3D printing of PCL-ceramic composite scaffolds for bone tissue engineering applications
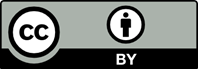
Three-dimensional (3D) printing was utilized for the fabrication of a composite scaffold of poly(ε-caprolactone) (PCL) and calcium magnesium phosphate (CMP) bioceramics for bone tissue engineering application. Four groups of scaffolds, that is, PMC-0, PMC-5, PMC-10, and PMC-15, were fabricated using a custom 3D printer. Rheology analysis, surface morphology, and wettability of the scaffolds were characterized. The PMC-0 scaffolds displayed a smoother surface texture and an increase in the ceramic content of the composite scaffolds exhibited a rougher structure. The hydrophilicity of the composite scaffold was significantly enhanced compared to the control PMC-0. The effect of ceramic content on the bioactivity of fibroblast NIH/3T3 cells in the composite scaffold was investigated. Cell viability and toxicity studies were evaluated by comparing results from lactate dehydrogenase (LDH) and Alamar Blue (AB) colorimetric assays, respectively. The live-dead cell assay illustrated the biocompatibility of the tested samples with more than 100% of live cells on day 3 compared to the control one. The LDH release indicated that the composite scaffolds improved cell attachment and proliferation. In this research, the fabrication of a customized composite 3D scaffold not only mimics the rough textured architecture, porosity, and chemical composition of natural bone tissue matrices but also serves as a source for soluble ions of calcium and magnesium that are favorable for bone cells to grow. These 3D-printed scaffolds thus provide a desirable microenvironment to facilitate biomineralization and could be a new effective approach for preparing constructs suitable for bone tissue engineering.
- Hasan A, 2017, Tissue Engineering for Artificial Organs: Regenerative Medicine, Smart Diagnostics and Personalized Medicine. United States: John Wiley and Sons.
- Van Blitterswijk C, de Boer J, Thomsen P, et al., 2008, Tissue Engineering. Netherlands: Elsevier.
- Williams DF, 2009, On the nature of biomaterials. Biomaterials, 30: 5897–5909. https://doi.org/10.1016/j.biomaterials.2009.07.027
- Williams DF, 2014, The biomaterials conundrum in tissue engineering. Tissue Eng Part A, 20: 1129–1131. https://doi.org/10.1089/ten.tea.2013.0769
- Lee EJ, Kasper FK, Mikos AG, 2014, Biomaterials for tissue engineering. Ann Biomed Eng, 42: 323–337. https://doi.org/10.1007/s10439–013–0859–6
- Dhandayuthapani B, Yoshida Y, Maekawa T, et al., 2011, Polymeric scaffolds in tissue engineering application: A review. Int J Polym Sci, 2011: 290602. https://doi.org/10.1155/2011/290602
- Desai S, Bidanda B, Bártolo PJ, 2021, Emerging trends in the applications of metallic and ceramic biomaterials. In: Bio-Materials and Prototyping Applications in Medicine. Germany: Springer. p1–17.
- Desai S, Shankar MR, 2021, Emerging trends in polymers, composites, and nano biomaterial applications. In: Bio- Materials and Prototyping Applications in Medicine. Germany: Springer, p19–34.
- Ratner BD, Hoffman AS, Schoen FJ, et al., 2004, Biomaterials Science: An Introduction to Materials in Medicine. San Diego, California: Academic Press. p162–164.
- Hanker JS, Giammara BL, 1988, Biomaterials and biomedical devices. Science, 242: 885–892. https://doi.org/10.1126/science.3055300.
- Hasirci V, Hasirci N, 2018, Fundamentals of Biomaterials. Germany: Springer.
- Chen FM, Liu X, 2016, Advancing biomaterials of human origin for tissue engineering. Prog Polym Sci, 53: 86–168. https:/doi.org/10.1016/j.progpolymsci.2015.02.004
- O’Brien FJ, 2011, Biomaterials and scaffolds for tissue engineering. Mater Today, 14: 88–95. https://doi.org/10.1016/S1369-7021(11)70058-X
- Keane TJ, Badylak SF, 2014, Biomaterials for tissue engineering applications. In: Seminars in Pediatric Surgery. Vol. 23. Netherlands: Elsevier. p112–118.
