Phase transformations in additively manufactured high carbon-bearing steel
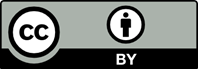
For high-carbon steels that are particularly sensitive to thermally induced phase transformations, the rapid solidification rates inherent to laser powder bed fusion (LPBF) offer a promising pathway to develop unconventional microstructures directly in the as-printed state. This study demonstrates the formation of a supersaturated austenitic matrix – engineered through carbon meta-stabilization and rapid solidification for subsequent heat treatments to develop complex, hierarchical microstructural constituents. A predominantly austenitic high-carbon steel, decorated with cellular segregation networks, was successfully fabricated using LPBF. Post-processing through cryogenic quenching and high-temperature solutionizing treatment, followed by low-temperature tempering, yielded a wide range of microstructures and hardness values. The cryogenically quenched sample exhibited a mixed microstructure of martensite, retained austenite, and cellularly segregated regions, achieving a hardness of 737 ± 31 HV. In contrast, the combination of solutionizing, cryogenic quenching, and tempering produced a multiphase matrix consisting of martensite, bainite, and austenite, with a hardness of 700 ± 20 HV. The insights gained into phase transformations and microstructural evolution during LPBF, along with secondary hardening via heat treatment, provide a foundation for developing tailored post-processing strategies for a broad class of hardenable steels produced by additive manufacturing.

- Mehta A, Zhou L, Huynh T, et al. Additive manufacturing and mechanical properties of the dense and crack free Zr-modified aluminum alloy 6061 fabricated by the laser-powder bed fusion. Addit Manuf. 2021;41:101966. doi: 10.1016/j.addma.2021.101966
- Zhou L, Hyer H, Park S, et al. Microstructure and mechanical properties of Zr-modified aluminum alloy 5083 manufactured by laser powder bed fusion. Addit Manuf. 2019;28:485-496. doi: 10.1016/j.addma.2019.05.027
- Zheng B, Zhou Y, Smugeresky J, Schoenung J, Lavernia E. Thermal behavior and microstructural evolution during laser deposition with laser-engineered net shaping: Part I. Numerical calculations. Metall Mater Trans A. 2008;39:2228-2236. doi: 10.1007/s11661-008-9557-7
- Krakhmalev P, Yadroitsava I, Fredriksson G, Yadroitsev I. In situ heat treatment in selective laser melted martensitic AISI 420 stainless steels. Mater Des. 2015;87:380-385. doi: 10.1016/j.matdes.2015.08.045
- Jelis E, Clemente M, Kerwien S, Ravindra NM, Hespos MR. Metallurgical and mechanical evaluation of 4340 steel produced by direct metal laser sintering. JOM J Miner Metals Mater Soc. 2015;67:582-589. doi: 10.1007/s11837-014-1273-8
- Shakerin S, Hadadzadeh A, Amirkhiz BS, Shamsdini S, Li J, Mohammadi M. Additive manufacturing of maraging steel-H13 bimetals using laser powder bed fusion technique. Addit Manuf. 2019;29:100797. doi: 10.1016/j.addma.2019.100797
- Fonseca EB, Gabriel AH, Araújo LC, Santos PL, Campo KN, Lopes ES. Assessment of laser power and scan speed influence on microstructural features and consolidation of AISI H13 tool steel processed by additive manufacturing. Addit Manuf. 2020;34:101250. doi: 10.1016/j.addma.2020.101250
- Lee J, Choe J, Park J, Yu J-H, Kim S, Sung H. Microstructural effects on the tensile and fracture behavior of selective laser melted H13 tool steel under varying conditions. Mater Charact. 2019;155:109817. doi: 10.1016/j.matchar.2019.109817
