Effect of bioactive borate glass on printability and physical properties of hydrogels
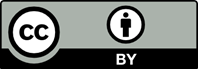
Hydrogels are a key component in bioinks and biomaterial inks for bioprinting due to their biocompatibility and printability at room temperature. The research described in the present paper contributes to the advancement of bioprinting by studying the effect of bioactive borate glass (BBG) incorporated into hydrogels on printability and physical properties. In this study, we fabricated 3D-printed hydrogel scaffolds using gelatin and alginate hydrogel mixture incorporated with various amounts of BBG, a bioceramic rich in therapeutic ions including boron, calcium, copper, and zinc. We investigated the effect of incorporating BBG on the density, viscosity, physical interactions, chemical structure, and shear thinning behavior of gelatin-alginate hydrogel biomaterial ink at different temperatures. After 3D printing and crosslinking of scaffolds, we measured mechanical properties and printing outcomes. The near-optimal extrusion temperature and pressure for uniform extrusion of hydrogel filaments at various BBG contents were determined. We compared the printing outcomes by quantifying the uniformity of printed filaments and shape fidelity of printed scaffolds. The rheological analysis showed that the addition of BBG increased the viscosity of the biomaterial inks and Young’s modulus of the 3D-printed scaffolds. Biomaterial inks with a dynamic viscosity within the range of 4.5 – 6.5 Pa·s showed the best printability across all samples. In conclusion, the inclusion of BBG contributes to a substantial improvement in the physical properties and printability of 3D-printed gelatin-alginate hydrogels.
Ming C. Leu serves as the Editorial Board Member of the journal, but did not in any way involve in the editorial and peer-review process conducted for this paper, directly or indirectly.
- Theus AS, Ning L, Hwang B, et al. Bioprintability: Physiomechanical and biological requirements of materials for 3D bioprinting processes. Polymers (Basel). 2020;12(10):2262. doi: 10.3390/polym12102262
- Matai I, Kaur G, Seyedsalehi A, McClinton A, Laurencin CT. Progress in 3D bioprinting technology for tissue/organ regenerative engineering. Biomaterials. 2020;226:119536. doi: 10.1016/J.BIOMATERIALS.2019.119536
- Groll J, Burdick JA, Cho DW, et al. A definition of bioinks and their distinction from biomaterial inks. Biofabrication. 2019;11(1):013001. doi: 10.1088/1758-5090/aaec52
- Fatimi A, Okoro OV, Podstawczyk D, Siminska- Stanny J, Shavandi A. Natural hydrogel-based bio-inks for 3D bioprinting in tissue engineering: A review. Gels. 2022;8(3):179. doi: 10.3390/gels8030179
- Mancha Sánchez E, Gómez-Blanco JC, López Nieto E, et al. Hydrogels for bioprinting: A systematic review of hydrogels synthesis, bioprinting parameters, and bioprinted structures behavior. Front Bioeng Biotechnol. 2020;8:776. doi: 10.3389/fbioe.2020.00776
- Gillispie G, Prim P, Copus J, et al. Assessment methodologies for extrusion-based bioink printability. Biofabrication. 2020;12(2):022003. doi: 10.1088/1758-5090/ab6f0d
- Fayyazbakhsh F, Leu MC. A brief review on 3D bioprinted skin substitutes. Procedia Manuf. 2020;48:790-796. doi: 10.1016/j.promfg.2020.05.115
