Neuroimaging associations with spatial navigation impairment in Alzheimer’s disease continuum: A narrative review
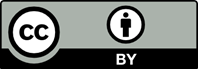
Identifying individuals with incipient Alzheimer’s disease (AD) are critical for early and targeted intervention before the dementia develops as AD progresses. Recently, emerging data have suggested that spatial navigation and neuroimaging could be utilized to identify individuals with prodromal AD. Compared to episodic memory, spatial navigation has fewer cultural and educational discrepancies and could serve as a promising marker for diagnosis and outcome measures in multicenter longitudinal studies with large cohorts. Furthermore, neuroimaging studies have contributed to our understanding of the structural and functional neural basis underlying spatial navigation and provided sensitive and non-invasive neuroimaging markers. The current review summarizes neuroimaging associations with spatial navigation impairment in the AD continuum, their potential pathophysiological mechanisms, and nonpharmacological interventions for spatial navigation impairments. We highlight the promising role of spatial navigation in the early identification of the preclinical and prodromal patients with potential risk of developing AD dementia. Multicenter large-scale longitudinal studies on patients across the AD continuum coupled with a standardized routine assessment of spatial navigation abilities in clinical settings are needed. This review may have implications for clinical practice and future research directions.
Dubois B, Villain N, Frisoni GB, et al., 2021, Clinical diagnosis of Alzheimer’s disease: Recommendations of the international working group. Lancet Neurol, 20(6): 484–496. https://doi.org/10.1016/s1474-4422(21)00066-1
Jack CR Jr., Bennett DA, Blennow K, et al., 2018, NIA-AA research framework: Toward a biological definition of Alzheimer’s disease. Alzheimers Dement, 14(4): 535–562. https://doi.org/10.1016/j.jalz.2018.02.018
Coughlan G, Laczo J, Hort J, et al., 2018, Spatial navigation deficits overlooked cognitive marker for preclinical Alzheimer disease? Nat Rev Neurol, 14(8): 496–506. https://doi.org/10.1038/s41582-018-0031-x
Gellersen HM, Coughlan G, Hornberger M, et al., 2021, Memory precision of object-location binding is unimpaired in APOE ε4-carriers with spatial navigation deficits. Brain Commun, 3(2): fcab087. https://doi.org/10.1093/braincomms/fcab087
Tuena C, Mancuso V, Stramba-Badiale C, et al., 2021, Egocentric and allocentric spatial memory in mild cognitive impairment with real-world and virtual navigation tasks: A systematic review. J Alzheimers Dis, 79(1): 95–116. https://doi.org/10.3233/jad-201017
Lithfous S, Dufour A, Després O, 2013, Spatial navigation in normal aging and the prodromal stage of Alzheimers disease: Insights from imaging and behavioral studies. Ageing Res Rev, 12(1): 201–213. https://doi.org/10.1016/j.arr.2012.04.007
Braak H, Del Tredici K, 2015, The preclinical phase of the pathological process underlying sporadic Alzheimer’s disease. Brain, 138(Pt 10): 2814–2833. https://doi.org/10.1093/brain/awv236
Howett D, Castegnaro A, Krzywicka K, et al., 2019, Differentiation of mild cognitive impairment using an entorhinal cortex-based test of virtual reality navigation. Brain, 142: 1751–1766. https://doi.org/10.1093/brain/awz116
Wang X, Huang W, Su L, et al., 2020, Neuroimaging advances regarding subjective cognitive decline in preclinical Alzheimer’s disease. Mol Neurodegene, 15(1): 55. https://doi.org/10.1186/s13024-020-00395-3
Gazova I, Vlcek K, Laczó J, et al., 2012, Spatial navigation-a unique window into physiological and pathological aging. Front Aging Neurosci, 4: 16. https://doi.org/10.3389/fnagi.2012.00016
Boccia M, Nemmi F, Guariglia C, 2014, Neuropsychology of environmental navigation in humans: Review and meta-analysis of FMRI studies in healthy participants. Neuropsychol Rev, 24(2): 236–251. https://doi.org/10.1007/s11065-014-9247-8
Muffato V, Meneghetti C, 2020, Learning a path from real navigation: The advantage of initial view, cardinal North and visuo-spatial ability. Brain Sci, 10(4): 204. https://doi.org/10.3390/brainsci10040204
Nedelska Z, Andel R, Laczo J, et al., 2012, Spatial navigation impairment is proportional to right hippocampal volume. Proc Natl Acad Sci U S A, 109(7): 2590–2594. https://doi.org/10.1073/pnas.1121588109
O’Keefe J, Nadel L, 1978, The Hippocampus as a Cognitive Map. Oxford: Clarendon Press Chicago.
