Adenine base editing as a promising therapy for cardiovascular diseases
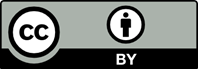
Cardiovascular diseases (CVDs) are the leading causes of human death worldwide. Genetic variants serve as the major risk factor for CVDs, with limited therapeutic interventions in clinical practice. The recent surge of genome editing technologies offers the hope to correct genetic variants and to cure genetic diseases. Among the diverse genome editing tools, adenine base editors (ABEs) exhibit high efficiency, high specificity, and low off-target effects, successfully entering a clinical trial and demonstrating the tremendous potential to transform modern cardiovascular therapy. In this review, we summarize the basic knowledge about ABE, showcase three hallmark studies using ABE to ameliorate or treat CVDs in experimental animals, and lastly discuss about the key technical concerns that should be addressed to achieve the full potential of ABEs in the future.
Cambien F, Tiret L. 2007, Genetics of cardiovascular diseases: From single mutations to the whole genome. Circulation, 116: 1714–1724. https://doi.org/10.1161/CIRCULATIONAHA.106.661751
Humphries SE. 2017, Common variants for cardiovascular disease: Clinical utility confirmed. Circulation, 135: 2102–2105. https://doi.org/10.1161/CIRCULATIONAHA.117.027798
Zhuang X, Tian M, Li L, et al., 2022, Identification of potential hub genes for the diagnosis and therapy of dilated cardiomyopathy with heart failure through bioinformatics analysis. GTM, 1: 104. https://doi.org/10.36922/gtm.v1i1.104
Kalayinia S, Goodarzynejad H, Maleki M, et al., 2018, Next generation sequencing applications for cardiovascular disease. Ann Med, 50:91–109. https://doi.org/10.1080/07853890.2017.1392595
Jiang W, Marraffini LA. 2015, CRISPR-Cas: New tools for genetic manipulations from bacterial immunity systems. Annu Rev Microbiol, 69: 209–228. https://doi.org/10.1146/annurev-micro-091014-104441
Marraffini LA. 2015, CRISPR-Cas immunity in prokaryotes. Nature, 526: 55–61. https://doi.org/10.1038/nature15386
Jinek M, Chylinski K, Fonfara I, et al., 2012, A programmable dual-RNA-guided DNA endonuclease in adaptive bacterial immunity. Science (New York, N.Y.), 337: 816–821. https://doi.org/10.1126/science.1225829
Komor AC, Kim YB, Packer MS, et al., 2016, Programmable editing of a target base in genomic DNA without double-stranded DNA cleavage. Nature, 533: 420–424. https://doi.org/10.1038/nature17946
Gaudelli NM, Komor AC, Rees HA, et al., 2017, Programmable base editing of A•T to G•C in genomic DNA without DNA cleavage. Nature, 551: 464–471. https://doi.org/10.1038/nature24644
Landrum MJ, Lee JM, Benson M, et al., 2016, ClinVar: Public archive of interpretations of clinically relevant variants. Nucleic Acids Res, 44: D862–D868. https://doi.org/10.1093/nar/gkv1222
Jin S, Zong Y, Gao G, et al., 2019, Cytosine, but not adenine, base editors induce genome-wide off-target mutations in rice. Science (New York, N.Y.), 364: 292–295. https://doi.org/10.1126/science.aaw7166
Zuo E, Sun Y, Wei W, et al., 2019, Cytosine base editor generates substantial off-target single-nucleotide variants in mouse embryos. Science, 364: 289–292. https://doi.org/10.1126/science.aav9973
Koblan LW, Doman JL, Wilson C, et al., 2018, Improving cytidine and adenine base editors by expression optimization and ancestral reconstruction. Nat Biotechnol, 36: 843–846. https://doi.org/10.1038/nbt.4172
