Inhibitory Potential of Chitosan Derivatives against Severe Acute Respiratory Syndrome Coronavirus 2: An In Silico Prospective
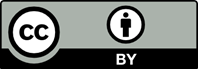
The present work was designed to investigate the antiviral potential of novel monomeric and oligomeric chitosan derivatives through in silico approaches. The goal was to identify potent broad-spectrum antiviral compounds as promising drug candidates against severe acute respiratory syndrome coronavirus 2 and understand their mode of action. Chitosan biopolymer and its derivatives were virtually screened against the spike glycoprotein and human angiotensin-converting enzyme 2 (ACE2) receptor of novel coronavirus-19. Hydroxypropyl trimethyl ammonium chloride chitosan (HTCC), a polymeric chitosan, has been reported to interact with the corona viral spike (S) protein and blocks its interaction with the ACE2 receptor. The enhancement of antiviral activity relies on better biocompatibility, structural correlations, variation in the degree of deacetylation, and molecular weight of modified chitosan derivatives. The chitosan derivatives constructively interact with viral S protein. Among the chitosan derivatives, N-carboxymethyl chitosan (NCMC) displayed efficient binding affinity. Comparing NCMC to mHTCC, monomeric chitosan, for their interaction with the S protein, receptor binding domain site, and ACE2 receptor, NCMC displayed better binding affinity of −7.9, −6.3, and −7.4 with binding energies of −6.2, −4.8, and −5.5 kcal/mol, respectively. Furthermore, through flexible docking, the interactions of the S protein with ACE2 receptor and ligand mHTCC-S protein complex and NCMC-S protein complex with ACE2 receptor were calculated, showing an efficient reduction of binding energy from −901.2 kJ/mol to −765.06 kJ/mol and −814.72 kJ/mol, respectively. This points to the decrease binding affinity of the viral S protein for the ACE2 receptor in the presence of NCMC/mHTCC. For the first time, the computational study envisages the antiviral efficiency of NCMC, mHTCC, and biocompatible chitosan derivatives as a preventive intervention against COVID-19.
[1] Jena, A.B.; Kanungo, N.; Chainy, G.B.N.; Devaraji, V.; Das, S.K.; Dandapat, J.A. Computational Insight on the Inhibitory Potential of 8‐Hydroxydihydrosanguinarine (8‐HDS), a Pyridone Containing Analog of Sanguinarine, against SARS CoV2. Chem. Biodivers., 2022, 19(11), e202200266.
[2] Jena, A.B.; Kanungo, N.; Nayak, V.; Chainy, G.B.N.; Dandapat, J. Catechin and Curcumin Interact with S Protein of SARS-CoV2 and ACE2 of Human Cell Membrane: Insights from Computational Studies. Sci. Rep., 2021, 11, 2043.
[3] Satapathy, S.; Rout, J.R.; Kerry, R.G.; Thatoi, H.; Sahoo, S.L. Biochemical Prospects of Various Microbial Pectinase and Pectin: An Approachable Concept in Pharmaceutical Bioprocessing. Front. Nutr., 2020, 7, 117.
[4] Cai, J.; Dang, Q.; Liu, C.; Fan, B.; Yan, J.; Xu, Y.; Li, J. Preparation and Characterization of N-benzoyl-O-acetyl-chitosan. Int. J. Biol. Macromol., 2015, 77, 52–8.
[5] Wang, W.; Meng, Q.; Li, Q.; Liu, J.; Zhou, M.; Jin, Z.; Zhao, K. Chitosan Derivatives and their Application in Biomedicine. Int. J. Mol. Sci., 2020, 21, 487.
[6] Wei, L.; Mi, Y.; Zhang, J.; Li, Q.; Dong, F.; Guo, Z. Evaluation of Quaternary Ammonium Chitosan Derivatives Differing in the Length of Alkyl Side-chain: Synthesis and Antifungal Activity. Int. J. Biol. Macromol., 2019, 129, 1127–32.
[7] Panda, P.K.; Yang, J.M.; Chang, Y.H. Preparation and Characterization of Ferulic Acid-modified Water Soluble Chitosan and Poly (γ-glutamic Acid) Polyelectrolyte Films through Layer-by-layer Assembly Towards Protein Adsorption. Int. J. Biol. Macromol., 2021, 171, 457–64.
[8] Yang, J.M.; Panda, P.K.; Jie, C.J.; Dash, P.; Chang, Y.H. Poly (Vinyl Alcohol)/Chitosan/Sodium Alginate Composite Blended Membrane: Preparation, Characterization, and Water-induced Shape Memory Phenomenon. Polym. Eng. Sci., 2022, 62, 1526–37.
[9] Panda, P.K.; Sadeghi, K.; Park, K.; Seo, J. Regeneration Approach to Enhance the Antimicrobial and Antioxidant Activities of Chitosan for Biomedical Applications. Polymers (Basel), 2023, 15, 132.