Loomis JM, Klatzky RL, Golledge RG, et al., 1993, Nonvisual navigation by blind and sighted: Assessment of path integration ability. J Exp Psychol Gen, 122(1): 73–91. https://doi.org/10.1037//0096-3445.122.1.73
Serino S, Riva G, 2013, Getting lost in Alzheimer’s disease: A break in the mental frame syncing. Med Hypotheses, 80(4): 416–421. https://doi.org/10.1016/j.mehy.2012.12.031
Coutrot A, Silva R, Manley E, et al., 2018, Global determinants of navigation ability. Curr Biol, 28(17): 2861– 2866.e2864. https://doi.org/10.1016/j.cub.2018.06.009
Lester AW, Moffat SD, Wiener JM, et al., 2017, The aging navigational system. Neuron, 95(5): 1019–1035. https://doi.org/10.1016/j.neuron.2017.06.037
Vann SD, Aggleton JP, Maguire EA, 2009, What does the retrosplenial cortex do? Nat Rev Neurosci, 10(11): 792–802. https://doi.org/10.1038/nrn2733
Vass LK, Epstein RA, 2013, Abstract representations of location and facing direction in the human brain. J Neurosci, 33(14): 6133–6142. https://doi.org/10.1523/JNEUROSCI.3873-12.2013
Clark BJ, Simmons CM, Berkowitz LE, et al., 2018, The retrosplenial-parietal network and reference frame coordination for spatial navigation. Behav Neurosci, 132(5): 416–429. https://doi.org/10.1037/bne0000260
O’Keefe J, Dostrovsky J, 1971, The hippocampus as a spatial map. Preliminary evidence from unit activity in the freely-moving rat. Brain Res, 34: 171–175. https://doi.org/10.1016/0006-8993(71)90358-1
Taube JS, Muller RU, Ranck JB Jr., 1990, Head-direction cells recorded from the postsubiculum in freely moving rats. I. Description and quantitative analysis. J Neurosci, 10(2): 420–435. https://doi.org/10.1523/JNEUROSCI.10-02-00420.1990
Doeller CF, Barry C, Burgess N, 2010, Evidence for grid cells in a human memory network. Nature, 463: 657–661. https://doi.org/10.1038/nature08704
Hafting T, Fyhn M, Molden S, et al., 2005, Microstructure of a spatial map in the entorhinal cortex. Nature, 436(7052): 801–806. https://doi.org/10.1038/nature03721
Solstad T, Boccara CN, Kropff E, et al., 2008, Representation of geometric borders in the entorhinal cortex. Science, 322(5909): 1865–1868. https://doi.org/10.1126/science.1166466
Kropff E, Carmichael JE, Moser MB, et al., 2015, Speed cells in the medial entorhinal cortex. Nature, 523(7561): 419–424. https://doi.org/10.1038/nature14622
Kunz L, Schroder TN, Lee H, et al., 2015, Reduced grid-cell-like representations in adults at genetic risk for Alzheimer’s disease. Science, 350(6259): 430–433. https://doi.org/10.1126/science.aac8128
Ekstrom AD, Kahana MJ, Caplan JB, et al., 2003, Cellular networks underlying human spatial navigation. Nature, 425(6954): 184–188. https://doi.org/10.1038/nature01964
Diehl GW, Hon OJ, Leutgeb S, et al., 2017, Grid and nongrid cells in medial entorhinal cortex represent spatial location and environmental features with complementary coding schemes. Neuron, 94(1): 83–92 e86. https://doi.org/10.1016/j.neuron.2017.03.004
Lever C, Burton S, Jeewajee A, et al., 2009, Boundary vector cells in the subiculum of the hippocampal formation. J Neurosci, 29(31): 9771–9777. https://doi.org/10.1523/JNEUROSCI.1319-09.2009
Okeefe J, Recce ML, 1993, Phase Relationship between hippocampal place units and the eeg theta-rhythm. Hippocampus, 3(3): 317–330. https://doi.org/10.1002/hipo.450030307
Buzsaki G, 2002, Theta oscillations in the hippocampus. Neuron, 33(3): 325–340. https://doi.org/10.1016/s0896-6273(02)00586-x
Qasim SE, Fried I, Jacobs J, 2021, Phase precession in the human hippocampus and entorhinal corex. Cell, 184(12): 3242–3255.e3210. https://doi.org/10.1016/j.cell.2021.04.017
Harris MA, Wolbers T, 2012, Ageing effects on path integration and landmark navigation. Hippocampus, 22(8): 1770–1780. https://doi.org/10.1002/hipo.22011
Mahmood O, Adamo D, Briceno E, et al., 2009, Age differences in visual path integration. Behav Brain Res, 205(1): 88–95. https://doi.org/10.1016/j.bbr.2009.08.001
Korman M, Weiss PL, Hochhauser M, et al., 2019, Effect of age on spatial memory performance in real museum vs. computer simulation. BMC Geriatr, 19(1): 165. https://doi.org/10.1186/s12877-019-1167-2
Head D, Isom M, 2010, Age effects on wayfinding and route learning skills. Behav Brain Res, 209(1): 49–58. https://doi.org/10.1016/j.bbr.2010.01.012
