Air-loaded Gas Vesicle Nanoparticles Promote Cell Growth in Three-dimensional Bioprinted Tissue Constructs
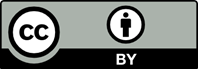
Three-dimensional (3D) bioprinting has emerged as a promising method for the engineering of tissues and organs. Still, it faces challenges in its widespread use due to issues with the development of bioink materials and the nutrient diffusion barrier inherent to these scaffold materials. Herein, we introduce a method to promote oxygen diffusion throughout the printed constructs using genetically encoded gas vesicles derived from haloarchaea. These hollow nanostructures are composed of a protein shell that allows gases to permeate freely while excluding the water flow. After printing cells with gas vesicles of various concentrations, the cells were observed to have increased activity and proliferation. These results suggest that air-filled gas vesicles can help overcome the diffusion barrier throughout the 3D bioprinted constructs by increasing oxygen availability to cells within the center of the construct. The biodegradable nature of the gas vesicle proteins combined with our promising results encourage their potential use as oxygen-promoting materials in biological samples.
1. Cui H, Nowicki M, Fisher JP, et al., 2017, 3D Bioprinting for Organ Regeneration. Adv Healthc Mater, 6:1601118. https://doi.org/10.1002/adhm.201601118
2. Khademhosseini A, Langer R, 2016, A decade of Progress in Tissue Engineering. Nat Protoc, 11:1775–81. https://doi.org/10.1038/nprot.2016.123
3. Matai I, Kaur G, Seyedsalehi A, et al., 2020, Progress in 3D Bioprinting Technology for Tissue/Organ Regenerative Engineering. Biomaterials, 226:119536. https://doi.org/10.1016/j.biomaterials.2019.119536
4. Ng WL, Chua CK, Shen YF, 2019, Print me an Organ! Why we are not there yet. Prog Polym Sci, 97:101145. https://doi.org/10.1016/j.progpolymsci.2019.101145
5. Bishop ES, Mostafa S, Pakvasa M, et al., 2017, 3-D Bioprinting Technologies in Tissue Engineering and Regenerative Medicine: Current and Future Trends. Genes Dis, 4:185–95. https://doi.org/10.1016/j.gendis.2017.10.002
6. Murphy SV, Atala A, 2014, 3D Bioprinting of Tissues and Organs. Nat Biotechnol, 32:773–85. https://doi.org/10.1038/nbt.2958
7. Seol YJ, Kang HW, Lee SJ, et al., 2014, Bioprinting Technology and its Applications. Eur J Cardio Thorac Surg, 46:342–8. https://doi.org/10.1093/ejcts/ezu148
8. Li X, Liu B, Pei B, et al., 2020, Inkjet Bioprinting of Biomaterials. Chem Rev, 120:10793–833. https://doi.org/10.1021/acs.chemrev.0c00008
9. Ng WL, Lee JM, Yeong WY, et al., 2017, Microvalve-based Bioprinting-process, Bio-inks and Applications. Biomater Sci, 5:632–47.
10. Jentsch S, Nasehi R, Kuckelkorn C, et al., 2021, Multiscale 3D Bioprinting by Nozzle‐Free Acoustic Droplet Ejection. Small Methods, 5:2000971. https://doi.org/10.1002/smtd.202000971
11. Dou C, Perez V, Qu J, et al., 2021, A State‐of‐the‐Art Review of Laser‐Assisted Bioprinting and its Future Research Trends. ChemBioEng Rev, 8:517–34. https://doi.org/10.1002/cben.202000037
12. Fu Z, Naghieh S, Xu C, et al., 2021, Printability in Extrusion Bioprinting. Biofabrication, 13:033001. https://doi.org/10.1088/1758-5090/abe7ab
13. Ng WL, Lee JM, Zhou M, et al., 2020, Vat Polymerization based Bioprinting-process, Materials, Applications and Regulatory Challenges. Biofabrication, 12:022001. https://doi.org/10.1088/1758-5090/ab6034
14. Ravnic DJ, Leberfinger AN, Koduru SV, et al., 2017, Transplantation of Bioprinted Tissues and Organs: Technical and Clinical Challenges and Future Perspectives. Ann Surg, 266:48-58. https://doi.org/10.1097/sla.0000000000002141
15. Hauser CA, Zhang SG, 2010, Designer Self-assembling Peptide Nanofiber Biological Materials. Chem Soc Rev, 39:2780–90. https://doi.org/10.1039/b921448h
