Impact behavior of AlSi10Mg porous structures with varying single-unit cell rotation angles fabricated via laser powder bed fusion
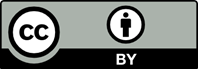
Porous structures offer lightweight design and geometric flexibility for applications in transportation and bioengineering. Additive manufacturing, particularly laser powder bed fusion (LPBF), enables the fabrication of complex porous architectures. However, achieving an optimal balance between weight reduction and mechanical performance remains challenging. Therefore, further investigation into the design of porous structures is essential. This study explores the dynamic mechanical behavior of porous AlSi10Mg structures designed using a parametric modeling approach and the Voronoi tessellation algorithm. The structures, fabricated via LPBF, feature varying single-unit cell rotation angles and porosities. The dynamic mechanical behaviors were experimentally investigated under different impact energies to assess the influence of single-unit cell rotation on impact properties, complemented by finite element analysis simulations. The results indicate that a slight decrease in porosity by 10% (from 90% to 80%) significantly enhances energy absorption and impact resistance while maintaining lightweight features. Significant variations are observed in peak contact force and energy absorption trends. The results demonstrate that single-unit cell rotation improves impact resistance in certain cases, leading to significant enhancements in energy absorption, specific energy absorption, and specific strength, which increased by approximately 18.9% (P90), 17.1% (P90), and 79.5% (P80), respectively, for the dodecahedral (Dodeca)-C structure compared to the original Dodeca-A counterpart at impact of 124 J. In addition, Dodeca-C P80 showed a remarkable 73.1% increase in energy absorption compared to Dodeca-A P80 at a higher impact energy of 248 J. This study provides insights for optimizing porous structures while maintaining consistent unit cell configurations and identical porosity, with rotating unit cell angles enhancing impact resistance.

- Babaei M, Kiarasi F, Asemi K, Hosseini M. Functionally graded saturated porous structures: A review. J Comput Appl Mech. 2022;53:297-308. doi: 10.22059/jcamech.2022.342710.719
- Wu ZY, Liu YJ, Wu X, Liu XC, Wang JC, Wang Q. Fatigue performance of beta titanium alloy topological porous structures fabricated by laser powder bed fusion. J Mater Res Technol. 2024;29:4772-4780. doi: 10.1016/j.jmrt.2024.02.190
- Siddique SH, Hazell PJ, Wang H, Escobedo JP, Ameri AAH. Lessons from nature: 3D printed bio-inspired porous structures for impact energy absorption-A review. Addit Manuf. 2022;58:103051. doi: 10.1016/j.addma.2022.103051
- Wu ZY, Liu YJ, Bai HW, et al. Microstructure and mechanical behavior of rhombic dodecahedron-structured porous β-Ti composites fabricated via laser powder bed fusion. J Mater Res Technol. 2024;31:298-310. doi: 10.1016/j.jmrt.2024.06.077
- Wang B, Luo M, Shi Z, et al. Porous titanium alloys for medical application: Progress in preparation process and surface modification research. MSAM. 2024;3:2753. doi: 10.36922/msam.2753
- Dul’nev GN, Volkov DP, Malarev VI. Thermal conductivity of moist porous materials. J Eng Phys. 1989;56:198-206. doi: 10.1007/BF00870578
- Yin H, Zhang W, Zhu L, Meng F, Liu J, Wen G. Review on lattice structures for energy absorption properties. Compos Struct. 2023;304:116397. doi: 10.1016/j.compstruct.2022.116397
- Xu K, Cao J, Zheng Z, et al. Deformation behavior of inconel 625 alloy with TPMS structure. Materials (Basel). 2025;18:396. doi: 10.3390/ma18020396
- Lu S, Zhang M, Guo S, Hur B, Yue X. Numerical investigation of impact behavior of strut-based cellular structures designed by spatial voronoi tessellation. Metals. 2022;12:1189. doi: 10.3390/met12071189
