3D Aerosol Jet® printing for microstructuring: Advantages and limitations
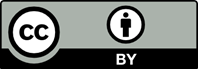
Aerosol Jet® printing (AJ®P) is a direct writing printing technology that deposits functional aerosolized solutions on free-form substrates. Its potential has been widely adopted for two-dimensional (2D) microscale constructs in printed electronics (PE), and it is rapidly growing toward surface structuring and biological interfaces. However, limited research has been devoted to its exploitation as a three-dimensional (3D) printing technique. In this study, we investigated AJ®P capabilities for 3D microstructuring of three inks, as well as their advantages and limitations by employing three proposed 3D AJ®P strategies (continuous jet deposition, layer-by-layer, and point-wise). In particular, 3D microstructures of increasing complexity based on silver nanoparticle (AgNPs)-, poly(3,4-ethylenedioxythiophene)polystyrene sulfonate (PEDOT:PSS)-, and collagen-based inks were investigated at various aspect ratios and resolutions. Biocompatibility assays were also performed to evaluate inks cytotoxicity effects on selected cellular lineages, including neuronal and osteoblast cell lines. Results show the possibility to print not only arrays of micropillars of different aspect ratios (AgNPs-ARs ~ 20, PEDOT:PSS-ARs ~ 4.5, collagen-ARs ~ 2.5), but also dense and complex (yet low reproducible) leaf- or flake-like structures (especially with the AgNPs-based ink), and lattice units (collagen-based ink). Specifically, this study demonstrates that the fabrication of 3D AJ®-printed microstructures is possible only with a specific set of printing parameters, and firmly depends on the ink (co-)solvents fast-drying phenomena during the printing process. Furthermore, the data concerning inks biocompatibility revealed high cytotoxicity levels for the AgNPs-based ink, while low ones for the PEDOT:PSS and the collagen-based inks. In conclusion, the paper provides general guidelines with respect to ink development and print strategies for 3D AJ®P microstructuring, opening its adoption in a vast range of applications in life science (tissue engineering, bioelectronic interfaces), electronics, and micromanufacturing.
- Xu J, Wang X, Wang C, et al., 2021, A review on micro/ nanoforming to fabricate 3D metallic structures. Adv Mater, 33(6):2000893. https//doi.org/10.1002/adma.202000893
- Vaezi M, Seitz H, Yang S, 2013, A review on 3D micro-additive manufacturing technologies. Int J Adv Manuf Technol, 67:1721–1754.
- Yunas J, Mulyanti B, Hamidah I, et al., 2020, Polymer-based MEMS electromagnetic actuator for biomedical application: A review. Polymer, 12:1184. https://doi.org/10.1007/s00170-012-4605-2
- Moitzheim S, Put B, Vereecken PM, 2019, Advances in 3D thin-film Li-ion batteries. Adv Mater Interfaces, 6(15):1900805. https//doi.org/10.1002/admi.201900805
- Moitzheim S, Balder JE, Ritasalo R, et al., 2019, Toward 3D thin-film batteries: Optimal current-collector design and scalable fabrication of TiO2 thin-film electrodes. ACS Appl Energy Mater, 2(3):1774–1783. https//doi.org/10.1021/acsaem.8b01905
- Kong T, Luo G, Zhao Y, et al., 2019, Bioinspired superwettability micro/nanoarchitectures: Fabrications and applications. Adv Funct Mater, 29(11):1808012. https//doi.org/10.1002/adfm.201808012
- Penmatsa V, Kim T, Beidaghi M, et al., 2012, Three-dimensional graphene nanosheet encrusted carbon micropillar arrays for electrochemical sensing. Nanoscale, 4(12):3673–3678. https//doi.org/10.1039/c2nr30161j