- Fayyazbakhsh F, Khayat MJ, Leu MC. 3D-printed gelatin-alginate hydrogel dressings for burn wound healing: A comprehensive study. Int J Bioprint. 2022;8(4):618. doi: 10.18063/ijb.v8i4.618
- Mantha S, Pillai S, Khayambashi P, et al. Smart hydrogels in tissue engineering and regenerative medicine. Materials (Basel). 2019;12(20):3323. doi: 10.3390/MA12203323
- Ho TC, Chang CC, Chan HP, et al. Hydrogels: Properties and applications in biomedicine. Molecules. 2022;27(9):2902. doi: 10.3390/MOLECULES27092902
- El-Sherbiny IM, Yacoub MH. Hydrogel scaffolds for tissue engineering: Progress and challenges. Glob Cardiol Sci Pract. 2013;2013(3):316-342. doi: 10.5339/gcsp.2013.38
- Lin X, Zhao X, Xu C, Wang L, Xia Y. Progress in the mechanical enhancement of hydrogels: Fabrication strategies and underlying mechanisms. J Polym Sci. 2022;60(17):2525-2542. doi: 10.1002/pol.20220154
- Maciel BR, Baki K, Oelschlaeger C, Willenbacher N. The influence of rheological and wetting properties of hydrogel-based bio-inks on extrusion-based bioprinting. Chem Ing Tech. 2022;94(3):393-401. doi: 10.1002/cite.202100139
- Unagolla JM, Jayasuriya AC. Hydrogel-based 3D bioprinting: A comprehensive review on cell-laden hydrogels, bioink formulations, and future perspectives. Appl Mater Today. 2020;18:100479. doi: 10.1016/j.apmt.2019.100479
- Gillispie GJ, Copus J, Uzun-Per M, et al. The correlation between rheological properties and extrusion-based printability in bioink artifact quantification. Mater Des. 2023;233:112237. doi: 10.1016/j.matdes.2023.112237
- Lee SC, Gillispie G, Prim P, Lee SJ. Physical and chemical factors influencing the printability of hydrogel-based extrusion bioinks. Chem Rev. 2020;120(19):10834-10886. doi: 10.1021/acs.chemrev.0c00015
- He Y, Yang F, Zhao H, Gao Q, Xia B, Fu J. Research on the printability of hydrogels in 3D bioprinting. Sci Rep. 2016;6:29977. doi: 10.1038/srep29977
- Mondal A, Gebeyehu A, Miranda M, et al. Characterization and printability of sodium alginate-Gelatin hydrogel for bioprinting NSCLC co-culture. Sci Rep. 2019;9(1):19914. doi: 10.1038/s41598-019-55034-9
- Pepelanova I, Kruppa K, Scheper T, Lavrentieva A. Gelatin-methacryloyl (GelMA) hydrogels with defined degree of functionalization as a versatile toolkit for 3D Cell culture and extrusion bioprinting. Bioengineering (Basel). 2018;5(3):55. doi: 10.3390/BIOENGINEERING5030055
- Schwab A, Levato R, D’Este M, Piluso S, Eglin D, Malda J. Printability and shape fidelity of bioinks in 3D bioprinting. Chem Rev. 2020;120(19):11028-11055. doi: 10.1021/acs.chemrev.0c00084
- Fayyazbakhsh F, Khayat MJ, Sadler C, Day D, Huang YW, Leu MC. 3D-printed hydrogels dressings with bioactive borate glass for continuous hydration and treatment of second-degree burns. Int J Bioprint. 2023;9(6):0118. doi: 10.36922/IJB.0118
- Simorgh S, Alasvand N, Khodadadi M, et al. Additive manufacturing of bioactive glass biomaterials. Methods. 2022;208:75-91. doi: 10.1016/J.YMETH.2022.10.010
- Kolan KCR, Semon JA, Bromet B, Day DE, Leu MC. Bioprinting with human stem cell-laden alginate-gelatin bioink and bioactive glass for tissue engineering. Int J Bioprint. 2019;5(2.2):204. doi: 10.18063/ijb.v5i2.2.204
- Heid S, Boccaccini AR. Advancing bioinks for 3D bioprinting using reactive fillers: A review. Acta Biomater. 2020;113:1-22. doi: 10.1016/j.actbio.2020.06.040