Bryden KJ, Charlton JL, Oxley JA, et al., 2013, Self-reported wayfinding ability of older drivers. Accid Anal Prev, 59: 277–282. https://doi.org/10.1016/j.aap.2013.06.017
Rodgers MK, Sindone JA 3rd, Moffat SD, 2012, Effects of age on navigation strategy. Neurobiol Aging, 33(1): e215–e222. https://doi.org/10.1016/j.neurobiolaging.2010.07.021
Harris MA, Wiener JM, Wolbers T, 2012, Aging specifically impairs switching to an allocentric navigational strategy. Front Aging Neurosci, 4: 29. https://doi.org/10.3389/fnagi.2012.00029
Harris MA, Wolbers T, 2014, How age-related strategy switching deficits affect wayfinding in complex environments. Neurobiol Aging, 35(5): 1095–1102. https://doi.org/10.1016/j.neurobiolaging.2013.10.086
Carelli L, Rusconi ML, Scarabelli C, et al., 2011, The transfer from survey (map-like) to route representations into virtual reality mazes: Effect of age and cerebral lesion. J Neuroeng Rehabil, 8: 6. https://doi.org/10.1186/1743-0003-8-6
Moffat SD, Kennedy KM, Rodrigue KM, et al., 2007, Extrahippocampal contributions to age differences in human spatial navigation. Cereb Cortex, 17(6): 1274–1282. https://doi.org/10.1093/cercor/bhl036
Driscoll I, Davatzikos C, An Y, et al., 2009, Longitudinal pattern of regional brain volume change differentiates normal aging from MCI. Neurology, 72(22): 1906–1913. https://doi.org/10.1212/WNL.0b013e3181a82634
Kerbler GM, Nedelska Z, Fripp J, et al., 2015, Basal forebrain atrophy contributes to allocentric navigation impairment in Alzheimer’s disease patients. Front Aging Neurosci, 7: 185. https://doi.org/10.3389/fnagi.2015.00185
Lovden M, Schaefer S, Noack H, et al., 2012, Spatial navigation training protects the hippocampus against age-related changes during early and late adulthood. Neurobiol Aging, 33(3): 620 e629–620 e622. https://doi.org/10.1016/j.neurobiolaging.2011.02.013
Colombo D, Serino S, Tuena C, et al., 2017, Egocentric and allocentric spatial reference frames in aging: A systematic review. Neurosci Biobehav Rev, 80: 605–621. https://doi.org/10.1016/j.neubiorev.2017.07.012
Konishi K, Etchamendy N, Roy S, et al., 2013, Decreased functional magnetic resonance imaging activity in the hippocampus in favor of the caudate nucleus in older adults tested in a virtual navigation task. Hippocampus, 23(11): 1005–1014. https://doi.org/10.1002/hipo.22181
Moffat SD, Elkins W, Resnick SM, 2006, Age differences in the neural systems supporting human allocentric spatial navigation. Neurobiol Aging, 27(7): 965–972. https://doi.org/10.1016/j.neurobiolaging.2005.05.011
Antonova E, Parslow D, Brammer M, et al., 2009, Age-related neural activity during allocentric spatial memory. Memory (Hove, England), 17(2): 125–143. https://doi.org/10.1080/09658210802077348
Jheng SS, Pai MC, 2009, Cognitive map in patients with mild Alzheimer’s disease: A computer-generated arena study. Behav Brain Res, 200(1): 42–47. https://doi.org/10.1016/j.bbr.2008.12.029
Zanco M, Plácido J, Marinho V, et al., 2018, Spatial navigation in the elderly with Alzheimer’s disease: A cross-sectional study. J Alzheimers Dis, 66(4): 1683–1694. https://doi.org/10.3233/jad-180819
Pai MC, Jan SS, 2020, Have i been here? Sense of location in people with Alzheimer’s disease. Front Aging Neurosci, 12: 582525. https://doi.org/10.3389/fnagi.2020.582525
Morganti F, Stefanini S, Riva G, 2013, From allo-to egocentric spatial ability in early Alzheimer’s disease: A study with virtual reality spatial tasks. Cogn Neurosci, 4(3–4): 171–180. https://doi.org/10.1080/17588928.2013.854762
Serino S, Morganti F, Di Stefano F, et al., 2015, Detecting early egocentric and allocentric impairments deficits in Alzheimer’s disease: An experimental study with virtual reality. Front Aging Neurosci, 7: 88. https://doi.org/10.3389/fnagi.2015.00088
Parizkova M, Lerch O, Moffat SD, et al., 2018, The effect of Alzheimer’s disease on spatial navigation strategies. Neurobiol Aging, 64: 107–115. https://doi.org/10.1016/j.neurobiolaging.2017.12.019
Plácido J, Ferreira JV, Araújo J, et al., 2021, Beyond the mini-mental state examination: The use of physical and spatial navigation tests to help to screen for mild cognitive impairment and Alzheimer’s disease. J Alzheimers Dis, 81(3): 1243–1252. https://doi.org/10.3233/jad-210106