16. Chen C, Bang S, Cho Y, et al., 2016, Research Trends in Biomimetic Medical Materials for Tissue Engineering: 3D Bioprinting, Surface Modification, Nano/Micro-technology and Clinical Aspects in Tissue Engineering of Cartilage and Bone. Biomater Res, 20:10. https://doi.org/10.1186/s40824-016-0057-3
17. Visconti RP, Kasyanov V, Gentile C, et al., 2010, Towards Organ Printing: Engineering an Intra-organ Branched Vascular Tree. Expert Opin Biol Ther, 10:409–20. https://doi.org/10.1517/14712590903563352
18. Kolesky DB, Homan KA, Skylar-Scott MA, et al., 2016, Three-dimensional Bioprinting of Thick Vascularized Tissues. Proc Natl Acad Sci, 113:3179–84. https://doi.org/10.1073/pnas.1521342113
19. Zhang B, Luo Y, Ma L, et al., 2018, 3D Bioprinting: An Emerging Technology Full of Opportunities and Challenges. Biodes Manuf, 1:2-13. https://doi.org/10.1007/s42242-018-0004-3
20. Zhang YS, Yue K, Aleman J, et al., 2017, 3D Bioprinting for Tissue and Organ Fabrication. Ann Biomed Eng, 45:148–63. https://doi.org/10.1007/s10439-016-1612-8
21. Suvarnapathaki S, Wu X, Lantigua D, et al., 2019, Breathing Life into Engineered Tissues Using Oxygen-releasing Biomaterials. NPG Asia Mater, 11:65. https://doi.org/10.1038/s41427-019-0166-2
22. Erdem A, Darabi MA, Nasiri R, et al., 2020, 3D Bioprinting of Oxygenated Cell‐Laden Gelatin Methacryloyl Constructs. Adv Healthc Mater, 9:1901794. https://doi.org/10.1002/adhm.201901794
23. Pedraza E, Coronel MM, Fraker CA, et al., 2012, Preventing Hypoxia-induced Cell Death in Beta Cells and Islets Via Hydrolytically Activated, Oxygen-generating Biomaterials. Proc Natl Acad Sci U S A, 109:4245–4250. https://doi.org/10.1073/pnas.1113560109
24. McQuilling JP, Sittadjody S, Pendergraft S, et al., 2017, Applications of Particulate Oxygen-generating Substances (POGS) in the Bioartificial Pancreas. Biomater Sci, 5:2437–47. https://doi.org/10.1039/c7bm00790f
25. Ward KR, Huvard GS, McHugh M, et al., 2013, Chemical Oxygen Generation. Respir Care, 58:184–95. https://doi.org/10.4187/respcare.01983
26. Pfeifer F, 2015, Haloarchaea and the Formation of Gas Vesicles. Life, 5:385–402.
27. DasSarma S, Karan R, DasSarma P, et al., 2013, An Improved Genetic System for Bioengineering Buoyant Gas Vesicle Nanoparticles from Haloarchaea. BMC Biotechnol, 13:112. https://doi.org/10.1186/1472-6750-13-112
28. Kunth M, Lu GJ, Witte C, et al., 2018, Protein Nanostructures Produce Self-Adjusting Hyperpolarized Magnetic Resonance Imaging Contrast through Physical Gas Partitioning. ACS Nano, 12:10939–48. https://doi.org/10.1021/acsnano.8b04222
29. Pfeifer F, 2012, Distribution, Formation and Regulation of Gas Vesicles. Nat Rev Microbiol, 10:705–15. https://doi.org/10.1038/nrmicro2834
30. Andar AU, Karan R, Pecher WT, et al., 2017, Microneedle-Assisted Skin Permeation by Nontoxic Bioengineerable Gas Vesicle Nanoparticles. Mol Pharm, 14:953–8. https://doi.org/10.1021/acs.molpharmaceut.6b00859
31. Lu GJ, Chou LD, Malounda D, et al., 2020, Genetically Encodable Contrast Agents for Optical Coherence Tomography. ACS Nano, 14:7823–31. https://doi.org/10.1021/acsnano.9b08432
32. Szablowski JO, Bar-Zion A, Shapiro MG, 2019, Achieving Spatial and Molecular Specificity with Ultrasound-targeted Biomolecular Nanotherapeutics. Acc Chem Res, 52:2427–34. https://doi.org/10.1021/acs.accounts.9b00277
33. Lu GJ, Farhadi A, Szablowski JO, et al., 2018, Acoustically Modulated Magnetic Resonance Imaging of Gas-filled Protein Nanostructures. Nat Mater, 17:456–63. https://doi.org/10.1038 s41563-018-0023-7
34. Lakshmanan A, Farhadi A, Nety SP, et al., 2016, Molecular Engineering of Acoustic Protein Nanostructures. ACS Nano, 10:7314–22. https://doi.org/10.1021/acsnano.6b03364
35. DasSarma P, Negi VD, Balakrishnan A, et al., 2014, Haloarchaeal Gas Vesicle Nanoparticles Displaying Salmonella SopB Antigen Reduce Bacterial Burden when Administered with Live Attenuated Bacteria. Vaccine, 32:4543–9. https://doi.org/10.1016/j.vaccine.2014.06.021