- Ha NS, Pham TM, Hao H, Lu G. Energy absorption characteristics of bio-inspired hierarchical multi-cell square tubes under axial crushing. Int J Mech Sci. 2021;201:106464. doi: 10.1016/j.ijmecsci.2021.106464
- Ha NS, Pham TM, Tran TT, Hao H, Lu G. Mechanical properties and energy absorption of bio-inspired hierarchical circular honeycomb. Compos Part B Eng. 2022;236:109818. doi: 10.1016/j.compositesb.2022.109818
- Zhang L, Feih S, Daynes S, et al. Energy absorption characteristics of metallic triply periodic minimal surface sheet structures under compressive loading. Addit Manuf. 2018;23:505-515. doi: 10.1016/j.addma.2018.08.007
- Zhao M, Zhang DZ, Liu F, Li Z, Ma Z, Ren Z. Mechanical and energy absorption characteristics of additively manufactured functionally graded sheet lattice structures with minimal surfaces. Int J Mech Sci. 2020;167:105262. doi: 10.1016/j.ijmecsci.2019.105262
- Hu D, Mei X, Shi P, Zhou X. Inner-pipe structure to improve column heterogeneity and peak shape. J Chromatogr Sci. 2014;53:565-570. doi: 10.1093/chromsci/bmu085
- Liu YJ, Zhang ZL, Wang JC, et al. In-situ micro-CT analysis of deformation behavior in sandwich-structured meta-stable beta Ti-35Nb alloy. Trans Nonferrous Met Soc China. 2024;34:2552-2562. doi: 10.1016/S1003-6326(24)66559-3
- Wang Z. Recent advances in novel metallic honeycomb structure. Compos Part B Eng. 2019;166:731-741. doi: 10.1016/j.compositesb.2019.02.011
- Song ZL, Ma LQ, Wu ZJ, He DP. Effects of viscosity on cellular structure of foamed aluminum in foaming process. J Mater Sci. 2000;35:15-20. doi: 10.1023/A:1004715926692
- Zhang M, Bi G, Chen J. Research on impact resistance of AlSi7Mg uniform and gradient porous structures manufactured by laser powder bed fusion. MSAM. 2024;3:5729. doi: 10.36922/msam.5729
- Al-Saedi DSJ, Masood SH, Faizan-Ur-Rab M, Alomarah A, Ponnusamy P. Mechanical properties and energy absorption capability of functionally graded F2BCC lattice fabricated by SLM. Mater Des. 2018;144:32-44. doi: 10.1016/j.matdes.2018.01.059
- Liu YJ, Zhang JS, Liu XC, et al. Non-layer-wise fracture and deformation mechanism in beta titanium cubic lattice structure manufactured by selective laser melting. Mater Sci Eng A. 2021;822:141696. doi: 10.1016/j.msea.2021.141696
- Şerban DA, Negru R, Sărăndan S, Belgiu G, Marşavina L. Numerical and experimental investigations on the mechanical properties of cellular structures with open Kelvin cells. Mech Adv Mater Struct. 2021;28:1367-1376. doi: 10.1080/15376494.2019.1669093
- Lovinger Z, Czarnota C, Ravindran S, Molinari A, Ravichandran G. The role of micro-inertia on the shock structure in porous metals. J Mech Phys Solids. 2021;154:104508. doi: 10.1016/j.jmps.2021.104508
- Czarnota C, Molinari A, Mercier S. Steady shock waves in porous metals: Viscosity and micro-inertia effects. Int J Plast. 2020;135:102816. doi: 10.1016/j.ijplas.2020.102816
- Fíla T, Koudelka P, Falta J, et al. Dynamic impact testing of cellular solids and lattice structures: Application of two-sided direct impact Hopkinson bar. Int J Impact Eng. 2021;148:103767. doi: 10.1016/j.ijimpeng.2020.103767
- Jin N, Wang F, Wang Y, Zhang B, Cheng H, Zhang H. Failure and energy absorption characteristics of four lattice structures under dynamic loading. Mater Des. 2019;169:107655. doi: 10.1016/j.matdes.2019.107655
- Cao X, Duan S, Liang J, Wen W, Fang D. Mechanical properties of an improved 3D-printed rhombic dodecahedron stainless steel lattice structure of variable cross section. Int J Mech Sci. 2018;145:53-63. doi: 10.1016/j.ijmecsci.2018.07.006
- Kim JH, Kim D, Lee MG, Lee JK. Multiscale analysis of open-cell aluminum foam for impact energy absorption. J Mater Eng Perform. 2016;25:3977-3984. doi: 10.1007/s11665-016-2187-0