- Burdick JA, Mauck RL, 2010, Biomaterials for Tissue Engineering Applications: A Review of the Past and Future Trends. Germany: Springer.
- López‐Rodríguez N, López‐Arraiza A, Meaurio E, et al., 2006, Crystallization, morphology, and mechanical behavior of polylactide/poly (ε‐caprolactone) blends. Polym Eng Sci, 46: 1299–1308. https://doi.org/10.1002/pen.20609
- Yoshimoto H, Shin YM, Terai H, et al., 2003, A biodegradable nanofiber scaffold by electrospinning and its potential for bone tissue engineering. Biomaterials, 24: 2077–2082. https://doi.org/10.1016/s0142-9612(02)00635-x
- Rezwan K, Chen QZ, Blaker JJ, et al., 2006, Biodegradable and bioactive porous polymer/inorganic composite scaffolds for bone tissue engineering. Biomaterials, 27: 3413–3431. https://doi.org/10.1016/j.biomaterials.2006.01.039
- Ma PX, 2004, Scaffolds for tissue fabrication. Mater Today, 7: 30–40. https://doi.org/10.1016/S1369-7021(04)00233-0
- Choi SW, Zhang Y, Xia Y, 2010, Three-dimensional scaffolds for tissue engineering: The importance of uniformity in pore size and structure. Langmuir, 26: 19001–19006. https://doi.org/10.1021/la104206h
- Desai S, Harrison B, 2010, Direct-writing of biomedia for drug delivery and tissue regeneration. In: Printed Biomaterials: Novel Processing and Modeling Techniques for Medicine and Surgery. Germany: Springer Verlag.
- Desai S, Perkins J, Harrison BS, et al., 2010, Understanding release kinetics of biopolymer drug delivery microcapsules for biomedical applications. Mater Sci Eng B, 168: 127–131. https://doi.org/10.1016/j.mseb.2009.11.006
- Desai S, Moore A, Harrison B, et al., 2008, Understanding microdroplet formations for biomedical applications. In: ASME International Mechanical Engineering Congress and Exposition. New York, United States: ASME. p1–4.
- Abedalwafa M, Wang F, Wang L, et al., 2013, Biodegradable poly-epsilon-caprolactone (PCL) for tissue engineering applications: A review. Rev Adv Mater Sci, 34: 123–140.
- Grandi C, di Liddo R, Paganin P, et al., 2011, Porous alginate/poly (ε-caprolactone) scaffolds: Preparation, characterization and in vitro biological activity. Int J Mol Med, 27: 455–467. https://doi.org/10.3892/ijmm.2010.593
- Zhang XC, 2016, Science and Principles of Biodegradable and Bioresorbable Medical Polymers: Materials and Properties. Sawston, United Kingdom: Woodhead Publishing.
