Evaluation of surgical fixation methods for the implantation of melt electrowriting-reinforced hyaluronic acid hydrogel composites in porcine cartilage defects
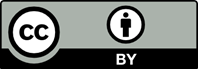
The surgical repair of articular cartilage remains an ongoing challenge in orthopedics. Tissue engineering is a promising approach to treat cartilage defects; however, scaffolds must (i) possess the requisite material properties to support neocartilage formation, (ii) exhibit sufficient mechanical integrity for handling during implantation, and (iii) be reliably fixed within cartilage defects during surgery. In this study, we demonstrate the reinforcement of soft norbornene-modified hyaluronic acid (NorHA) hydrogels via the melt electrowriting (MEW) of polycaprolactone to fabricate composite scaffolds that support encapsulated porcine mesenchymal stromal cell (pMSC, three donors) chondrogenesis and cartilage formation and exhibit mechanical properties suitable for handling during implantation. Thereafter, acellular MEW-NorHA composites or MEW-NorHA composites with encapsulated pMSCs and precultured for 28 days were implanted in full-thickness cartilage defects in porcine knees using either bioresorbable pins or fibrin glue to assess surgical fixation methods. Fixation of composites with either biodegradable pins or fibrin glue ensured implant retention in most cases (80%); however, defects treated with pinned composites exhibited more subchondral bone remodeling and inferior cartilage repair, as evidenced by micro-computed tomography (micro-CT) and safranin O/fast green staining, respectively, when compared to defects treated with glued composites. Interestingly, no differences in repair tissue were observed between acellular and cellularized implants. Additional work is required to assess the full potential of these scaffolds for cartilage repair. However, these results suggest that future approaches for cartilage repair with MEW-reinforced hydrogels should be carefully evaluated with regard to their fixation approach for construct retention and surrounding cartilage tissue damage.
Kalson NS, Gikas PD, Briggs TWR, 2010, Current strategies for knee cartilage repair. Int J Clin Pract, 64(10): 1444–1452. https://doi.org/10.1111/j.1742-1241.2010.02420.x
Everhart JS, Abouljoud MM, Flanigan DC, 2019, Role of full-thickness cartilage defects in knee osteoarthritis (OA) incidence and progression: Data from the OA Initiative. J Orthop Res, 37(1): 77–83. https://doi.org/10.1002/jor.24140
Carey JL, 2012, Fibrocartilage following microfracture is not as robust as native articular cartilage. J Bone Joint Surg, 94(11): e80. https://doi.org/10.2106/JBJS.L.00319
Martín AR, Patel JM, Zlotnick HM, et al., 2019, Emerging therapies for cartilage regeneration in currently excluded ‘red knee’ populations. NPJ Regen Med, 4(12): 1–11. https://doi.org/10.1038/s41536-019-0074-7
Johnstone B, Stoddart MJ, Im GI, 2020, Multi-disciplinary approaches for cell-based cartilage regeneration. J Orthop Res, 38(3): 463–472. https://doi.org/10.1002/jor.24458
Bothe F, Deubel AK, Hesse E, et al., 2019, Treatment of focal cartilage defects in minipigs with zonal chondrocyte/ mesenchymal progenitor cell constructs. Int J Mol Sci, 20(3): 1–17. https://doi.org/10.3390/ijms20030653
Diloksumpan P, Abinzano F, de Ruijter M, et al., 2021, The complexity of joint regeneration: How an advanced implant could fail by its in vivo proven bone component. J Trial Error, 2(1): 1–20. https://doi.org/10.36850/e3
Mancini IAD, Schmidt S, Brommer H, et al., 2020, A composite hydrogel-3D printed thermoplast osteochondral anchor as example for a zonal approach to cartilage repair: In vivo performance in a long-term equine model. Biofabrication, 12(3): 035028. https://doi.org/10.1088/1758-5090/ab94ce
Sennett ML, Friedman JM, Ashley BS, et al., 2021, Long term outcomes of biomaterial -mediated repair of focal cartilage defects in a large animal model. Eur Cell Mater, 41: 40–51. https://doi.org/10.22203/eCM.v041a04
Chu CR, Szczodry M, Bruno S, 2010, Animal models for cartilage regeneration. Tissue Eng Part B Rev, 16(1): 105–115. https://doi.org/10.1089=ten.teb.2009.0452
Ahern BJ, Parvizi J, Boston R, et al., 2009, Preclinical animal models in single site cartilage defect testing: A systematic review. Osteoarthr Cartil, 17(6): 705–713. https://doi.org/10.1016/j.joca.2008.11.008