- Tancogne-Dejean T, Spierings AB, Mohr D. Additively-manufactured metallic micro-lattice materials for high specific energy absorption under static and dynamic loading. Acta Mater. 2016;116:14-28. doi: 10.1016/j.actamat.2016.05.054
- Wang JC, Liu YJ, Rabadia CD, Liang SX, Sercombe TB, Zhang LC. Microstructural homogeneity and mechanical behavior of a selective laser melted Ti-35Nb alloy produced from an elemental powder mixture. J Mater Sci Technol. 2021;61:221-233. doi: 10.1016/j.jmst.2020.05.052
- Zheng Z, Qiu S, Yue X, Wang J, Hou J. Detecting irradiation defects in materials: A machine learning approach to analyze helium bubble images. J Nucl Mater. 2024;596:155117. doi: 10.1016/j.jnucmat.2024.155117
- Lu WQ, Liu YJ, Wu X, Liu XC, Wang JC. Corrosion and passivation behavior of Ti-6Al-4V surfaces treated with high-energy pulsed laser: A comparative study of cast and 3D-printed specimens in a NaCl solution. Surf Coat Technol. 2023;470:129849. doi: 10.1016/j.surfcoat.2023.129849
- Wei SY, Ji LN, Wu WJ, Ma HL. Selective laser melting of lanthanum oxide-reinforced tungsten composites: Microstructure and mechanical properties. Tungsten. 2022;4:67-78. doi: 10.1007/s42864-021-00127-0
- Ma HY, Wang JC, Qin P, et al. Advances in additively manufactured titanium alloys by powder bed fusion and directed energy deposition: Microstructure, defects, and mechanical behavior. J Mater Sci Technol. 2024;183:32-62. doi: 10.1016/j.jmst.2023.11.003
- Ma HY, Wang JC, Liu YJ, et al. In-situ microstructural evolution and deformation mechanisms of metastable beta titanium fabricated by laser powder bed fusion under flexural stress. Mater Sci Eng A. 2025;925:147873. doi: 10.1016/j.msea.2025.147873
- Wang JC, Liu YJ, Liang SX, et al. Comparison of microstructure and mechanical behavior of Ti-35Nb manufactured by laser powder bed fusion from elemental powder mixture and prealloyed powder. J Mater Sci Technol. 2022;105:1-16. doi: 10.1016/j.jmst.2021.07.021
- Zhang LC, Wang JC. Stabilizing 3D-printed metal alloys. Science. 2024;383:586-587. doi: 10.1126/science.adn6566
- Lu W, Liu Y, Wu X, Liu X, Wang J. Corrosion behavior and microstructural effects on passivation film mechanisms in forged Ti-5Al-5Mo-5V-1Cr-1Fe titanium alloy under laser surface remelting. Corros Sci. 2024;241:112542. doi: 10.1016/j.corsci.2024.112542
- Wang JC, Liu YJ, Qin P, Liang SX, Sercombe TB, Zhang LC. Selective laser melting of Ti–35Nb composite from elemental powder mixture: Microstructure, mechanical behavior and corrosion behavior. Mater Sci Eng A. 2019;760:214-224. doi: 10.1016/j.msea.2019.06.001
- Nie X, Chen Z, Qi Y, Zhang H, Zhu H. Spreading behavior and hot cracking mechanism of single tracks in high strength Al–Cu–Mg–Mn Alloy fabricated by laser powder bed fusion. Acta Metall Sin (Engl Lett). 2023;36:1454-1464. doi: 10.1007/s40195-023-01553-4
- Lin S, Deng YL, Lin HQ, et al. Microstructure, mechanical properties and stress corrosion behavior of friction stir welded joint of Al–Mg–Si alloy extrusion. Rare Met. 2023;42:2057-2067. doi: 10.1007/s12598-018-1126-7
- Sun SB, Zheng LJ, Liu JH, Zhang H. Microstructure, cracking behavior and control of Al–Fe–V–Si alloy produced by selective laser melting. Rare Met. 2023;42:1353-1362. doi: 10.1007/s12598-016-0846-9
- Wang JC, Zhu R, Liu YJ, Zhang LC. Understanding melt pool characteristics in laser powder bed fusion: An overview of single and multi-track melt pools for process optimization. Adv Powder Mater. 2023;2:100137. doi: 10.1016/j.apmate.2023.100137
- Guo S, Yue X, Kitazono K. Anisotropic compression behavior of additively manufactured porous titanium with ordered open-cell structures at different temperatures. Mater Trans. 2021;62:1771-1776. doi: 10.2320/matertrans.MT-M2021149
- Bezci SE, Klineberg EO, O’Connell GD. Effects of axial compression and rotation angle on torsional mechanical properties of bovine caudal discs. J Mech Behav Biomed Mater. 2018;77:353-359. doi: 10.1016/j.jmbbm.2017.09.022
