A Review of the Major Forms of Apoptosis, Necrosis and Autophagy. Cell Biol Int, 43:582–92. https://doi.org/10.1002/cbin.11137
52. Ndoye A, Weeraratna AT, 2016, Autophagy an emerging Target for Melanoma Therapy. F1000Res, 5:F1000 Faculty Rev-1888. https://doi.org/10.12688/f1000research.8347.1
53. Pan L, Liu J, Shi J, 2017, Nuclear-Targeting Gold Nanorods for Extremely Low NIR Activated Photothermal Therapy. ACS Appl Mater Interfaces, 9:15952–61. https://doi.org/10.1021/acsami.7b03017
54. Melamed JR, Edelstein RS, Day ES, 2015, Elucidating the Fundamental Mechanisms of Cell Death Triggered by Photothermal Therapy. ACS Nano, 9:6–11. https://doi.org/10.1021/acsnano.5b00021
55. Aioub M, El-Sayed MA, 2016, A Real-Time Surface Enhanced Raman Spectroscopy Study of Plasmonic Photothermal Cell Death Using Targeted Gold Nanoparticles. J Am Chem Soc, 138:1258–64. https://doi.org/10.1021/jacs.5b10997
56. Banin E, Hughes D, Kuipers OP, 2017, Editorial: Bacterial Pathogens, Antibiotics and Antibiotic Resistance. FEMS Microbiol Rev, 41:450–2. https://doi.org/10.1093/femsre/fux016
57. Yu L, He T, Yao J, et al., 2022, Cu Ions and Cetyltrimethylammonium Bromide Loaded Into Montmorillonite: A Synergistic Antibacterial System for Bone Scaffolds. Mater Chem Front, 6:103–16. https://doi.org/10.1039/D1QM01278A
58. Liu J, He K, Wu W, et al., 2017, In Situ Synthesis of Highly Dispersed and Ultrafine Metal Nanoparticles from Chalcogels. J Am Chem Soc, 139:2900–3. https://doi.org/10.1021/jacs.6b13279
59. Li YT, Lin SB, Chen LC, et al., 2017, Antimicrobial Activity and Controlled Release of Nanosilvers in Bacterial Cellulose Composites Films Incorporated with Montmorillonites. Cellulose, 24:4871–83.
https://doi.org/10.1007/s10570-017-1487-3
60. Qi F, Wang Z, Shuai Y, et al., 2022, Sr2+ Sustained Release System Augments Bioactivity of Polymer Scaffold. ACS Appl Polym Mater, 4:2691–702. https://doi.org/10.1021/acsapm.2c00024
61. Deng F, Wu P, Qian G, et al., 2022, Silver-Decorated Black Phosphorus: A Synergistic Antibacterial Strategy. Nanotechnology, 33(24): 245708 https://doi.org/10.1088/1361-6528/ac5aee
62. Sukhorukova IV, Sheveyko AN, Shvindina NV, et al., 2017, Approaches for Controlled Ag (+) Ion Release: Influence of Surface Topography, Roughness, and Bactericide Content. ACS Appl Mater Interfaces, 9:4259–71. https://doi.org/10.1021/acsami.6b15096
63. Su HL, Chou CC, Hung DJ, et al., 2009, The Disruption of Bacterial Membrane Integrity Through ROS Generation Induced by Nanohybrids of Silver and Clay. Biomaterials, 30:5979–87. https://doi.org/10.1016/j.biomaterials.2009.07.030