- Lao Z, Xia N, Wang S, et al., 2021, Tethered and untethered 3D microactuators fabricated by two-photon polymerization: A review. Micromachines, 12(4):465. https//doi.org/10.3390/mi12040465
- Qian Y, Magginetti DJ, Jeon S, et al., 2020, Heterogeneous optoelectronic characteristics of Si micropillar arrays fabricated by metal-assisted chemical etching. Sci Rep, 10(1):1–10. https//doi.org/10.1038/s41598-020-73445-x
- Park YG, Min H, Kim H, et al., 2019, Three-dimensional, high-resolution printing of carbon nanotube/liquid metal composites with mechanical and electrical reinforcement. Nano Lett, 19(8):4866–4872. https//doi.org/10.1021/acs.nanolett.9b00150
- Lejeune M, Chartier T, Dossou-Yovo C, et al., 2009, Ink-jet printing of ceramic micro-pillar arrays. J Eur Ceram Soc, 29(5):905–911. https//doi.org/10.1016/j.jeurceramsoc.2008.07.040
- Jannah F, Kim JH, Lee JW, et al., 2018, Immobilized polydiacetylene lipid vesicles on polydimethylsiloxane micropillars as a surfactin-based label-free bacterial sensor platform. Front Mater, 5:57. https//doi.org/10.3389/fmats.2018.00057
- Cutarelli A, Ghio S, Zasso J, et al., 2020, Vertically-aligned functionalized silicon micropillars for 3D culture of human pluripotent stem cell-derived cortical progenitors. Cells, 9(1):88. https//doi.org/10.3390/cells9010088
- Wang X, Yu H, Yang T, et al., 2021, Density regulation and localization of cell clusters by self-assembled femtosecond-laser-fabricated micropillar arrays. ACS Appl Mater Interfaces, 13(49):58261–58269. https//doi.org/10.1021/acsami.1c13818
- Fan S, Qi L, Li J, et al., 2021, Guiding the patterned growth of neuronal axons and dendrites using anisotropic micropillar scaffolds. Adv Healthc Mater, 10(12):2100094. https//doi.org/10.1002/adhm.202100094
- Palankar R, Glaubitz M, Martens U, et al., 2016, 3D micropillars guide the mechanobiology of human induced pluripotent stem cell-derived cardiomyocytes. Adv Healthc Mater, 5(3):335–341. https//doi.org/10.1002/adhm.201500740
- Klein F, Richter B, Striebel T, et al., 2011, Two-component polymer scaffolds for controlled three-dimensional cell culture. Adv Mater, 23(11):1341–1345. https//doi.org/10.1002/adma.201004060
- Liu Y, McGuire AF, Lou HY, et al., 2018, Soft conductive micropillar electrode arrays for biologically relevant electrophysiological recording. Proc Natl Acad Sci U S A, 115(46):11718–11723. https//doi.org/10.1073/pnas.1810827115
- Yadav A, Verma N, 2019, Efficient hydrogen production using Ni-graphene oxide-dispersed laser-engraved 3D carbon micropillars as electrodes for microbial electrolytic cell. Renew Energy, 138:628–638. https//doi.org/10.1016/j.renene.2019.01.100
- Goh GL, Zhang H, Chong TH, et al., 2021, 3D printing of multilayered and multimaterial electronics: A review. Adv Electron Mater, 7(10):2100445. https//doi.org/10.1002/aelm.202100445
- Tan HW, Choong YYC, Kuo CN, et al., 2022, 3D printed electronics: Processes, materials and future trends. Prog Mater Sci, 127:100945. https//doi.org/10.1016/j.pmatsci.2022.100945
- Cooper C, Hughes B, 2020, Aerosol jet printing of electronics: An enabling technology for wearable devices. Pan Pac Microelectron Symp, 1–11. https//doi.org/10.23919/PanPacific48324.2020.9059444
- Machiels J, Verma A, Appeltans R, et al., 2021, Printed electronics (PE) as an enabling technology to realize flexible mass customized smart applications. Proc CIRP, 96:115–120. https//doi.org/10.1016/j.procir.2021.01.062