[10] Manisha D.P.; Chawla, R.; Dutta, P.K. ‘Click’ Synthesized Calcium-chitosan-triazole Nanocomplex from CaC2 as an Efficient Drug Carrier, Antimicrobial and Antioxidant Polymer. Int. J. Biol. Macromol., 2023, 240, 124290.
[11] Chen, Z.; Yao, X.; Liu, L.; Guan, J.; Liu, M.; Li, Z.; Yang, J.; Huang, S.; Wu, J.; Tian, F.; Jing, M. Blood Coagulation Evaluation of N-alkylated Chitosan. Carbohydr. Polym., 2017, 173, 259–68.
[12] He, X.; Xing, R.; Liu, S.; Qin, Y.; Li, K.; Yu, H.; Li, P. The Improved Antiviral Activities of Amino-modified Chitosan Derivatives on Newcastle Virus. Drug Chem. Toxicol., 2019, 44, 355–40.
[13] Mohammadi, E.; Daraei, H.; Ghanbari, R.; Athar, S.D.; Zandsalimi, Y.; Ziaee, A.; Maleki, A.; Yetilmezsoy, K. Synthesis of Carboxylated Chitosan Modified with Ferromagnetic Nanoparticles for Adsorptive Removal of Fluoride, Nitrate, and Phosphate Anions from Aqueous Solutions. J. Mol. Liquids, 2019, 273, 116–24.
[14] Benediktsdóttir, B.E.; Baldursson, Ó.; Másson, M. Challenges in Evaluation of Chitosan and Trimethylated Chitosan (TMC) as Mucosal Permeation Enhancers: From Synthesis to in Vitro Application. J. Control Release, 2014, 173, 18–31.
[15] Cheah, W.Y.; Show, P.L.; Ng, I.S.; Lin, G.Y.; Chiu, C.Y.; Chang, Y.K. Antibacterial Activity of Quaternized Chitosan Modified Nanofiber Membrane. Int. J. Biol. Macromol., 2019, 126, 569–77.
[16] Xue, H.; Hu, L.; Xiong, Y.; Zhu, X.; Wei, C.; Cao, F.; Zhou, W.; Sun, Y.; Endo, Y.; Liu, M.; Liu, Y.; Liu, J.; Abududilibaier, A.; Chen, L.; Yan, C.; Mi, B.; Liu, G. Quaternized Chitosan-matrigel-polyacrylamide Hydrogels as Wound Dressing for Wound Repair and Regeneration. Carbohydr. Polym., 2019, 226, 115302.
[17] Milewska, A.; Chi, Y.; Szczepanski, A.; Barreto-Duran, E.; Dabrowska, A.; Botwina, P.; Obloza, M.; Liu, K.; Liu, D.; Guo, X.; Ge, Y.; Li, J.; Cui, L.; Ochman, M.; Urlik, M.; Rodziewicz-Motowidlo, S.; Zhu, F.; Szczubialka, K.; Nowakowska, M.; Pyrc, K. HTCC as a Polymeric Inhibitor of SARS-CoV-2 and MERS-CoV. J. Virol., 2021, 95, e01622–20.
[18] Milewska, A.; Kaminski, K.; Ciejka, J.; Kosowicz, K.; Zeglen, S.; Wojarski, J.; Nowakowska, M.; Szczubiałka, K.; Pyrc, K. HTCC: Broad Range Inhibitor of Coronavirus Entry. PLoS One, 2016, 11, e0156552.
[19] Milewska, A.; Ciejka, J.; Kaminski, K.; Karewicz, A.; Bielska, D.; Zeglen, S.; Karolak, W.; Nowakowska, M.; Potempa, J.; Bosch, B.J.; Pyrc, K.; Szczubialka, K. Novel Polymeric Inhibitors of HCoV-NL63. Antiviral Res., 2013, 97, 112–21.
[20] Chirkov, S.N. The Antiviral Activity of Chitosan (Review). Prikl. Biokhim. Mikrobiol., 2002, 38, 5–13.
[21] Jones, D.T.; Taylor, W.R.; Thornton, J.M. The Rapid Generation of Mutation Data Matrices from Protein Sequences. Comput. Appl. Biosci., 1992, 8, 275–82.
[22] Jena, A.B.; Samal, R.R.; Kumari, K.; Pradhan, J.; Chainy, G.B.N.; Subudhi, U.; Pal, S.; Dandapat, J. The Benzene Metabolite p-benzoquinone Inhibits the Catalytic Activity of Bovine Liver Catalase: A Biophysical Study. Int. J. Biol. Macromol., 2021, 167, 871–80.
[23] Li, M.; Ding, J.; Tao, Y.; Shi, B.; Chen, J.H. Polysaccharides for Biomedical Applications. Int. J. Polym. Sci., 2019, 2019, 7841836.
[24] Pettersen, E.F.; Goddard, T.D.; Huang, C.C.; Couch, G.S.; Greenblatt, D.M.; Meng, E.C.; Ferrin, T.E. UCSF Chimera--a Visualization System for Exploratory Research and Analysis. J. Comput. Chem., 2004, 25, 1605–12.