- Yan J, Zheng D, Li H, et al. Selective laser melting of H13: Microstructure and residual stress. J Mater Sci. 2017;52:12476-12485. doi: 10.1007/s10853-017-1380-3
- Zhang M, Chen C, Qin L, et al. Laser additive manufacturing of M2 high-speed steel. Mater Sci Technol. 2018;34(1):69-78. doi: 10.1080/02670836.2017.1355584
- Zumofen L, Beck C, Kirchheim A, Dennig HJ. Quality Related Effects of the Preheating Temperature on Laser Melted High Carbon Content Steels. In: Industrializing Additive Manufacturing - Proceedings of Additive Manufacturing in Products and Applications - AMPA2017. Cham: Springer; 2018. p. 210-219. doi: 10.1007/978-3-319-66866-6_21
- Huber F, Bischof C, Hentschel O, et al. Laser beam melting and heat-treatment of 1.2343 (AISI H11) tool steel-microstructure and mechanical properties. Mater Sci Eng A. 2019;742:109-115. doi: 10.1016/j.msea.2018.11.001
- Saewe J, Gayer C, Vogelpoth A, Schleifenbaum JH. Feasability investigation for laser powder bed fusion of high-speed steel AISI M50 with base preheating system. Berg Huettenmaenn Monatsh. 2019;164:101-107. doi: 10.1007/s00501-019-0828-y
- Boes J, Röttger A, Mutke C, Escher C, Theisen W. Microstructure and mechanical properties of X65MoCrWV3-2 cold-work tool steel produced by selective laser melting. Addit Manuf. 2018;23:170-180. doi: 10.1016/j.addma.2018.08.005
- Taha M, Yousef A, Gany K, Sabour H. On selective laser melting of ultra high carbon steel: Effect of scan speed and post heat treatment. Materialwissenschaft Werkstofftechnik. 2012;43(11):913-923. doi: 10.1002/mawe.201200030
- Casati R, Coduri M, Lecis N, Andrianopoli C, Vedani M. Microstructure and mechanical behavior of hot-work tool steels processed by selective laser melting. Mater Charact. 2018;137:50-57. doi: 10.1016/j.matchar.2018.01.015
- Yuan M, Cao Y, Karamchedu S, et al. Characteristics of a modified H13 hot-work tool steel fabricated by means of laser beam powder bed fusion. Mater Sci Eng A. 2022;831:142322. doi: 10.1016/j.msea.2021.142322
- Holzweissig MJ, Taube A, Brenne F, Schaper M, Niendorf T. Microstructural characterization and mechanical performance of hot work tool steel processed by selective laser melting. Metall Mater Trans B. 2015;46:545-549. doi: 10.1007/s11663-014-0267-9
- Kempen K, Vrancken B, Buls S, Thijs L, Van Humbeeck J, Kruth JP. Selective laser melting of crack-free high density M2 high speed steel parts by baseplate preheating. J Manuf Sci Eng. 2014;136(6):061026. doi: 10.1115/1.4028513
- Tan C, Li R, Su J, et al. Review on field assisted metal additive manufacturing. Int J Mach Tools Manuf. 2023;189:104032. doi: 10.1016/j.ijmachtools.2023.104032
- Dilip J, Ram GJ, Starr TL, Stucker B. Selective laser melting of HY100 steel: Process parameters, microstructure and mechanical properties. Addit Manuf. 2017;13:49-60. doi: 10.1016/j.addma.2016.11.003
- Kudzal AD, McWilliams BA, Taggart-Scarff J, Knezevic M. Fabrication of a low alloy ultra-high strength (>1500 MPa yield) steel using powder bed fusion additive manufacturing. Mater Sci Eng A. 2020;770:138512. doi: 10.1016/j.msea.2019.138512
- Seede R, Shoukr D, Zhang B, et al. An ultra-high strength martensitic steel fabricated using selective laser melting additive manufacturing: Densification, microstructure, and mechanical properties. Acta Mater. 2020;186:199-214. doi: 10.1016/j.actamat.2019.12.037
- Zhang S, Wang Q, Yang R, Dong C. Composition equivalents of stainless steels understood via gamma stabilizing efficiency. Sci Rep. 2021;11(1):5423. doi: 10.1038/s41598-021-84917-z
- Seede R, Zhang B, Whitt A, et al. Effect of heat treatments on the microstructure and mechanical properties of an ultra-high strength martensitic steel fabricated via laser powder bed fusion additive manufacturing. Addit Manuf. 2021;47:102255. doi: 10.1016/j.addma.2021.102255