Ghosh A, Puthusseryppady V, Chan D, et al., 2022, Machine learning detects altered spatial navigation features in outdoor behaviour of Alzheimer’s disease patients. Sci Rep, 12(1): 3160. https://doi.org/10.1038/s41598-022-06899-w
Hao X, Huang Y, Li X, et al., 2016, Structural and functional neural correlates of spatial navigation: A combined voxel-based morphometry and functional connectivity study. Brain Behav, 6(12): e00572. https://doi.org/10.1002/brb3.572
Chadwick MJ, Jolly AE, Amos DP, et al., 2015, A goal direction signal in the human entorhinal/subicular region. Curr Biol, 25(1): 87–92. https://doi.org/10.1016/j.cub.2014.11.001
Hirshhorn M, Grady C, Rosenbaum RS, et al., 2012, The hippocampus is involved in mental navigation for a recently learned, but not a highly familiar environment: A longitudinal fMRI study. Hippocampus, 22(4): 842–852. https://doi.org/10.1002/hipo.20944
Viard A, Doeller CF, Hartley T, et al., 2011, Anterior hippocampus and goal-directed spatial decision making. J Neurosci, 31(12): 4613–4621. https://doi.org/10.1523/jneurosci.4640-10.2011
Xu J, Evensmoen HR, Lehn H, et al., 2010, Persistent posterior and transient anterior medial temporal lobe activity during navigation. Neuroimage, 52(4): 1654–1666. https://doi.org/10.1016/j.neuroimage.2010.05.074
Ramanoël S, York E, Le Petit M, et al., 2019, Age-related differences in functional and structural connectivity in the spatial navigation brain network. Front Neural Circuits, 13: 69. https://doi.org/10.3389/fncir.2019.00069
Boccia M, Sulpizio V, Nemmi F, et al., 2017, Direct and indirect parieto-medial temporal pathways for spatial navigation in humans: Evidence from resting-state functional connectivity. Brain Struct Funct, 222(4): 1945–1957. https://doi.org/10.1007/s00429-016-1318-6
Hao X, Wang X, Song Y, et al., 2018, Dual roles of the hippocampus and intraparietal sulcus in network integration and segregation support scene recognition. Brain Struct Funct, 223(3): 1473–1485.https://doi.org/10.1007/s00429-017-1564-2
Sulpizio V, Boccia M, Guariglia C, et al., 2016, Functional connectivity between posterior hippocampus and retrosplenial complex predicts individual differences in navigational ability. Hippocampus, 26(7): 841–847. https://doi.org/10.1002/hipo.22592
Izen SC, Chrastil ER, Stern CE, 2018, Resting state connectivity between medial temporal lobe regions and intrinsic cortical networks predicts performance in a path integration task. Front Hum Neurosci, 12: 415. https://doi.org/10.3389/fnhum.2018.00415
Zajac L, Burte H, Taylor HA, et al., 2019, Self-reported navigation ability is associated with optic flow-sensitive regions’ functional connectivity patterns during visual path integration. Brain Behav, 9(4): e01236. https://doi.org/10.1002/brb3.1236
Irish M, Halena S, Kamminga J, et al., 2015, Scene construction impairments in Alzheimer’s disease a unique role for the posterior cingulate cortex. Cortex, 73: 10–23. https://doi.org/10.1016/j.cortex.2015.08.004
Mesulam MM, Mufson EJ, Levey AI, et al., 1983, Cholinergic innervation of cortex by the basal forebrain: Cytochemistry and cortical connections of the septal area, diagonal band nuclei, nucleus basalis (substantia innominata), and hypothalamus in the rhesus monkey. J Comp Neurol, 214(2): 170–197. https://doi.org/10.1002/cne.902140206
Pengas G, Williams GB, Acosta-Cabronero J, et al., 2012, The relationship of topographical memory performance to regional neurodegeneration in Alzheimer’s disease. Front Aging Neurosci, 4: 17. https://doi.org/10.3389/fnagi.2012.00017
Csukly G, Sirály E, Fodor Z, et al., 2016, The differentiation of amnestic type MCI from the non-amnestic types by structural MRI. Front Aging Neurosci, 8: 52–52. https://doi.org/10.3389/fnagi.2016.00052
Qing Z, Li W, Nedelska Z, et al., 2017, Spatial navigation impairment is associated with alterations in subcortical intrinsic activity in mild cognitive impairment: A resting-state fMRI Study. Behav Neurol, 2017: 6364314. https://doi.org/10.1155/2017/6364314
Li W, Zhao H, Qing Z, et al., 2021, Disrupted network topology contributed to spatial navigation impairment in patients with mild cognitive impairment. Front Aging Neurosci, 13: 630677. https://doi.org/10.3389/fnagi.2021.630677