36. Balakrishnan A, DasSarma P, Bhattacharjee O, et al., 2016, Halobacterial nano vesicles displaying murine bactericidal permeability-increasing protein rescue mice from lethal endotoxic shock. Sci Rep, 6:1–11. https://doi.org/10.1038/srep33679
37. Loo Y, Lakshmanan A, Ni M, et al., 2015, Peptide Bioink: Self-assembling Nanofibrous Scaffolds for Three-dimensional Organotypic Cultures. Nano Lett, 15:6919–25. https://doi.org/10.1021/acs.nanolett.5b02859
38. Loo Y, Hauser CA, 2015, Bioprinting Synthetic Self assembling Peptide Hydrogels for Biomedical Applications. Biomed Mater, 11:014103. https://doi.org/10.1088/1748-6041/11/1/014103
39. Sundaramurthi D, Rauf S, Hauser C, 2016, 3D Bioprinting Technology for Regenerative Medicine Applications. Int J Bioprint, 2:78. https://doi.org/10.18063/IJB.2016.02.010
40. Khan Z, Kahin K, Rauf S, et al., 2019, Optimization of a 3D Bioprinting Process Using Ultrashort Peptide Bioinks. Int J Bioprint, 5:173. https://doi.org/10.18063/ijb.v5i1.173
41. Kahin K, Khan Z, Albagami M, et al., 2019, Development of a Robotic 3D Bioprinting and Microfluidic Pumping System for Tissue and Organ Engineering, Microfluidics, BioMEMS, and Medical Microsystems XVII. International Society for Optics and Photonics, 108750Q.
42. Rauf S, Susapto HH, Kahin K, et al., 2020, Self assembling Tetrameric Peptides Allow In Situ 3D Bioprinting under Physiological Conditions. J Mater Chem B, 9:1069–81. https://doi.org/10.1039/D0TB02424D
43. Susapto HH, Alhattab D, Abdelrahman S, et al., 2021, Ultrashort Peptide Bioinks Support Automated Printing of Large-Scale Constructs Assuring Long-Term Survival of Printed Tissue Constructs. Nano Lett, 21:2719–29. https://doi.org/10.1021/acs.nanolett.0c04426
44. Sundararajan A, Ju LK, 2006, Use of Cyanobacterial Gas Vesicles as Oxygen Carriers in Cell Culture. Cytotechnology, 52:139–49. https://doi.org/10.1007/s10616-007-9044-9
45. DasSarma S, Fleischmann E, Rodriguez-Valera F, 1995, Media for halophiles. In: Robb FT, Place AR, Sowers KR, Schreier HJ, DasSarma S, Fleischmann ME, editors. Archaea: A Laboratory Manual. Vol. 1. Cold Spring Harbor, NY: Cold Spring Harbor Laboratory Press, p225–30.
46. Strillinger E, Grötzinger SW, Allers T, et al., 2016, Production of Halophilic Proteins Using Haloferax volcanii H1895 in a Stirred-tank Bioreactor. Appl Microbiol Biotechnol, 100:1183–95. https://doi.org/10.1007/s00253-015-7007-1
47. Grotzinger SW, Karan R, Strillinger E, et al., 2018, Identification and Experimental Characterization of an Extremophilic Brine Pool Alcohol Dehydrogenase from Single Amplified Genomes. ACS Chem Biol, 13:161–70. https://doi.org/10.1021/acschembio.7b00792
48. Grote A, Hiller K, Scheer M, et al., 2005, JCat: A Novel Tool to Adapt Codon Usage of a Target Gene to its Potential Expression Host. Nucleic Acids Res, 33(Web Server issue):W526–31. https://doi.org/10.1093/nar/gki376
49. Dyall-Smith M, 2008, The Halohandbook-Protocols for Haloarchaeal Genetics, Version 7.0. Bathurst, NSW, Australia. Available from: https://haloarchaea.com/wpcontent/ uploads/2018/10/Halohandbook_2009_v7.3mds.pdf
50. Ghalayini S, Susapto HH, Hall S, et al., 2019, Preparation and Printability of Ultrashort Self-assembling Peptide Nanoparticles. Int J Bioprint, 5:239. https://doi.org/10.18063/ijb.v5i2.239
51. Akal AL, Karan R, Hohl A, et al., 2019, A Polyextremophilic Alcohol Dehydrogenase from the Atlantis II Deep Red Sea Brine Pool. FEBS Open Bio, 9:194–205. https://doi.org/10.1002/2211-5463.12557
52. Silva IC, Delgado AB, Silva PH, et al., 2018, Focused ultrasound and Alzheimer’s disease A systematic review. Dement Neuropsychol, 12:353–9. https://doi.org/10.1590/1980-57642018dn12-040003