Huang S, Liao Z, Li X, et al., 2019, Developing ABEmax-NG with precise targeting and expanded editing scope to model pathogenic splice site mutations in vivo. iScience, 15: 640–648. https://doi.org/10.1016/j.isci.2019.05.008
Richter MF, Zhao KT, Eton E, et al., 2020, Phage-assisted evolution of an adenine base editor with improved Cas domain compatibility and activity. Nat Biotechnol, 38: 883–891. https://doi.org/10.1038/s41587-020-0453-z
Gaudelli NM, Lam DK, Rees HA, et al., 2020, Directed evolution of adenine base editors with increased activity and therapeutic application. Nat Biotechnol, 38: 892–900. https://doi.org/10.1038/s41587-020-0491-6
Hu JH, Miller SM, Geurts MH, et al., 2018, Evolved Cas9 variants with broad PAM compatibility and high DNA specificity. Nature, 556: 57–63. https://doi.org/10.1038/nature26155
Hsu PD, Scott DA, Weinstein JA, et al., 2013, DNA targeting specificity of RNA-guided Cas9 nucleases. Nat Biotechnol, 31: 827–832. https://doi.org/10.1038/nbt.2647
Ran FA, Cong L, Yan WX, et al., 2015, In vivo genome editing using Staphylococcus aureus Cas9. Nature, 520: 186–191. https://doi.org/10.1038/nature14299
Jiang F, Doudna JA. 2017, CRISPR-Cas9 structures and mechanisms. Annu Rev Biophys, 46: 505–529. https://doi.org/10.1146/annurev-biophys-062215-010822.
Makarova KS, Wolf YI, Alkhnbashi OS, et al., 2015, An updated evolutionary classification of CRISPR-Cas systems. Nat Rev Microbiol, 13: 722–736. https://doi.org/10.1038/nrmicro3569
Makarova KS, Wolf YI, Iranzo J, et al., 2020, Evolutionary classification of CRISPR-Cas systems: A burst of class 2 and derived variants. Nat Rev Microbiol, 18: 67–83. https://doi.org/10.1038/s41579-019-0299-x
Kleinstiver BP, Pattanayak V, Prew MS, et al., 2016, High-fidelity CRISPR-Cas9 nucleases with no detectable genome-wide off-target effects. Nature, 529: 490–495. https://doi.org/10.1038/nature16526
Nishimasu H, Shi X, Ishiguro S, et al., 2018, Engineered CRISPR-Cas9 nuclease with expanded targeting space. Science, 361: 1259–1262. https://doi.org/10.1126/science.aas9129
Walton RT, Christie KA, Whittaker MN, et al., 2020, Unconstrained genome targeting with near-PAMless engineered CRISPR-Cas9 variants. Science, 368: 290–296. https://doi.org/10.1126/science.aba8853
Hermonat PL, Muzyczka N. 1984, Use of adeno-associated virus as a mammalian DNA cloning vector: Transduction of neomycin resistance into mammalian tissue culture cells. Proc Natl Acad Sci U S A, 81: 6466–6470. https://doi.org/10.1073/pnas.81.20.6466
Tratschin JD, West MH, Sandbank T, et al., 1984, A human parvovirus, adeno-associated virus, as a eucaryotic vector: Transient expression and encapsidation of the procaryotic gene for chloramphenicol acetyltransferase. Mol Cell Biol, 4: 2072–2081. https://doi.org/10.1128/mcb.4.10.2072-2081
Pupo A, Fernández A, Low Sh, et al., 2022, AAAV vectors: The Rubik’s cube of human gene therapy. Mol Ther, 30: 3515–3541. https://doi.org/10.1016/j.ymthe.2022.09.015
Truong DJ, Kühner K, Kühn R, et al., 2015, Development of an intein-mediated split-Cas9 system for gene therapy. Nucleic Acids Res, 43, 6450–6458. https://doi.org/10.1093/nar/gkv601
Koblan LW, Erdos MR, Wilson C, 2021, In vivo base editing rescues Hutchinson-Gilford progeria syndrome in mice. Nature, 589: 608–614. https://doi.org/10.1038/s41586-020-03086-7