- Yang J, Gu D, Lin K, et al. Laser powder bed fusion of mechanically efficient helicoidal structure inspired by mantis shrimp. Int J Mech Sci. 2022;231:107573. doi: 10.1016/j.ijmecsci.2022.107573
- Aktürk M, Boy M, Gupta MK, Waqar S, Krolczyk GM, Korkmaz ME. Numerical and experimental investigations of built orientation dependent Johnson-Cook model for selective laser melting manufactured AlSi10Mg. J Mater Res Technol. 2021;15:6244-6259. doi: 10.1016/j.jmrt.2021.11.062
- Nurel B, Nahmany M, Frage N, Stern A, Sadot O. Split Hopkinson pressure bar tests for investigating dynamic properties of additively manufactured AlSi10Mg alloy by selective laser melting. Addit Manuf. 2018;22:823-833. doi: 10.1016/j.addma.2018.06.001
- Hou Y, Li Y, Cai X, et al. Mechanical response and response mechanism of AlSi10Mg porous structures manufactured by laser powder bed fusion: Experimental, theoretical and numerical studies. Mater Sci Eng A. 2022;849:143381. doi: 10.1016/j.msea.2022.143381
- Andrew JJ, Schneider J, Ubaid J, Velmurugan R, Gupta NK, Kumar S. Energy absorption characteristics of additively manufactured plate-lattices under low- velocity impact loading. Int J Impact Eng. 2021;149:103768. doi: 10.1016/j.ijimpeng.2020.103768
- Al-Ketan O, Rowshan R, Abu Al-Rub RK. Topology-mechanical property relationship of 3D printed strut, skeletal, and sheet based periodic metallic cellular materials. Addit Manuf. 2018;19:167-183. doi: 10.1016/j.addma.2017.12.006
- Paul MJ, Liu Q, Best JP, et al. Fracture resistance of AlSi10Mg fabricated by laser powder bed fusion. Acta Mater. 2021;211:116869. doi: 10.1016/j.actamat.2021.116869
- Peters M, Brodie EG, Thomas S, et al. On the importance of nano-oxide control in laser powder bed fusion manufactured Ni-based alloys to enhance fracture properties. Materialia. 2023;32:101958. doi: 10.1016/j.mtla.2023.101958
- Shenghao C, Xiang W, Haowei B, Yujing L, Yihao W, Jincheng W. Effects of selective laser surface treatment on plasticity of 2060 Al-Li alloy. Spec Cast Nonferrous Alloys. 2025;45:334-341. doi: 10.15980/j.tzzz.T20240066
- Wang R, Wang J, Lei LM, et al. Laser additive manufacturing of strong and ductile Al-12Si alloy under static magnetic field. J Mater Sci Technol. 2023;163:101-112. doi: 10.1016/j.jmst.2023.04.021
- Sheng X, Guo A, Guo S, et al. Laser powder bed fusion for the fabrication of triply periodic minimal surface lattice structures: Synergistic macroscopic and microscopic optimization. J Manuf Process. 2024;119:179-192. doi: 10.1016/j.jmapro.2024.03.081
- Chen R, Zheng C, Ma H, et al. Multi-parameter optimization and corrosion behavior of FeCoNiCrAl HEA coatings via laser cladding. Metals. 2025;15:406. doi: 10.3390/met15040406
- Rubben T, Revilla RI, De Graeve I. Influence of heat treatments on the corrosion mechanism of additive manufactured AlSi10Mg. Corros Sci. 2019;147:406-415. doi: 10.1016/j.corsci.2018.11.038
- Liu B, Li BQ, Li Z. Selective laser remelting of an additive layer manufacturing process on AlSi10Mg. Results Phys. 2019;12:982-988. doi: 10.1016/j.rinp.2018.12.018
- Li Z, Li Z, Tan Z, Xiong DB, Guo Q. Stress relaxation and the cellular structure-dependence of plastic deformation in additively manufactured AlSi10Mg alloys. Int J Plast. 2020;127:102640. doi: 10.1016/j.ijplas.2019.12.003
- Wang H, Fu Y, Su M, Hao H. Effect of structure design on compressive properties and energy absorption behavior of ordered porous aluminum prepared by rapid casting. Mater Des. 2019;167:107631. doi: 10.1016/j.matdes.2019.107631
- Chua C, Sing SL, Chua CK. Characterisation of in-situ alloyed titanium-tantalum lattice structures by laser powder bed fusion using finite element analysis. Virtual Phys Prototyp. 2023;18:e2138463. doi: 10.1080/17452759.2022.2138463