53. Hauser CA, Deng R, Mishra A, et al., 2011, Natural Tri- to Hexapeptides Self-assemble in Water to Amyloid Beta-type Fiber Aggregates by Unexpected Alpha-helical Intermediate Structures. Proc Natl Acad Sci U S A, 108:1361–6. https://doi.org/10.1073/pnas.1014796108
54. Alshehri S, Susapto HH, Hauser CA, 2021, Scaffolds from Self-Assembling Tetrapeptides Support 3D Spreading, Osteogenic Differentiation, and Angiogenesis of Mesenchymal Stem Cells. Biomacromolecules, 22:2094–106. https://doi.org/10.1021/acs.biomac.1c00205
55. Gustavo Ramirez-Calderon H H S a C A E H, 2021, Delivery of Endothelial Cell-Laden Microgel Elicits Angiogenesis in Self-Assembling Ultrashort Peptide Hydrogels In Vitro. ACS Appl Mater Interfaces, 13:29281–92. https://doi.org/10.1021/acsami.1c03787
56. Stuart ES, Morshed F, Sremac M, et al., 2001, Antigen Presentation Using Novel Particulate Organelles from Halophilic Archaea. J Biotechnol, 88:119–28. https://doi.org/10.1016/S0168-1656(01)00267-X
57. DasSarma P, Negi V, Balakrishnan A, et al., 2015, Haloarchaeal Gas Vesicle Nanoparticles Displaying Salmonella Antigens as a Novel Approach to Vaccine Development. Proc Vaccinol, 9:16. https://doi.org/10.1016/j.provac.2015.05.003
58. Sremac M, Stuart ES, 2008, Recombinant Gas Vesicles from Halobacterium sp. Displaying SIV Peptides Demonstrate Biotechnology Potential as a Pathogen Peptide Delivery Vehicle. BMC Biotechnol, 8:9. https://doi.org/10.1186/1472-6750-8-9
59. Stuart ES, Morshed F, Sremac M, et al., 2004, Cassette-based Presentation of SIV Epitopes with Recombinant Gas Vesicles from Halophilic Archaea. J Biotechnol, 114:225–37. https://doi.org/10.1016/j.jbiotec.2004.01.005
60. Khan Z, Kahin K, Melle F, et al., 2019, Assessing the Bioprintability of Self-Assembling Peptide Bioinks in Terms of Structure Fidelity and Cell Viability. 9th International Conference on Advances in Applied Science and Environmental Technology, p8-14.
61. Vogler M, Karan R, Renn D, et al., 2020, Crystal Structure and Active Site Engineering of a Halophilic gamma-Carbonic Anhydrase. Front Microbiol, 11:742. https://doi.org/10.3389/fmicb.2020.00742
62. Karan R, Mathew S, Muhammad R, et al., 2020, Understanding High-salt and Cold Adaptation of a Polyextremophilic Enzyme. Microorganisms, 8:1594. https://doi.org/10.3390/microorganisms8101594
63. Graham AD, Olof SN, Burke MJ, et al., 2017, High-resolution Patterned Cellular Constructs by Droplet-based 3D Printing. Sci Rep, 7:7004. https://doi.org/10.1038/s41598-017-06358-x
64. Nair K, Gandhi M, Khalil S, et al., 2009, Characterization of Cell Viability during Bioprinting Processes. Biotechnol J, 4:1168–77. https://doi.org/10.1002/biot.200900004
65. Yu Y, Zhang Y, Martin JA, et al., 2013, Evaluation of Cell Viability and Functionality in Vessel-like Bioprintable Cell laden Tubular Channels. J Biomech Eng, 135:91011. https://doi.org/10.1115/1.4024575
66. Yan KC, Nair K, Sun W, 2010, Three Dimensional Multi-scale Modelling and Analysis of Cell Damage in Cell-encapsulated Alginate Constructs. J Biomech, 43:1031–8. https://doi.org/10.1016/j.jbiomech.2009.12.018
67. Jakab K, Norotte C, Marga F, et al., 2010, Tissue Engineering by Self-assembly and Bio-printing of Living Cells. Biofabrication, 2:022001. https://doi.org/10.1088/1758-5082/2/2/022001
68. De Jong OG, Van Balkom BW, Schiffelers RM, et al., 2014, Extracellular Vesicles: Potential Roles in Regenerative Medicine. Front Immunol, 5:608. https://doi.org/10.3389/fimmu.2014.00608
69. DasSarma S, DasSarma P, 2015, Gas Vesicle Nanoparticles for Antigen Display. Vaccines, 3:686–702. https://doi.org/10.3390/vaccines3030686
70. Dutta S, DasSarma P, DasSarma S, et al., 2015, Immunogenicity and Protective Potential of a Plasmodium spp. Enolase Peptide Displayed on Archaeal Gas Vesicle Nanoparticles. Malaria J, 14:406. https://doi.org/10.1186/s12936-015-0914