- Li W, Danielson KG, Alexander PG, et al., 2003, Biological response of chondrocytes cultured in three‐dimensional nanofibrous poly (ϵ‐caprolactone) scaffolds. J Biomed Mater Res A, 67: 1105–1114. https://doi.org/10.1002/jbm.a.10101
- Albertsson AC, Varma IK, 2003, Recent developments in ring opening polymerization of lactones for biomedical applications. Biomacromolecules, 4: 1466–1486. https://doi.org/10.1021/bm034247a
- Saudi S, Bhattarai SR, Adhikari U, et al., 2020, Nanonet-nano fiber electrospun mesh of PCL-chitosan for controlled and extended release of diclofenac sodium. Nanoscale, 12: 23556–23569. https://doi.org/10.1039/D0NR05968D
- Adarkwa E, Kotoka R, Desai S, 2021, 3D printing of polymeric Coatings on AZ31 Mg alloy Substrate for Corrosion Protection of biomedical implants. Med Devices Sens, 4: e10167. https://doi.org/10.1002/MDS3.10167
- Marquetti I, Desai S, 2018, Adsorption behavior of bone morphogenetic protein-2 on a graphite substrate for biomedical applications. Am J Eng Appl Sci, 11: 1037–1044. https://doi.org/10.3844/ajeassp.2018.1037.1044
- Wu F, Liu C, O’Neill B, et al., 2012, Fabrication and properties of porous scaffold of magnesium phosphate/ polycaprolactone biocomposite for bone tissue engineering. Appl Surf Sci, 258: 7589–7595. https://doi.org/10.1016/j.apsusc.2012.04.094
- Liu L, Wang Y, Guo S, et al., 2012, Porous polycaprolactone/ nanohydroxyapatite tissue engineering scaffolds fabricated by combining NaCl and PEG as co-porogens: Structure, property, and chondrocyte-scaffold interaction in vitro. J Biomed Mater Res B Appl Biomater, 100: 956–966. https://doi.org/10.1002/JBM.B.32658
- Diba M, Kharaziha M, Fathi MH, et al., 2012, Preparation and characterization of polycaprolactone/forsterite nanocomposite porous scaffolds designed for bone tissue regeneration. Compos Sci Technol, 72: 716–723. https://doi.org/10.1016/J.COMPSCITECH.2012.01.023
- Lei B, Shin KH, Noh DY, et al., 2012, Bioactive glass microspheres as reinforcement for improving the mechanical properties and biological performance of poly(ε-caprolactone) polymer for bone tissue regeneration. J Biomed Mater Res B Appl Biomater, 100B: 967–975. https://doi.org/10.1002/JBM.B.32659
- Dong Z, Wu Y, Wang Q, et al., 2012, Reinforcement of electrospun membranes using nanoscale Al2O3 whiskers for improved tissue scaffolds. J Biomed Mater Res A, 100: 903–910. https://doi.org/10.1002/jbm.a.34027
- Marquetti I, Desai S, 2019, Orientation effects on the nanoscale adsorption behavior of bone morphogenetic protein-2 on hydrophilic silicon dioxide. RSC Adv, 9: 906–916. https://doi.org/10.1039/C8RA09165J
- Marquetti I, Desai S, 2018, Molecular modeling the adsorption behavior of bone morphogenetic protein-2 on hydrophobic and hydrophilic substrates. Chem Phys Lett, 706: 285–294. https://doi.org/10.1016/j.cplett.2018.06.015
- Marquetti I, Desai S, 2022, An atomistic investigation of adsorption of bone morphogenetic protein-2 on gold with nanoscale topographies. Surfaces, 5: 176–185. https://doi.org/10.3390/surfaces5010010
- Huang B, Caetano G, Vyas C, et al., 2018, Polymer-ceramic composite scaffolds: The effect of hydroxyapatite and β-tri-calcium phosphate. Materials, 11: 129. https://doi.org/10.3390/ma11010129
- Klein CP, Driessen AA, de Groot K, et al., 1983, Biodegradation behavior of various calcium phosphate materials in bone tissue. J Biomed Mater Res, 17: 769–784. https://doi.org/10.1002/jbm.820170505
- Parupelli S, Desai S, 2019, A comprehensive review of additive manufacturing (3d printing): Processes, applications and future potential. Am J Appl Sci, 16: 244–272. https://doi.org/10.3844/ajassp.2019.244.272
- Parupelli SK, Aljohani A, Desai S, 2019, Direct jet printing and characterization of calcium alginate microcapsules for biomedical applications. In: Proceedings of the 2019 IISE Annual Conference. Florida, USA: Institute of Industrial and Systems Engineers (IISE).