Mancini IAD, Vindas Bolaños RA, Brommer H, et al., 2017, Fixation of hydrogel constructs for cartilage repair in the equine model: A challenging issue. Tissue Eng Part C Methods, 23(11): 804–814. https://doi.org/10.1089/ten.tec.2017.0200
Gotterbarm T, Breusch SJ, Schneider U, et al., 2008, The minipig model for experimental chondral and osteochondral defect repair in tissue engineering: Retrospective analysis of 180 defects. Lab Anim, 42(1): 71–82. https://doi.org/10.1258/la.2007.06029e
Drobnič M, Radosavljevič D, Ravnik D, et al., 2006, Comparison of four techniques for the fixation of a collagen scaffold in the human cadaveric knee. Osteoarthr Cartil, 14(4): 337–344. https://doi.org/10.1016/j.joca.2005.11.007
Duchow J, Hess T, Kohn D, 2000, Primary stability of press-fit-implanted osteochondral grafts: influence of graft size, repeated insertion, and harvesting technique. Am J Sports Med, 28(1): 24–27. https://doi.org/10.1177/03635465000280011601
Patel JM, Sennett ML, Martin AR, et al., 2020, Resorbable pins to enhance scaffold retention in a porcine chondral defect model. Cartilage, 13(2): 1676S–1687S. https://doi.org/10.1177/1947603520962568
Efe T, Füglein A, Heyse TJ, et al., 2012, Fibrin glue does not improve the fixation of press-fitted cell-free collagen gel plugs in an ex vivo cartilage repair model. Knee Sur Sports Traumatol Arthrosc, 20(2): 210–215. https://doi.org/10.1007/s00167-011-1571-4
Friedman JM, Sennett ML, Bonadio MB, et al., 2018, Comparison of fixation techniques of 3D-woven poly(∈-Caprolactone) scaffolds for cartilage repair in a weightbearing porcine large animal model. Cartilage, 9(4): 428–437. https://doi.org/10.1177/1947603517700953
Hunziker EB, Stähli A, 2008, Surgical suturing of articular cartilage induces osteoarthritis-like changes. Bone, 23(1): 1–7. https://doi.org/10.1016/j.joca.2008.01.009
Vikingsson L, Sancho-Tello M, Ruiz-Saurí A, et al., 2015, Implantation of a polycaprolactone scaffold with subchondral bone anchoring ameliorates nodules formation and other tissue alterations. Int J Artif Organs, 38(12): 659–666. https://doi.org/10.5301/ijao.5000457
Galarraga JH, Locke RC, Witherel CE, et al., 2022, Fabrication of MSC-laden composites of hyaluronic acid hydrogels reinforced with MEW scaffolds for cartilage repair. Biofabrication, 14(1): 014106. https://doi.org/10.1088/1758-5090/ac3acb
Castilho M, Hochleitner G, Wilson W, et al., 2018, Mechanical behavior of a soft hydrogel reinforced with three-dimensional printed microfibre scaffolds. Sci Rep, 8(1): 1245. https://doi.org/10.1038/s41598-018-19502-y
Galarraga JH, Kwon MY, Burdick JA, 2019, 3D bioprinting via an in situ crosslinking technique towards engineering cartilage tissue. Sci Rep, 9(1): 19987. https://doi.org/10.1038/s41598-019-56117-3
Kim M, Erickson IE, Choudhury M, et al., 2012, Transient exposure to TGF-β3 improves the functional chondrogenesis of MSC-laden hyaluronic acid hydrogels. J Mech Behav Biomed Mater, 11: 92–101. https://doi.org/10.1016/j.jmbbm.2012.03.006
Kwon MY, Vega SL, Gramlich WM, et al., 2018, Dose and timing of N-cadherin mimetic peptides regulate MSC chondrogenesis within hydrogels. Adv Healthc Mater, 7(9): 1701199. https://doi.org/10.1002/adhm.201701199
Zlotnick HM, Locke RC, Stoeckl BD, et al., 2021, Marked differences in local bone remodelling in response to different marrow stimulation techniques in a large animal. Eur Cell Mater, 41: 546–557. https://doi.org/10.22203/eCM.v041a35
Fisher MB, Belkin NS, Milby AH, et al., 2015, Cartilage repair and subchondral bone remodeling in response to focal lesions in a mini-pig model: Implications for tissue engineering. Tissue Eng Part A, 21(3–4): 850–860. https://doi.org/10.1089/ten.tea.2014.0384
Collins D, Simons B, 2020, Significantly delayed polyglactin 910 suture-related pseudoinfection in a yucatan pig. BMC Vet Res, 16(1): 1–7. https://doi.org/10.1186/s12917-020-02662-3
Smith G, Taylor J, Almqvist KF, et al., 2005, Arthroscopic assessment of cartilage repair: A validation study of 2 scoring systems. Arthroscopy, 21(12): 1462–1467. https://doi.org/10.1016/j.arthro.2005.09.007
Goebel L, Orth P, Müller A, et al., 2012, Experimental scoring systems for macroscopic articular cartilage repair correlate with the MOCART score assessed by a high-field MRI at 9.4 T - comparative evaluation of five macroscopic scoring systems in a large animal cartilage defect model. Osteoarthr Cartil, 20(9): 1046–1055. https://doi.org/10.1016/j.joca.2012.05.010