[25] Trott, O.; Olson, A.J. AutoDock Vina: Improving the Speed and Accuracy of Docking with a New Scoring Function, Efficient Optimization, and Multithreading. J. Comput. Chem., 2010, 31, 455–61.
[26] Dhanasekaran, S.; Rameshthangam, P.; Venkatesan, S.; Singh, S.K.; Vijayan, S.R. In Vitro and in Silico Studies of Chitin and Chitosan Based Nanocarriers for Curcumin and Insulin Delivery. J. Polym. Environ., 2018, 26, 4095–113.
[27] Gao, H.; Mei, S.; Zhao, J.; Zheng, K.; Liao, S. Study on the Binding Mode of a Pyrrolotriazin Derivative with JAK2 by Docking and MD Simulation. Mol. Simul., 2018, 45, 1–9.
[28] Sawicki, S.G.; Sawicki, D.L.; Siddell, S.G. A Contemporary View of Coronavirus Transcription. J. Virol., 2007, 81, 20–9.
[29] Pi, M.; Kapoor, K.; Ye, R.; Nishimoto, S.K.; Smith, J.C.; Baudry, J.; Quarles, L.D. Evidence for Osteocalcin Binding and Activation of GPRC6A in β-cells. Endocrinology, 2016, 157, 1866–80.
[30] Ishihara, C.; Yoshimatsu, K.; Tsuji, M.; Arikawa, J.; Saiki, I.; Tokura, S.; Azuma, I. Anti-viral Activity of Sulfated Chitin Derivatives Against Friend Murine Leukaemia and Herpes Simplex Type-1 Viruses. Vaccine, 1993, 11, 670–4.
[31] Malik, A.; Gupta, M.; Gupta, V.; Gogoi, H.; Bhatnagar, R. Novel Application of Trimethyl Chitosan as an Adjuvant in Vaccine Delivery. Int. J. Nanomedicine, 2018, 13, 7959–70.
[32] Tao, W.; Zheng, H.Q.; Fu, T.; He, Z.J.; Hong, Y. N-(2-hydroxy) Propyl-3-trimethylammonium Chitosan Chloride: An Immune-enhancing Adjuvant for Hepatitis E Virus Recombinant Polypeptide Vaccine in Mice. Hum. Vaccin. Immunother., 2017, 13, 1818–22.
[33] Loutfy, S.A.; Abdel-Salam, A.I.; Moatasim, Y.; Gomaa, M.R.; Fattah, N.F.A.; Emam, M.H.; Ali, F.; ElShehaby, H.A.; Ragab, E.A.; El-Din, H.M.A.; Mostafa, A.; Ali, M.A.; Kasry, A. Antiviral Activity of Chitosan Nanoparticles Encapsulating Silymarin (SIL-CNPS) against SARS-COV-2 (in Silico and in Vitro Study). RSC Adv., 2022, 12, 15775–86.
[34] Zhuo, S.H.; Wu, J.J.; Zhao, L.; Li, W.H.; Zhao, Y.F.; Li, Y.M. A Chitosan-mediated Inhalable Nanovaccine Against SARS-CoV-2. Nano Res., 2022, 15, 4191–200.
[35] Pereira, P.F.S.; de Paula E Silva, A.C.A.; da Silva Pimentel, B.N.A.; Pinatti, I.M.; Simões, A.Z.; Vergani, C.E.; Barreto-Vieira, D.F.; da Silva, M.A.N.; Miranda, M.D.; Monteiro, M.E.S.; Tucci, A.; Doñate-Buendía, C.; Mínguez-Vega, G.; Andrés, J.; Longo, E. Inactivation of SARS-CoV-2 by a Chitosan/α-Ag2WO4 Composite Generated by Femtosecond Laser Irradiation. Sci. Rep., 2022, 12, 8118.
[36] Vörös-Horváth, B.; Živković, P.; Bánfai, K.; Bóvári-Biri, J.; Pongrácz, J.; Bálint, G.; Pál, S.; Széchenyi, A. Preparation and Characterization of ACE2 Receptor Inhibitor-loaded Chitosan Hydrogels for Nasal Formulation to Reduce the Risk of COVID-19 Viral Infection. ACS Omega, 2022, 7, 3240–53.
[37] Ciejka, J.; Wolski, K.; Nowakowska, M.; Pyrc, K.; Szczubiałka, K. Biopolymeric Nano/Microspheres for Selective and Reversible Adsorption of Coronaviruses. Mater. Sci. Eng. C Mater. Biol. Appl., 2017, 76, 735–42.
[38] Pramanik, A.; Jones, S.; Gao, Y.; Sweet, C.; Begum, S.; Shukla, M.K.; Buchanan, J.P.; Moser, R.D.; Ray, P.C. Bio-conjugated Chitosan Wrapped CNT Based 3D Nanoporous Architecture for Separation and Inactivation of Rotavirus and Shigella Waterborne Pathogens. J. Mater. Chem. B, 2017, 5, 9522–31.