- Perkins J, Xu Z, Smith C, et al., 2015, Direct writing of polymeric coatings on magnesium alloy for tracheal stent applications. Ann Biomed Eng, 43: 1158–1165. https://doi.org/10.1007/s10439-014-1169-3
- Papaioannou TG, Manolesou D, Dimakakos E, et al., 2019, 3D bioprinting methods and techniques: Applications on artificial blood vessel fabrication. Acta Cardiol Sin, 35: 284. https://doi.org/10.6515/ACS.201905_35(3).20181115A
- Ning L, Chen X, 2017, A brief review of extrusion-based tissue scaffold bio-printing. Biotechnol J, 12: 1600671. https://doi.org/10.1002/BIOT.201600671
- Deo KA, Singh KA, Peak CW, et al., 2020, Bioprinting 101: Design, fabrication, and evaluation of cell-laden 3D bioprinted scaffolds. Tissue Eng Part A, 26: 318–338. https://doi.org/10.1089/TEN.TEA.2019.0298
- Kačarević ŽP, Rider PM, Alkildani S, et al., 2018, An introduction to 3D bioprinting: Possibilities, challenges and future aspects. Materials, 11: 2199. https://doi.org/10.3390/MA11112199
- Agarwal S, Saha S, Balla VK, et al., 2020, Current developments in 3D bioprinting for tissue and organ regeneration-a review. Front Mech Eng, 6: 589171. https://doi.org/10.3389/FMECH.2020.589171
- Gmeiner R, Deisinger U, Schönherr J, et al., 2015, Additive manufacturing of bioactive glasses and silicate bioceramics. J Ceram Sci, 6: 75–86. https://doi.org/10.4416/JCST2015-00001
- Sobral JM, Caridade SG, Sousa RA, et al., 2011, Three-dimensional plotted scaffolds with controlled pore size gradients: Effect of scaffold geometry on mechanical performance and cell seeding efficiency. Acta Biomater, 7: 1009–1018. https://doi.org/10.1016/j.actbio.2010.11.003
- Chen WH, Liu YY, Zhang FH, et al., 2015, Osteochondral integrated scaffolds with gradient structure by 3D printing forming. Int J Autom Comput, 12: 220–228. https://doi.org/10.1007/s11633-014-0853-y
- Thomas M, Willerth SM, 2017, 3-D bioprinting of neural tissue for applications in cell therapy and drug screening. Front Bioeng Biotechnol, 5: 69. https://doi.org/10.3389/fbioe.2017.00069
- Kundu J, Shim JH, Jang J, et al., 2015, An additive manufacturing‐based PCL-alginate-chondrocyte bioprinted scaffold for cartilage tissue engineering. J Tissue Eng Regen Med, 9: 1286–1297. https://doi.org/10.1002/term.1682
- Ma J, Lin L, Zuo Y, et al., 2019, Modification of 3D printed PCL scaffolds by PVAc and HA to enhance cytocompatibility and osteogenesis. RSC Adv, 9: 5338–5346. https://doi.org/10.1039/C8RA06652C
- Adhikari U, Rijal NP, Khanal S, et al., 2016, Magnesium and calcium-containing scaffolds for bone tissue regeneration. In: ASME International Mechanical Engineering Congress and Exposition. Vol. 50688. New York, United States: American Society of Mechanical Engineers. https://doi.org/10.1115/IMECE2016-66835
- Schneider CA, Rasband WS, Eliceiri KW, 2012, NIH Image to ImageJ: 25 years of image analysis. Nat Methods, 9: 671–675. https://doi.org/10.1038/nmeth.2089
- Huang B, Bártolo PJ, 2018, Rheological characterization of polymer/ceramic blends for 3D printing of bone scaffolds. Polym Test, 68: 365–378. https://doi.org/10.1016/j.polymertesting.2018.04.033
- Jiang W, Shi J, Li W, et al., 2012, Morphology, wettability, and mechanical properties of polycaprolactone/hydroxyapatite composite scaffolds with interconnected pore structures fabricated by a mini‐deposition system. Polym Eng Sci, 52: 2396–2402. https://doi.org/10.1002/pen.23193