Keynejad RC, Marková H, Šiffelová K, et al., 2018, Spatial navigation deficits in amnestic mild cognitive impairment with neuropsychiatric comorbidity. Neuropsychol Deve Cogn B Aging, Neuropsychol Cogn, 25(2): 277–289. https://doi.org/10.1080/13825585.2017.1290212
Boccia M, Di Vita A, Diana S, et al., 2019, Is losing one’s way a sign of cognitive decay? Topographical memory deficit as an early marker of pathological aging. J Alzheimers Dis, 68(2): 679–693. https://doi.org/10.3233/jad-180890
Hort J, Laczó J, Vyhnálek M, et al., 2007, Spatial navigation deficit in amnestic mild cognitive impairment. Proc Natl Acad Sci U S A, 104(10): 4042–4047. https://doi.org/10.1073/pnas.0611314104
Rusconi ML, Suardi A, Zanetti M, et al., 2015, Spatial navigation in elderly healthy subjects, amnestic and non amnestic MCI patients. J Neurol Sci, 359(1-2): 430–437. https://doi.org/10.1016/j.jns.2015.10.010
Laczó J, Vlcek K, Vyhnálek M, et al., 2009, Spatial navigation testing discriminates two types of amnestic mild cognitive impairment. Behav Brain Res, 202(2): 252–259. https://doi.org/10.1016/j.bbr.2009.03.041
Laczó J, Andel R, Vyhnalek M, et al., 2012, From morris water maze to computer tests in the prediction of Alzheimer’s disease. Neurodegener Dis, 10(1-4): 153–157. https://doi.org/10.1159/000333121
Wood RA, Moodley KK, Lever C, et al., 2016, Allocentric spatial memory testing predicts conversion from mild cognitive impairment to dementia: An initial proof-of-concept study. Front Neurol, 7: 215. https://doi.org/10.3389/fneur.2016.00215
Vlček K, Laczó J, 2014, Neural correlates of spatial navigation changes in mild cognitive impairment and Alzheimer’s disease. Front Behav Neurosci, 8: 89. https://doi.org/10.3389/fnbeh.2014.00089
Hallab A, Lange C, Apostolova I, et al., 2020, Impairment of everyday spatial navigation abilities in mild cognitive impairment is weakly associated with reduced grey matter volume in the medial part of the entorhinal cortex. J Alzheimers Dis, 78(3): 1149–1159. https://doi.org/10.3233/jad-200520
Weniger G, Ruhleder M, Lange C, et al., 2011, Egocentric and allocentric memory as assessed by virtual reality in individuals with amnestic mild cognitive impairment. Neuropsychologia, 49(3): 518–527. https://doi.org/10.1016/j.neuropsychologia.2010.12.031
Mokrisova I, Laczo J, Andel R, et al., 2016, Real-space path integration is impaired in Alzheimer’s disease and mild cognitive impairment. Behav Brain Res, 307: 150–158. https://doi.org/10.1016/j.bbr.2016.03.052
Laczó J, Andel R, Nedelska Z, et al., 2017, Exploring the contribution of spatial navigation to cognitive functioning in older adults. Neurobiol Aging, 51: 67–70. https://doi.org/10.1016/j.neurobiolaging.2016.12.003
Peter J, Sandkamp R, Minkova L, et al., 2018, Real-world navigation in amnestic mild cognitive impairment: The relation to visuospatial memory and volume of hippocampal subregions. Neuropsychologia, 109: 86–94. https://doi.org/10.1016/j.neuropsychologia.2017.12.014
Laczó J, Andel R, Vyhnalek M, et al., 2014, APOE and spatial navigation in amnestic MCI: Results from a computer-based test. Neuropsychology, 28(5): 676–684. https://doi.org/10.1037/neu0000072
Laczó J, Andel R, Vyhnalek M, et al., 2015, The effect of TOMM40 on spatial navigation in amnestic mild cognitive impairment. Neurobiol Aging, 36(6): 2024–2033. https://doi.org/10.1016/j.neurobiolaging.2015.03.004
Laczó J, Andel R, Vlček K, et al., 2011, Spatial navigation and APOE in amnestic mild cognitive impairment. Neurodegener Dis, 8(4): 169–177. https://doi.org/10.1159/000321581
Xu WL, Caracciolo B, Wang HX, et al., 2013, Accelerated progression from mild cognitive impairment to dementia among APOE epsilon4epsilon4 carriers. J Alzheimers Dis, 33(2): 507–515. https://doi.org/10.3233/JAD-2012-121369
Laczó J, Cechova K, Parizkova M, et al., 2020, The combined effect of APOE and BDNF Val66Met polymorphisms on spatial navigation in older adults. J Alzheimers Dis, 78(4): 1473–1492. https://doi.org/10.3233/jad-200615
Migo EM, O’Daly O, Mitterschiffthaler M, et al., 2016, Investigating virtual reality navigation in amnestic mild cognitive impairment using fMRI. Neuropsychol Dev Cogn B Aging Neuropsychol Cogn, 23(2): 196–217. https://doi.org/10.1080/13825585.2015.1073218