Erickson IE, Kestle SR, Zellars KH, et al., 2012, Improved cartilage repair via in vitro pre-maturation of MSC-seeded hyaluronic acid hydrogels. Biomed Mater, 7(2): 024110. https://doi.org/10.1088/1748-6041/7/2/024110
Mainil-Varlet P, Van Damme B, Nesic D, et al., 2010, A new histology scoring system for the assessment of the quality of human cartilage repair: ICRS II. Am J Sports Med, 38(5): 880–890. https://doi.org/10.1177/0363546509359068
Stoeckl BD, Zlotnick HM, Farrell MJ, et al., 2021, The porcine accessory carpal bone as a model for biologic joint replacement for trapeziometacarpal osteoarthritis. Acta Biomater, 129: 159–168. https://doi.org/10.1016/j.actbio.2021.05.011
Patel JM, Wise BC, Bonnevie ED, et al., 2019, A systematic review and guide to mechanical testing for articular cartilage tissue engineering. Tissue Eng Part C Methods, 25(10): 593–608. https://doi.org/10.1089/ten.tec.2019.0116
Moore AC, DeLucca JF, Elliott DM, et al., 2016, Quantifying cartilage contact modulus, tension modulus, and permeability with hertzian biphasic creep. J Tribol, 138(4): 1–7. https://doi.org/10.1115/1.4032917
Fisher MB, Belkin NS, Milby AH, et al., 2016, Effects of mesenchymal stem cell and growth factor delivery on cartilage repair in a mini-pig model. Cartilage, 7(2): 174–184. https://doi.org/10.1177/1947603515623030
Galarraga JH, Dhand AP, Enzmann BP, et al., 2023, Synthesis, characterization, and digital light processing of a hydrolytically degradable hyaluronic acid hydrogel. Biomacromolecules, 24(1): 413–425. https://doi.org/10.1021/acs.biomac.2c01218
Qayed M, Copland I, Galipeau J, 2017, Allogeneic Versus Autologous Mesenchymal Stromal Cells and Donor-To-Donor Variability. Elsevier Inc, Amsterdam. https://doi.org/10.1016/b978-0-12-802826-1.00004-0
Pfeifer CG, Fisher MB, Saxena V, et al., 2017, Age-dependent subchondral bone remodeling and cartilage repair in a minipig defect model. Tissue Eng Part C Methods, 23(11): 745–753. https://doi.org/10.1089/ten.tec.2017.0109
Brittberg M, Winalski CS, 2003, Evaluation of cartilage injuries and repair. J Bone Joint Surg Series A, 85(2): 58–69. https://doi.org/10.2106/00004623-200300002-00008
Mithoefer K, Mcadams T, Williams RJ, et al., 2009, Clinical efficacy of the microfracture technique for articular cartilage repair in the knee: An evidence-based systematic analysis. Am J Sports Med, 37(10): 2053–2063. https://doi.org/10.1177/0363546508328414
Donell S, 2019, Subchondral bone remodelling in osteoarthritis. EFORT Open Rev, 4(6): 221–229. https://doi.org/10.1302/2058-5241.4.180102
de Ruijter M, Diloksumpan P, Dokter I, et al., 2022, Pivotal importance of reinforcement of cartilage implants confirmed in challenging large animal model; presence of transplanted cells probably secondary, in Tissue Engineering Part A, Mary Ann Liebert, Inc., USA, S289–S290.
Mansour JM, 2009, Biomechanics of Cartilage. In: Lupash EJ, Klingler AM, Glover SA, eds. Kinesiology: The Mechanics and Pathomechanics of Human Movement. Second. Lippincott Williams & Wilkins, Philadelphia, 69–81.
Huang CY, Mow VC, Ateshian GA, 2001, The role of flow-independent viscoelasticity in the biphasic tensile and compressive responses of articular cartilage. J Biomech Eng, 123(5): 410–417. https://doi.org/10.1115/1.1392316
Zhang X, Jiang Y, Han L, et al., 2021, Biodegradable polymer hydrogel-based tissue adhesives: A review. Biosurf Biotribol, 7(4): 163–179. https://doi.org/10.1049/bsb2.12016
Nam S, Mooney D, 2021, Polymeric tissue adhesives. Chem Rev, 121(18): 11336–11384. https://doi.org/10.1021/acs.chemrev.0c00798
Kim M, Steinberg DR, Burdick JA, et al., 2019, Extracellular vesicles mediate improved functional outcomes in engineered cartilage produced from MSC/chondrocyte cocultures. Proc Natl Acad Sci USA, 116(5): 1569–1578. https://doi.org/10.1073/pnas.1815447116
Vega SL, Kwon MY, Song KH, et al., 2018, Combinatorial hydrogels with biochemical gradients for screening 3D cellular microenvironments. Nat Commun, 9: 614. https://doi.org/10.1038/s41467-018-03021-5
Patel JM, Saleh KS, Burdick JA, et al., 2019, Bioactive factors for cartilage repair and regeneration: Improving delivery, retention, and activity. Acta Biomater, 93: 222–238. https://doi.org/10.1016/j.actbio.2019.01.061