- Goh GL, Dikshit V, Koneru R, et al., 2022, Fabrication of design-optimized multifunctional safety cage with conformal circuits for drone using hybrid 3D printing technology. Int J Adv Manuf Technol, 120(3–4):2573–2586. https//doi.org/10.1007/s00170-022-08831-y
- Paulsen JA, Renn M, Christenson K, et al., 2012, Printing conformal electronics on 3D structures with aerosol jet technology, in Future of Instrumentation International Workshop (FIIW) Proceedings, IEEE, 1–4. https//doi.org/10.1109/FIIW.2012.6378343
- Seiti M, Ginestra PS, Ferraro RM, et al., 2022, Aerosol Jet® printing of poly(3,4-ethylenedioxythiophene): Poly(styrenesulfonate) onto micropatterned substrates for neural cells in vitro stimulation. Int J Bioprint, 8(1):504. https//doi.org/10.18063/ijb.v8i1.504
- Williams NX, Watson N, Joh DY, et al., 2020, Aerosol jet printing of biological inks by ultrasonic delivery. Biofabrication, 12(2):025004. https//doi.org/10.1088/1758-5090/ab5cf5
- Saleh MS, Hu C, Panat R, 2017, Three-dimensional microarchitected materials and devices using nanoparticle assembly by pointwise spatial printing. Sci Adv, 3(3):e1601986. https//doi.org/10.1126/sciadv.1601986
- Zips S, Grob L, Rinklin P, et al., 2019, Fully printed μ-needle electrode array from conductive polymer ink for bioelectronic applications. ACS Appl Mater Interfaces, 11(36):32778–32786. https//doi.org/10.1021/acsami.9b11774
- Hohnholz A, Obata K, Nakajima Y, et al., 2019, Hybrid UV laser direct writing of UV-curable PDMS thin film using aerosol jet printing. Appl Phys A Mater Sci Process, 125(2):1–6. https//doi.org/10.1007/s00339-018-1902-0
- Di Novo NG, Cantù E, Tonello S, et al., 2019, Support-material-free microfluidics on an electrochemical sensors platform by aerosol jet printing. Sensors, 19(8). https//doi.org/10.3390/s19081842
- Vlnieska V, Gilshtein E, Kunka D, et al., 2022, Aerosol jet printing of 3D pillar arrays from photopolymer ink. Polymers, 14(16):3411. https//doi.org/10.3390/polym14163411
- Secor EB, 2018, Principles of aerosol jet printing. Flex Print Electron, 3(3). https//doi.org/10.1088/2058-8585/aace28
- Wilkinson NJ, Smith MAA, Kay RW, et al., 2019, A review of aerosol jet printing—A non-traditional hybrid process for micro-manufacturing. Int J Adv Manuf Technol, 1–21. https//doi.org/10.1007/s00170-019-03438-2
- Williams BA, Trejo ND, Wu A, et al., 2017, Copper–zinc– tin–sulfide thin films via annealing of ultrasonic spray deposited nanocrystal coatings. ACS Appl Mater Interfaces, 9(22):18865–18871. https//doi.org/10.1021/acsami.7b04414
- Gibney R, Ferraris E, 2021, Bioprinting of collagen type I and II via aerosol jet printing for the replication of dense collagenous tissues. Front Bioeng Biotechnol, 9:1–12. https//doi.org/10.3389/fbioe.2021.786945
- Seiti M, Ginestra P, Ferraro RM, et al., 2020, Nebulized jet-based printing of bio-electrical scaffolds for neural tissue engineering: A feasibility study. Biofabrication, 12(2):025024. https//doi.org/10.1088/1758-5090/ab71e0
- Johnston HJ, Hutchison G, Christensen FM, et al., 2010, A review of the in vivo and in vitro toxicity of silver and gold particulates: Particle attributes and biological mechanisms responsible for the observed toxicity. Crit Rev Toxicol, 40(4):328–346. https//doi.org/10.3109/10408440903453074
- Ali E, Ferraro RM, Lanzi G, et al., 2020, Generation of induced pluripotent stem cell (iPSC) lines from a Joubert syndrome patient with compound heterozygous mutations in C5orf42 gene. Stem Cell Res, 49:102007. https//doi.org/10.1016/j.scr.2020.102007