Davis JR, Wang X, Witte TP, et al., 2022, Efficient in vivo base editing via single adeno-associated viruses with size-optimized genomes encoding compact adenine base editors. Nat Biomed Eng, 6: 1272–1283. https://doi.org/10.1038/s41551-022-00911-4
Albertsen CH, Kulkarni JA, Witzigmann D, et al., 2022, The role of lipid components in lipid nanoparticles for vaccines and gene therapy. Adv Drug Deliv Rev, 188: 114416. https://doi.org/10.1016/j.addr.2022.114416
Cheng Q, Wei T, Farbiak L, et al., 2020, Selective organ targeting (SORT) nanoparticles for tissue-specific mRNA delivery and CRISPR-Cas gene editing. Nat Nanotechnol, 15: 313–320. https://doi.org/10.1038/s41565-020-0669-6
Hoy SM. 2018, Patisiran: First global approval. Drugs, 78: 1625–1631. https://doi.org/10.1007/s40265-018-0983-6
Ahmed MS, Ikram S, Bibi N, et al., 2018, Hutchinson- Gilford progeria syndrome: A premature aging disease. Mol Neurobiol, 55: 4417–4427. https://doi.org/10.1007/s12035-017-0610-7
De Sandre-Giovannoli A, Bernard R, Cau P, et al., 2003, Lamin a truncation in Hutchinson-Gilford progeria. Science, 300: 2055. https://doi.org/10.1126/science.1084125
Eriksson M, Brown WT, Gordon LB, et al., 2003, Recurrent de novo point mutations in lamin A cause Hutchinson- Gilford progeria syndrome. Nature, 423: 293–298. https://doi.org/10.1038/nature01629
Dechat T, Shimi T, Adam SA, et al., 2007, Alterations in mitosis and cell cycle progression caused by a mutant lamin A known to accelerate human aging. Proc Natl Acad Sci U S A, 104: 4955–4960. https://doi.org/10.1073/pnas.0700854104
Dhillon S. 2021, Lonafarnib: First approval. Drugs, 2021, 81: 283–289. https://doi.org/10.1007/s40265-020-01464-z
Maron BJ, Gardin JM, Flack JM, et al., 1995, Prevalence of hypertrophic cardiomyopathy in a general population of young adults. Echocardiographic analysis of 4111 subjects in the CARDIA Study. Coronary Artery Risk Development in (Young) Adults. Circulation, 92: 785–789. https://doi.org/10.1161/01.cir.92.4.785
Tuohy CV, Kaul S, Song HK, et al., 2020, Hypertrophic cardiomyopathy: The future of treatment. Eur J Heart Fail, 22: 228–240. https://doi.org/10.1002/ejhf.1715
Sabater-Molina M, Pérez-Sánchez I, Del Rincón JP, et al., 2018, Genetics of hypertrophic cardiomyopathy: A review of current state. Clin Genet, 93: 3–14. https://doi.org/10.1111/cge.13027
Keam SJ. 2022, Mavacamten: First approval. Drugs, 82: 1127–1135. https://doi.org/10.1007/s40265-022-01739-7
Saberi S, Cardim N, Yamani M, et al., 2021, Mavacamten favorably impacts cardiac structure in obstructive hypertrophic cardiomyopathy: EXPLORER-HCM cardiac magnetic resonance substudy analysis. Circulation, 143: 606–608. https://doi.org/10.1161/circulationaha.120.052359
Hwee DT, Hartman JJ, Wang J, et al., 2019, Abstract 332: Pharmacologic characterization of the cardiac myosin inhibitor, CK-3773274: A potential therapeutic approach for hypertrophic cardiomyopathy. Circ Res, 125: A332–A332. https://doi.org/10.1161/res.125.suppl_1.332
Ma S, Jiang W, Liu X, et al., 2021, Efficient correction of a hypertrophic cardiomyopathy mutation by ABEmax-NG. Circ Res, 129: 895–908. https://doi.org/10.1161/CIRCRESAHA.120.318674
Halpern J, O’Hara SE, Doxzen KW, et al., 2019, Societal and ethical impacts of germline genome editing: How can we secure human rights? CRISPR J, 2: 293–298. https://doi.org/10.1089/crispr.2019.0042