Jessen F, Amariglio RE, van Boxtel M, et al., 2014, A conceptual framework for research on subjective cognitive decline in preclinical Alzheimer’s disease. Alzheimers Dement, 10(6): 844–852. https://doi.org/10.1016/j.jalz.2014.01.001
Jessen F, Amariglio RE, Buckley RF, et al., 2020, The characterisation of subjective cognitive decline. Lancet Neurol, 19(3): 271–278. https://doi.org/10.1016/s1474-4422(19)30368-0
Hu X, Teunissen CE, Spottke A, et al., 2019, Smaller medial temporal lobe volumes in individuals with subjective cognitive decline and biomarker evidence of Alzheimer’s disease-data from three memory clinic studies. Alzheimers Dement, 15(2): 185–193. https://doi.org/10.1016/j.jalz.2018.09.002
Cerman J, Andel R, Laczo J, et al., 2018, Subjective spatial navigation complaints a frequent symptom reported by patients with subjective cognitive decline, mild cognitive impairment and Alzheimers disease. Curr Alzheimer Res, 15(3): 219–228. https://doi.org/10.2174/1567205014666171120145349
Chen Q, Qing Z, Jin J, et al., 2021, Ego and allo-network disconnection underlying spatial disorientation in subjective cognitive decline. Cortex, 137: 35–48. https://doi.org/10.1016/j.cortex.2020.12.022
Chen Q, Wu S, Li X, et al., 2021, Basal forebrain atrophy is associated with allocentric navigation deficits in subjective cognitive decline. Front Aging Neurosci, 13: 596025. https://doi.org/10.3389/fnagi.2021.596025
Tangen GG, Engedal K, Bergland A, et al., 2015, Spatial navigation measured by the floor maze test in patients with subjective cognitive impairment, mild cognitive impairment, and mild Alzheimer’s disease. Int Psychogeriatr, 27(8): 1401–1409. https://doi.org/10.1017/s1041610215000022
Berteau-Pavy F, Park B, Raber J, 2007, Effects of sex and APOE epsilon4 on object recognition and spatial navigation in the elderly. Neuroscience, 147(1): 6–17. https://doi.org/10.1016/j.neuroscience.2007.03.005
Coughlan G, Coutrot A, Khondoker M, et al., 2019, Toward personalized cognitive diagnostics of at-genetic-risk Alzheimer’s disease. Proc Natl Acad Sci U S A, 116(19): 9285–9292. https://doi.org/10.1073/pnas.1901600116
Coughlan G, Zhukovsky P, Puthusseryppady V, et al., 2020, Functional connectivity between the entorhinal and posterior cingulate cortices underpins navigation discrepancies in at-risk Alzheimer’s disease. Neurobiol Aging, 90: 110–118. https://doi.org/10.1016/j.neurobiolaging.2020.02.007
Lerch O, Laczó M, Vyhnálek M, et al., 2022, APOEɛ4 allele moderates the association between basal forebrain nuclei volumes and allocentric navigation in older adults without dementia. J Alzheimers Dis, 86(1): 155–171. https://doi.org/10.3233/jad-215034
Serrano-Pozo A, Qian J, Monsell SE, et al., 2015, APOEepsilon2 is associated with milder clinical and pathological Alzheimer disease. Ann Neurol, 77(6): 917–929. https://doi.org/10.1002/ana.24369
Konishi K, Bhat V, Banner H, et al., 2016, APOE2 Is associated with spatial navigational strategies and increased gray matter in the hippocampus. Front Hum Neurosci, 10: 349. https://doi.org/10.3389/fnhum.2016.00349
Allison SL, Fagan AM, Morris JC, et al., 2016, Spatial navigation in preclinical Alzheimer’s disease. J Alzheimers Dis, 52(1): 77–90. https://doi.org/10.3233/jad-150855
Verghese J, Lipton R, Ayers E, 2017, Spatial navigation and risk of cognitive impairment: A prospective cohort study. Alzheimers Dement, 13(9): 985–992. https://doi.org/10.1016/j.jalz.2017.01.023
Levine TF, Allison SL, Stojanovic M, et al., 2020, Spatial navigation ability predicts progression of dementia symptomatology. Alzheimers Dement, 16(3): 491–500. https://doi.org/10.1002/alz.12031
Hu W, Zhang X, Tung YC, et al., 2016, Hyperphosphorylation determines both the spread and the morphology of tau pathology. Alzheimers Dement, 12(10): 1066–1077. https://doi.org/10.1016/j.jalz.2016.01.014
Li Y, Xu J, Liu Y, et al., 2017, A distinct entorhinal cortex to hippocampal CA1 direct circuit for olfactory associative learning. Nat Neurosc, 20(4): 559–570. https://doi.org/10.1038/nn.4517
Leigh A. Holcomb MN, Jantzen P, et al., 1999, Behavioral changes in transgenic mice expressing both amyloid precursor protein and presenilin-1 mutations: Lack of association with amyloid deposits. Behav Genet, 29(3): 177–185. https://doi.org/10.1023/a:1021691918517