- Herrada-Manchón H, Fernández MA, Aguilar E. Essential guide to hydrogel rheology in extrusion 3D printing: How to measure it and why it matters? Gels. 2023;9(7):517. doi: 10.3390/GELS9070517
- Han D, Lu Z, Chester SA, Lee H. Micro 3D printing of a temperature-responsive hydrogel using projection micro-stereolithography. Sci Rep. 2018;8(1):1963. doi: 10.1038/s41598-018-20385-2
- Fischer B, Schulz A, Gepp MM, Neubauer J, Gentile L, Zimmermann H. 3D printing of hydrogels in a temperature controlled environment with high spatial resolution. Curr Dir Biomed Eng. 2016;2(1):109-112. doi: 10.1515/cdbme-2016-0027
- Jo Y, Kim JY, Kim SY, et al. 3D-printable, highly conductive hybrid composites employing chemically-reinforced, complex dimensional fillers and thermoplastic triblock copolymers. Nanoscale. 2017;9(16):5072-5084. doi: 10.1039/c6nr09610g
- Julie Chandra CS, Sasi S, Bindu Sharmila TK. Material applications of gelatin. In: Handbook of Biopolymers. Singapore: Springer; 2023. doi: 10.1007/978-981-16-6603-2_28-1
- De Farias BS, Rizzi FZ, Ribeiro ES, et al. Influence of gelatin type on physicochemical properties of electrospun nanofibers. Sci Rep. 2023;13(1):15195. doi: 10.1038/s41598-023-42472-9
- Asim S, Tabish TA, Liaqat U, Ozbolat IT, Rizwan M. Advances in gelatin bioinks to optimize bioprinted cell functions. Adv Healthc Mater. 2023;12(17):2203148. doi: 10.1002/adhm.202203148
- Mu X, Agostinacchio F, Xiang N, et al. Recent advances in 3D printing with protein-based inks. Prog Polym Sci. 2021;115:101375. doi: 10.1016/j.progpolymsci.2021.101375
- Gregory T, Benhal P, Scutte A, et al. Rheological characterization of cell-laden alginate-gelatin hydrogels for 3D biofabrication. J Mech Behav Biomed Mater. 2022;136:105474. doi: 10.1016/j.jmbbm.2022.105474
- Abka‐khajouei R, Tounsi L, Shahabi N, Patel AK, Abdelkafi S, Michaud P. Structures, properties and applications of alginates. Mar Drugs. 2022;20(6):364. doi: 10.3390/md20060364
- Axpe E, Oyen ML. Applications of alginate-based bioinks in 3D bioprinting. Int J Mol Sci. 2016;17(12):1976. doi: 10.3390/ijms17121976
- Piras CC, Smith DK. Multicomponent polysaccharide alginate-based bioinks. J Mater Chem B. 2020;8(36):8171-8188. doi: 10.1039/d0tb01005g
- Lou L, Rubinsky B. Temperature-controlled 3D cryoprinting inks made of mixtures of alginate and agar. Gels. 2023;9(9):689. doi: 10.3390/gels9090689
- Kolan KCR, Semon JA, Bindbeutel AT, Day DE, Leu MC. Bioprinting with bioactive glass loaded polylactic acid composite and human adipose stem cells. Bioprinting. 2020;18:e00075. doi: 10.1016/j.bprint.2020.e00075
- Kolan K, Liu Y, Baldridge J, et al. Solvent based 3D printing of biopolymer/bioactive glass composite and hydrogel for tissue engineering applications. Procedia CIRP. 2017;65:38-43. doi: 10.1016/j.procir.2017.04.022
- Caroline Murphy, Kolan K, Li W, Semon J, Day D, Leu M. 3D bioprinting of stem cells and polymer/bioactive glass composite scaffolds for bone tissue engineering. Int J Bioprint. 2017;3(1):005. doi: 10.18063/IJB.2017.01.005
- Zakeri Siavashani A, Haghbin Nazarpak M, Fayyazbakhsh F, Toliyat T, McInnes SJP, Solati-Hashjin M. Effect of amino-functionalization on insulin delivery and cell viability for two types of silica mesoporous structures. J Mater Sci. 2016;51(24):10897-10909. doi: 10.1007/s10853-016-0301-1