Guo Y, Pu WT. 2020, Cardiomyocyte maturation: New phase in development. Circ Res, 126: 1086–1106. https://doi.org/10.1161/CIRCRESAHA.119.315862
Virani SS, Alonso A, Benjamin EJ, et al., 2020, Heart disease and stroke statistics-2020 update: A report from the American Heart Association. Circulation, 141: e139–e596, https://doi.org/10.1161/cir.0000000000000757
Chen H, Howatt DA, Franklin MK, et al., 2022, A mini-review on quantification of atherosclerosis in hypercholesterolemic mice. GTM, 1: 72. https://doi.org/10.36922/gtm.v1i1.76
Ference BA, Ginsberg HN, Graham I, et al., 2017, Low-density lipoproteins cause atherosclerotic cardiovascular disease. 1. Evidence from genetic, epidemiologic, and clinical studies. A consensus statement from the European Atherosclerosis Society Consensus Panel. Eur Heart J, 38: 2459–2472. https://doi.org/10.1093/eurheartj/ehx144
Qian YW, Schmidt RJ, Zhang Y, et al., 2007, Secreted PCSK9 downregulates low density lipoprotein receptor through receptor-mediated endocytosis. J Lipid Res, 48: 1488–1498. https://doi.org/10.1194/jlr.M700071-JLR200
Cohen J, Pertsemlidis A, Kotowski IK, 2005, Low LDL cholesterol in individuals of African descent resulting from frequent nonsense mutations in PCSK9. Nat Genet, 37: 161–165. https://doi.org/10.1038/ng1509
Cohen JC, Boerwinkle E, Mosley TH Jr., et al., 2006, Sequence variations in PCSK9, low LDL, and protection against coronary heart disease. N Engl J Med, 354: 1264–1272. https://doi.org/10.1056/NEJMoa054013
Musunuru K, Chadwick AC, Mizoguchi T, et al., 2021, In vivo CRISPR base editing of PCSK9 durably lowers cholesterol in primates. Nature, 593: 429–434. https://doi.org/10.1038/s41586-021-03534-y
Ledford H. 2022, CRISPR ‘cousin’ put to the test in landmark heart-disease trial. Nature, 607: 647. https://doi.org/10.1038/d41586-022-01951-1
Miller SM, Wang T, Randolph PB, et al., 2020, Continuous evolution of SpCas9 variants compatible with non-G PAMs. Nat Biotechnol, 38: 471–481. https://doi.org/10.1038/s41587-020-0412-8
Huang TP, Zhao KT, Miller SM, et al., 2019, Circularly permuted and PAM-modified Cas9 variants broaden the targeting scope of base editors. Nat Biotechnol, 37: 626–631. https://doi.org/10.1038/s41587-019-0134-y
Chen L, Zhang S, Xue N, et al., 2022, Engineering a precise adenine base editor with minimal bystander editing. Nat Chem Biol, 19: 101–110. https://doi.org/10.1038/s41589-022-01163-8
Marquart KF, Allam A, Janjuha S, et al., 2021, Predicting base editing outcomes with an attention-based deep learning algorithm trained on high-throughput target library screens. Nat Commun, 12: 5114. https://doi.org/10.1038/s41467-021-25375-z
Pallaseni A, Peets EM, Koeppel J, et al., 2022, Predicting base editing outcomes using position-specific sequence determinants. Nucleic Acids Res, 50: 3551–3564. https://doi.org/10.1093/nar/gkac161
Arbab M, Shen MW, Mok B, et al., 2020, Determinants of base editing outcomes from target library analysis and machine learning. Cell, 182: 463–480.e430. https://doi.org/10.1016/j.cell.2020.05.037
Winter J, Luu A, Gapinske M, et al., 2019, Targeted exon skipping with AAV-mediated split adenine base editors. Cell Discov, 5: 41. https://doi.org/10.1038/s41421-019-0109-7