Puoliväli J, Wang J, Heikkinen T, et al., 2002, Hippocampal A beta 42 levels correlate with spatial memory deficit in APP and PS1 double transgenic mice. Neurobiol Dis, 9(3): 339–347. https://doi.org/10.1006/nbdi.2002.0481
Allison S, Babulal GM, Stout SH, et al., 2018, Alzheimer disease biomarkers and driving in clinically normal older adults role of spatial navigation abilities. Alzheimer Dis Assoc Disord, 32: 101–106. https://doi.org/10.1097/WAD.0000000000000257
Fu H, Rodriguez GA, Herman M, et al., 2017, Tau pathology induces excitatory neuron loss, grid cell dysfunction, and spatial memory deficits reminiscent of early Alzheimer’s Disease. Neuron, 93(3): 533–541. https://doi.org/10.1016/j.neuron.2016.12.023
Stimmell AC, Xu Z, Moseley SC, et al., 2021, Tau pathology profile across a parietal-hippocampal brain network is associated with spatial reorientation learning and memory performance in the 3xTg-AD mouse. Front Aging, 2: 655015. https://doi.org/10.3389/fragi.2021.655015
Stancu IC, Ris L, Vasconcelos B, et al., 2014, Tauopathy contributes to synaptic and cognitive deficits in a murine model for Alzheimer’s disease. FASEB J, 28(6): 2620–2631. https://doi.org/10.1096/fj.13-246702
Killian NJ, Jutras MJ, Buffalo EA, 2012, A map of visual space in the primate entorhinal cortex. Nature, 491(7426): 761–764. https://doi.org/10.1038/nature11587
Rowland DC, Roudi Y, Moser MB, et al., 2016, Ten years of grid cells. Annu Rev Neurosci, 39: 19–40. https://doi.org/10.1146/annurev-neuro-070815-013824
McNaughton BL, Battaglia FP, Jensen O, et al., 2006, Path integration and the neural basis of the “cognitive map”. Nat Rev Neurosci, 7(8): 663–678. https://doi.org/10.1038/nrn1932
Van Wijngaarden JB, Babl SS, Ito HT, 2020, Entorhinal-retrosplenial circuits for allocentric-egocentric transformation of boundary coding. Life, 9: e59816. https://doi.org/10.7554/eLife.59816
Sargolini F, Fyhn M, Hafting T, et al., 2006, Conjunctive representation of position, direction, and velocity in entorhinal cortex. Science, 312(5774): 758–762. https://doi.org/10.1126/science.1125572
Kunz L, Lee H, Montag C, et al., 2015, Reduced grid-cell-like representations in adults at genetic risk forAlzheimer’s disease. Science, 350(6259): 430–433. https://doi.org/10.1126/science.aac8128
Bates SL, Wolbers T, 2014, How cognitive aging affects multisensory integration of navigational cues. Neurobiol Aging, 35(12): 2761–2769. https://doi.org/10.1016/j.neurobiolaging.2014.04.003
Muessig L, Hauser J, Wills TJ, et al., 2015, A developmental switch in place cell accuracy coincides with grid cell maturation. Neuron, 86(5): 1167–1173. https://doi.org/10.1016/j.neuron.2015.05.011
Reifenstein ET, Kempter R, Schreiber S, et al., 2012, Grid cells in rat entorhinal cortex encode physical space with independent firing fields and phase precession at the single-trial level. Proc Natl Acad Sci U S A, 109(16): 6301–6306. https://doi.org/10.1073/pnas.1109599109
Sakkaki S, Barrière S, Bender AC, et al., 2020, Focal dorsal hippocampal nav1.1 knock down alters place cell temporal coordination and spatial behavior. Cereb Cortex, 30(9): 5049–5066. https://doi.org/10.1093/cercor/bhaa101
Moreno A, Wall KJ, Thangavelu K, et al., 2019, A systematic review of the use of virtual reality and its effects on cognition in individuals with neurocognitive disorders. Alzheimers Dement (NY), 5: 834–850. https://doi.org/10.1016/j.trci.2019.09.016
Oliveira J, Gamito P, Souto T, et al., 2021, Virtual reality-based cognitive stimulation on people with mild to moderate dementia due to Alzheimer’s disease: A pilot randomized controlled trial. Int J Environ Res Public Health, 18(10): 5290. https://doi.org/10.3390/ijerph18105290
Micarelli A, Viziano A, Micarelli B, et al., 2019, Vestibular rehabilitation in older adults with and without mild cognitive impairment: Effects of virtual reality using a head-mounted display. Arch Gerontol Geriatr, 83: 246–256. https://doi.org/10.1016/j.archger.2019.05.008
Hwang J, Lee S, 2017, The effect of virtual reality program on the cognitive function and balance of the people with mild cognitive impairment. J Phys Ther Sci, 29(8):1283–1286. https://doi.org/10.1589/jpts.29.1283
Kober GW, Hofer D, Kreuzig W, et al., 2013, Virtual reality in neurologic rehabilitation of spatial disorientation. J NeuroEng Rehabil, 10(1): 17.