- Ferraro RM, Ginestra PS, Giliani S, et al., 2020, Carbonization of polymer precursors substrates to direct human iPSC-derived neurons differentiation and maturation. Proc CIRP, 39–44. https//doi.org/10.1016/j.procir.2020.05.116
- Degryse O, Bloemen V, Ferraris E, 2022, Collagen composite inks for Aerosol Jet® printing in bone tissue engineering applications. Proc CIRP, 110(C):180–185. https//doi.org/10.1016/j.procir.2022.06.033
- Hart GJ, Russell AE, Cooper DR, 1971, The effects of certain glycols, substituted glycols and related organic solvents on the thermal stability of soluble collagen. Biochem J, 125(2):599–604. https//doi.org/10.1042/bj1250599
- Penkova R, Goshev I, Gorinstein S, et al., 1999, Stabilizing effect of glycerol on collagen type I isolated from different species. Food Chem, 66(4):483–487. https//doi.org/10.1016/S0308-8146(99)00097-7
- Usha R, Ramasami T, 2008, Role of solvents in stability of collagen. J Therm Anal Calorim, 93(2):541–545. https//doi.org/10.1007/s10973-006-7887-5
- Li J, Li G, 2011, The thermal behavior of collagen in solution: Effect of glycerol and 2-propanol. Int J Biol Macromol, 48(2):364–368. https//doi.org/10.1016/j.ijbiomac.2010.12.013
- Ronsin O, Caroli C, Baumberger T, 2017, Preferential hydration fully controls the renaturation dynamics of collagen in water-glycerol solvents. Eur Phys J E, 40(5):1–5. https//doi.org/10.1140/epje/i2017-11545-1
- Vagenende V, Yap MGS, Trout BL, 2009, Mechanisms of protein stabilization and prevention of protein aggregation by glycerol. Biochemistry, 48(46):11084–11096. https//doi.org/10.1021/bi900649t
- Na GC, Butz LJ, Bailey DG, et al., 1986, In vitro collagen fibril assembly in glycerol solution: Evidence for a helical cooperative mechanism involving microfibrils. Biochemistry, 25(5):958–966. https//doi.org/ 10.1021/bi00353a003
- Yu L, Rowe DW, Perera IP, et al., 2020, Intrafibrillar mineralized collagen-hydroxyapatite-based scaffoldsfor bone regeneration. ACS Appl Mater Interfaces, 12(16):18235– 18249. https//doi.org/10.1021/acsami.0c00275
- Pongrac IM, Ahmed LB, Mlinarić H, et al., 2018, Surface coating affects uptake of silver nanoparticles in neural stem cells. J Trace Elem Med Biol, 50:684–692. https//doi.org/10.1016/j.jtemb.2017.12.003
- Zhang XF, Liu ZG, Shen W, et al., 2016, Silver nanoparticles: Synthesis, characterization, properties, applications, and therapeutic approaches. Int J Mol Sci, 17(9):1534. https//doi.org/10.3390/ijms17091534
- McShan D, Ray PC, Yu H, 2014, Molecular toxicity mechanism of nanosilver. J Food Drug Anal, 22(1):116–127. https//doi.org/10.1016/j.jfda.2014.01.010
- Ferraro RM, Seiti M, Ginestra PS, et al., 2022, Biocompatibility evaluation of encapsulated silver-based printed circuits for in-vitro long-term sensing devices. Proc CIRP, 110(C):99–104. https//doi.org/10.1016/j.procir.2022.06.020.
- Renn MJ, Schrandt M, Renn J, et al., 2017, Localized laser sintering of metal nanoparticle inks printed with Aerosol Jet® technology for flexible electronics. J Microelectron Electron Packag, 14:132–139. https//doi.org/10.4071/imaps.521797
- Wei Tan H, An J, Kai Chua C, et al., 2019, Metallic nanoparticle inks for 3D printing of electronics. Adv Electron Mater, 5(5):1800831. https//doi.org/10.1002/aelm.201800831
- Seiti M, Degryse O, Ferraris E, 2022, Aerosol Jet® printing 3D capabilities for metal and polymeric inks. Mater Today Proc, 70:38–44. https//doi.org/10.1016/j.matpr.2022.08.488