- Agrawal P, Shukla S, Thapliyal S, et al. Microstructure-property correlation in a laser powder bed fusion processed high‐strength AF‐9628 steel. Adv Eng Mater. 2021;23(1):2000845. doi: 10.1002/adem.202000845
- Aguilar F, Huynh T, Kljestan N, Knezevic M, Sohn Y. Microstructure and mechanical characterization of AISI 4340 steel additively manufactured by laser powder bed fusion. Metals. 2025;15(4):412. doi: 10.3390/met15040412
- Yao J, Tan Q, Venezuela J, Atrens A, Zhang MX. Additive manufacturing of high-strength low-alloy AISI 4340 steel with an optimal strength-ductility-toughness trade-off. Addit Manuf. 2024;94:104496. doi: 10.1016/j.addma.2024.104496
- Song Y, Li X, Rong L, Li Y. The influence of tempering temperature on the reversed austenite formation and tensile properties in Fe–13%Cr–4%Ni–Mo low carbon martensite stainless steels. Mater Sci Eng A. 2011;528(12):4075-4079. doi: 10.1016/j.msea.2011.01.078
- Tanaka M, CHOI CS. The effects of carbon contents and Ms temperatures on the hardness of martensitic Fe-Ni-C Alloys. Trans Iron Steel Inst Japan. 1972;12(1):16-25. doi: 10.2355/isijinternational1966.12.16
- Qiao X, Han L, Zhang W, Gu J. Thermal stability of retained austenite in high-carbon steels during cryogenic and tempering treatments. ISIJ Int. 2016;56(1):140-147. doi: 10.2355/isijinternational.ISIJINT-2015-248
- Garcia-Mateo C, FG C, HKDH B. Development of hard bainite. ISIJ Int. 2003;43(8):1238-1243. doi: 10.2355/isijinternational.43.1238
- Caballero FG, Bhadeshia HKD. Very strong bainite. Curr Opin Solid State Mater Sci. 2004;8(3-4):251-257. doi: 10.1016/j.cossms.2004.09.005
- Young CH, Bhadeshia HKD. Strength of mixtures of bainite and martensite. Mater Sci Technol. 1994;10(3):209-214. doi: 10.1179/mst.1994.10.3.209
- Kawata H, Hayashi K, Sugiura N, Yoshinaga N, Takahashi M. Effect of martensite in initial structure on bainite transformation. Mater Sci Forum. 2010;638-642:3307-3312. doi: 10.4028/www.scientific.net/MSF.638-642.3307
- Bodnar RL, Hansen SS. Effects of austenite grain size and cooling rate on Widmanstätten ferrite formation in low-alloy steels. Metall Mater Trans A. 1994;25:665-675. doi: 10.1007/BF02665443
- Cochrane R, Mintz B, Ward J. Influence of prior microstructure on normalising response of C–Mn–Al–Nb steels. Mater Sci Technol. 1989;5(1):20-28. doi: 10.1179/mst.1989.5.1.20
- Krauss G. Steels: Processing, Structure, and Performance. United States: ASM International; 2015.
- Bhadeshia HKDH, Honeycombe RWK. Steels: Microstructure and Properties. United Kingdom: Butterworth-Heinemann; 2017.
- Chou CY, Pettersson NH, Durga A, et al. Influence of solidification structure on austenite to martensite transformation in additively manufactured hot-work tool steels. Acta Mater. 2021;215:117044. doi: 10.1016/j.actamat.2021.117044
- Kim KS, Kim YK, Yang S, Koo B, Lee KA. Microstructure and mechanical properties of carbon-bearing ultrahigh-strength high Co-Ni Steel (AerMet 340) fabricated via laser powder bed fusion. Materialia. 2021;20:101244. doi: 10.1016/j.mtla.2021.101244
- Krell J, Röttger A, Geenen K, Theisen W. General investigations on processing tool steel X40CrMoV5-1 with selective laser melting. J Mater Process Technol. 2018;255:679-688. doi: 10.1016/j.jmatprotec.2018.01.012
- Wang J, Van Der Wolk P, Van Der Zwaag S. On the influence of alloying elements on the bainite reaction in low alloy steels during continuous cooling. J Mater Sci. 2000;35:4393-4404. doi: 10.1023/A:1004865209116