Serino S, Pedroli E, Tuena C, et al., 2017, A novel virtual reality-based training protocol for the enhancement of the “Mental frame syncing” in individuals with Alzheimer’s disease: A development-of-concept trial. Front Aging Neurosci, 9: 240. https://doi.org/10.3389/fnagi.2017.00240
White PJ, Moussavi Z, 2016, Neurocognitive treatment for a patient with Alzheimer’s disease using a virtual reality navigational environment. J Exp Neurosci, 10: 129–135. https://doi.org/10.4137/JEN.S40827
Oliveira LM, Evangelista ES, Alves MR, et al., 2020, 2D virtual reality-based exercise improves spatial navigation in institutionalized non-robust older persons: A preliminary data report of a single-blind, randomized, and controlled study. Front Neurol, 11: 609988. https://doi.org/10.3389/fneur.2020.609988
Aaronson ST, Philip NS, 2018, Neuromodulation. Psychiatr Clin North Am, 41(3): 13–16. https://doi.org/10.1016/j.psc.2018.06.001
Deeb W, Salvato B, Almeida L, et al., 2019, Fornix-region deep brain stimulation induced memory flashbacks in Alzheimer’s disease. N Engl J Med, 381(8): 783–785. https://doi.org/10.1056/NEJMc1905240
Yang Z, Sheng X, Qin R, et al., 2022, Cognitive improvement via left angular gyrus-navigated repetitive transcranial magnetic stimulation inducing the neuroplasticity of thalamic system in amnesic mild cognitive impairment patients. J Alzheimers Dis, 86(2): 537–551. https://doi.org/10.3233/JAD-215390
Manenti R, Sandrini M, Gobbi E, et al., 2017, Strengthening of existing episodic memories through non-invasive stimulation of prefrontal cortex in older adults with subjective memory complaints. Front Aging Neurosci, 9: 401. https://doi.org/10.3389/fnagi.2017.00401
Jeong H, Im JJ, Park JS, et al., 2021, A pilot clinical study of low-intensity transcranial focused ultrasound in Alzheimer’s disease. Ultrasonography, 40(4): 512–519. https://doi.org/10.14366/usg.20138
Fyock C, England HB, Hampstead BM, et al., 2015, Transcranial direct current stimulation modulates spatial memory in cognitively intact adults. Behav Brain Res Int J. 283:191–195. https://doi.org/10.1016/j.bbr.2015.01.044
Krishnamurthy V, Gopinath K, Brown GS, et al., 2015, Resting-state fMRI reveals enhanced functional connectivity in spatial navigation networks after transcranial direct current stimulation. Neurosci Lett, 604: 80–85. https://doi.org/10.1016/j.neulet.2015.07.042
Iordan AD, Ryan S, Tyszkowski T, et al., 2022, High-definition transcranial direct current stimulation enhances network segregation during spatial navigation in mild cognitive impairment. Cereb Cortex, 00: 1–12. https://doi.org/10.1093/cercor/bhac010
Suthana N, Haneef Z, Stern J, et al., 2012, Memory enhancement and deep-brain stimulation of the entorhinal area. N Engl J Med, 366(6): 502–510. https://doi.org/10.1056/NEJMoa1107212
Laczó J, Markova H, Lobellova V, et al., 2017, Scopolamine disrupts place navigation in rats and humans: A translational validation of the hidden goal task in the morris water maze and a real maze for humans. Psychopharmacology, 234(4): 535–547. https://doi.org/10.1007/s00213-016-4488-2
Poos JM, van der Ham IJM, Leeuwis AE, et al., 2021, Short digital spatial memory test detects impairment in Alzheimer’s disease and mild cognitive impairment. Brain Sci, 11(10): 1350. https://doi.org/10.3390/brainsci11101350
Laczó M, Wiener JM, Kalinova J, et al., 2021, Spatial navigation and visuospatial strategies in typical and atypical aging. Brain Sci, 11(11): 1421. https://doi.org/10.3390/brainsci11111421
Da Costa RQ, Pompeu JE, Moretto E, et al., 2021, Two immersive virtual reality tasks for the assessment of spatial orientation in older adults with and without cognitive impairment: Concurrent validity, group comparison, and accuracy results. J Int Neuropsychol Soc, 28(5): 460–472. https://doi.org/10.1017/s1355617721000655
Puthusseryppady V, Emrich-Mills L, Lowry E, et al., 2020, Spatial disorientation in Alzheimer’s disease: The missing path from virtual reality to real world. Front Aging Neurosci, 12: 550514. https://doi.org/10.3389/fnagi.2020.550514