- Siavashani AZ, Nazarpak MH, Bakhsh FF, Toliyat T, Solati- Hashjin M. Preparation of mesoporous silica nanoparticles for insulin drug delivery. Adv Mat Res. 2014;829:251-257. doi: 10.4028/www.scientific.net/AMR.829.251
- Mehrabi T, Mesgar AS, Mohammadi Z. Bioactive glasses: A promising therapeutic ion release strategy for enhancing wound healing. ACS Biomater Sci Eng. 2020;6(10):5399-5430. doi: 10.1021/acsbiomaterials.0c00528
- Solanki AK, Lali FV, Autefage H, et al. Bioactive glasses and electrospun composites that release cobalt to stimulate the HIF pathway for wound healing applications. Biomater Res. 2021;25(1):1. doi: 10.1186/s40824-020-00202-6
- Chen YH, Rao ZF, Liu YJ, et al. Multifunctional injectable hydrogel loaded with cerium-containing bioactive glass nanoparticles for diabetic wound healing. Biomolecules. 2021;11(5):702. doi: 10.3390/biom11050702
- Ege D, Zheng K, Boccaccini AR. Borate bioactive glasses (BBG): Bone regeneration, wound healing applications, and future directions. ACS Appl Bio Mater. 2022;5(8):3608-3622. doi: 10.1021/acsabm.2c00384
- Bi L, Rahaman MN, Day DE, et al. Effect of bioactive borate glass microstructure on bone regeneration, angiogenesis, and hydroxyapatite conversion in a rat calvarial defect model. Acta Biomater. 2013;9(8):8015-8026. doi: 10.1016/j.actbio.2013.04.043
- Lin Y, Brown RF, Jung SB, Day DE. Angiogenic effects of borate glass microfibers in a rodent model. J Biomed Mater Res A. 2014;102(12):4491-4499. doi: 10.1002/jbm.a.35120
- Zhao S, Li L, Wang H, et al. Wound dressings composed of copper-doped borate bioactive glass microfibers stimulate angiogenesis and heal full-thickness skin defects in a rodent model. Biomaterials. 2015;53:379-391. doi: 10.1016/j.biomaterials.2015.02.112
- Homaeigohar S, Li M, Boccaccini AR. Bioactive glass-based fibrous wound dressings. Burns Trauma. 2022;10:tkac038. doi: 10.1093/burnst/tkac038
- Ouyang L, Yao R, Zhao Y, Sun W. Effect of bioink properties on printability and cell viability for 3D bioplotting of embryonic stem cells. Biofabrication. 2016;8(3):035020. doi: 10.1088/1758-5090/8/3/035020
- Kyle S, Jessop ZM, Al-Sabah A, Whitaker IS. ‘Printability’ of candidate biomaterials for extrusion based 3D printing: State-of-the-art. Adv Healthc Mater. 2017;6(16):1700264. doi: 10.1002/adhm.201700264
- Cano-Vicent A, Tuñón-Molina A, Bakshi H, et al. Biocompatible alginate film crosslinked with Ca2+ and Zn2+ possesses antibacterial, antiviral, and anticancer activities. ACS Omega. 2023;8(27):24396-24405. doi: 10.1021/acsomega.3c01935
- Matyash M, Despang F, Ikonomidou C, Gelinsky M. Swelling and mechanical properties of alginate hydrogels with respect to promotion of neural growth. Tissue Eng Part C Methods. 2014;20(5):401-411. doi: 10.1089/ten.tec.2013.0252
- Pailler-Mattei C, Bec S, Zahouani H. In vivo measurements of the elastic mechanical properties of human skin by indentation tests. Med Eng Phys. 2008;30(5):599-606. doi: 10.1016/j.medengphy.2007.06.011
- Khalil S, Sun W. Biopolymer deposition for freeform fabrication of hydrogel tissue constructs. Mater Sci Eng C. 2007;27(3):469-478. doi: 10.1016/j.msec.2006.05.023
- Loebel C, Rodell CB, Chen MH, Burdick JA. Shear-thinning and self-healing hydrogels as injectable therapeutics and for 3D-printing. Nat Protoc. 2017;12(8):1521-1541. doi: 10.1038/nprot.2017.053