- Jia J, Zhou H, Wei J, et al., 2010, Development of magnesium calcium phosphate biocement for bone regeneration. J R Soc Interface, 7: 1171–1180. https://doi.org/10.1098/rsif.2009.0559
- Fazeli N, Arefian E, Irani S, et al., 2021, 3D-printed PCL scaffolds coated with nanobioceramics enhance osteogenic differentiation of stem cells. ACS Omega, 6: 35284–35296. https://doi.org/10.1021/acsomega.1c04015
- Yang X, Wang Y, Zhou Y, et al., 2021, The application of polycaprolactone in three-dimensional printing scaffolds for bone tissue engineering. Polymers (Basel), 13: 2754. https://doi.org/10.3390/polym13162754
- Liu D, Nie W, Li D, et al., 2019, 3D printed PCL/SrHA scaffold for enhanced bone regeneration. Chem Eng J, 362: 269–279. https://doi.org/10.1016/j.cej.2019.01.015
- Park SA, Lee SJ, Seok JM, et al., 2018, Fabrication of 3D printed PCL/PEG polyblend scaffold using rapid prototyping system for bone tissue engineering application. J Bionic Eng, 15: 435–442. https://doi.org/10.1007/s42235-018-0034-8
- Park SA, Lee HJ, Kim KS, et al., 2018, In vivo evaluation of 3D-printed polycaprolactone scaffold implantation combined with β-TCP powder for alveolar bone augmentation in a beagle defect model. Materials, 11: 238. https://doi.org/10.3390/ma11020238
- Murphy CM, Haugh MG, O’brien FJ, 2010, The effect of mean pore size on cell attachment, proliferation and migration in collagen-glycosaminoglycan scaffolds for bone tissue engineering. Biomaterials, 31: 461–466. https://doi.org/10.1016/j.biomaterials.2009.09.063
- O’Brien FJ, Harley BA, Yannas IV, et al., 2005, The effect of pore size on cell adhesion in collagen-GAG scaffolds. Biomaterials, 26: 433–441. https://doi.org/10.1016/j.biomaterials.2004.02.052
- Wibowo A, Vyas C, Cooper G, et al., 2020, 3D printing of polycaprolactone-polyaniline electroactive scaffolds for bone tissue engineering. Materials, 13: 512. https://doi.org/10.3390/ma13030512
- Loh QL, Choong C, 2013, Three-dimensional scaffolds for tissue engineering applications: Role of porosity and pore size. Tissue Eng Part B Rev, 19: 485–502. https://doi.org/10.1089/ten.TEB.2012.0437
- Karageorgiou V, Kaplan D, 2005, Porosity of 3D biomaterial scaffolds and osteogenesis. Biomaterials, 26: 5474–5491. https://doi.org/10.1016/j.biomaterials.2005.02.002
- Edwards A, Jarvis D, Hopkins T, et al., 2015, Poly (ε-caprolactone)/keratin-based composite nanofibers for biomedical applications. J Biomed Mater Res B Appl Biomater, 103: 21–30. https://doi.org/10.1002/jbm.b.33172
- Gomes SR, Rodrigues G, Martins GG, et al., 2015, In vitro and in vivo evaluation of electrospun nanofibers of PCL, chitosan and gelatin: A comparative study. Mater Sci Eng C, 46: 348–358. https://doi.org/10.1016/j.msec.2014.10.051
- Wang J, Witte F, Xi T, et al., 2015, Recommendation for modifying current cytotoxicity testing standards for biodegradable magnesium-based materials. Acta Biomater, 21: 237–249. https://doi.org/10.1016/j.actbio.2015.04.011
- Dietrich E, Oudadesse H, Lucas‐Girot A, et al., 2009, In vitro bioactivity of melt‐derived glass 46S6 doped with magnesium. J Biomed Mater Res A, 88: 1087–1096. https://doi.org/10.1002/jbm.a.31901
- Sader MS, LeGeros RZ, Soares GA, 2009, Human osteoblasts adhesion and proliferation on magnesium-substituted tricalcium phosphate dense tablets. J Mater Sci Mater Med, 20: 521–527. https://doi.org/10.1007/s10856-008-3610-3