Zhou C, Sun Y, Yan R, et al., 2019, Off-target RNA mutation induced by DNA base editing and its elimination by mutagenesis. Nature, 571: 275–278. https://doi.org/10.1038/s41586-019-1314-0
Fu Y, Foden JA, Khayter C, et al., 2013, High-frequency off-target mutagenesis induced by CRISPR-Cas nucleases in human cells. Nat Biotechnol, 31: 822–826. https://doi.org/10.1038/nbt.2623
Pattanayak V, Lin S, Guilinger JP, et al., 2013, High-throughput profiling of off-target DNA cleavage reveals RNA-programmed Cas9 nuclease specificity. Nat Biotechnol, 31: 839–843. https://doi.org/10.1038/nbt.2673
Doman JL, Raguram A, Newby GA, et al., 2020, Evaluation and minimization of Cas9-independent off-target DNA editing by cytosine base editors. Nat Biotechnol, 38: 620–628. https://doi.org/10.1038/s41587-020-0414-6
Bae S, Park J, Kim JS. 2014, Cas-OFFinder: A fast and versatile algorithm that searches for potential off-target sites of Cas9 RNA-guided endonucleases. Bioinformatics, 30: 1473–1475. https://doi.org/10.1093/bioinformatics/btu048
Xiao A, Cheng Z, Kong L, et al., 2014, CasOT: A genome-wide Cas9/gRNA off-target searching tool. Bioinformatics, 30: 1180–1182. https://doi.org/10.1093/bioinformatics/btt764
Chuai G, Ma H, Yan J, et al., 2018, DeepCRISPR: Optimized CRISPR guide RNA design by deep learning. Genome Biol, 19: 80. https://doi.org/10.1186/s13059-018-1459-4
Listgarten J, Weinstein M, Kleinstiver BP, et al., 2018, Prediction of off-target activities for the end-to-end design of CRISPR guide RNAs. Nat Biomed Eng, 2: 38–47. https://doi.org/10.1038/s41551-017-0178-6
Liang P, Xie X, Zhi S, et al., 2019, Genome-wide profiling of adenine base editor specificity by EndoV-seq. Nat Commun, 10: 67. https://doi.org/10.1038/s41467-018-07988-z
Cameron P, Fuller CK, Donohoue PD, et al., 2017, Mapping the genomic landscape of CRISPR-Cas9 cleavage. Nat Methods, 14: 600–606. https://doi.org/10.1038/nmeth.4284
Kim D, Bae S, Park J, et al., 2015, Digenome-seq: Genome-wide profiling of CRISPR-Cas9 off-target effects in human cells. Nat Methods, 12: 237–243, 1 p following 243. https://doi.org/10.1038/nmeth.3284
Tsai SQ, Nguyen NT, Malagon-Lopez J, et al., 2017, CIRCLE-seq: A highly sensitive in vitro screen for genome-wide CRISPR-Cas9 nuclease off-targets. Nat Methods, 14: 607–614. https://doi.org/10.1038/nmeth.4278
Tsai SQ, Zheng Z, Nguyen NT, et al., 2015, GUIDE-seq enables genome-wide profiling of off-target cleavage by CRISPR-Cas nucleases. Nat Biotechnol, 33: 187–197. https://doi.org/10.1038/nbt.3117
Wang X, Wang Y, Wu X, et al., 2015, Unbiased detection of off-target cleavage by CRISPR-Cas9 and TALENs using integrase-defective lentiviral vectors. Nat Biotechnol, 33: 175–178. https://doi.org/10.1038/nbt.3127
Iyer V, Shen B, Zhang W, et al., 2015, Off-target mutations are rare in Cas9-modified mice. Nat Methods, 12: 479. https://doi.org/10.1038/nmeth.3408
Wienert B, Wyman SK, Richardson CD, et al., 2019, Unbiased detection of CRISPR off-targets in vivo using DISCOVER-Seq. Science (New York, N.Y.), 364: 286–289. https://doi.org/10.1126/science.aav9023
Liang SQ, Liu P, Smith JL, et al., 2022, Genome-wide detection of CRISPR editing in vivo using GUIDE-tag. Nat Commun, 13: 437. https://doi.org/10.1038/s41467-022-28135-9
Li J, Yu W, Huang S, et al., 2021, Structure-guided engineering of adenine base editor with minimized RNA off-targeting activity. Nat Commun, 12: 2287. https://doi.org/10.1038/s41467-021-22519-z