Silk fibroin–gelatin photo-crosslinked 3D-bioprinted hydrogel with MOF-methylene blue nanoparticles for infected wound healing
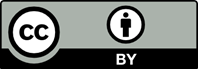
Photo-crosslinked hydrogel (PH) is an outstanding candidate for three-dimensional (3D) printing as a wound dressing because of its high efficiency in crosslinking and injectability. In this study, methylene blue (MB)-loaded UiO-66(Ce) nanoparticles (NPs) were synthesized to prevent drug self-aggregation and achieve the photodynamic therapy (PDT) effect for efficient antibacterial action. Then, a composite photocrosslinked silk fibroin (SF)/gelatin hydrogel loaded with MB@UiO-66(Ce) NPs (MB@UiO-66(Ce)/PH) was fabricated. The printability and the improvement of the mechanical properties of the hydrogel by the NPs were clarified. The hydrogel exhibited good biocompatibility and promoted the migration and proliferation of fibroblasts. With the PDT effect of MB@UiO-66(Ce) NPs, the hydrogel showed an excellent antibacterial effect, which became more pronounced as the concentration increased. In vivo study showed that the MB@UiO-66(Ce)/PH could fill the defects without gaps and accelerate the repair rate of full-thickness skin defects in mice. The MB@UiO-66(Ce)/PH with antibacterial properties and tissue healing-promoting ability provides a new strategy involving 3D bioprinting for preparing wound dressings.
Kechichian E, Ezzedine K, 2018, Vitamin D and the skin: An update for dermatologists. Am J Clin Dermatol, 19: 223–235.
Falcone M, De Angelis B, Pea F, et al., 2021, Challenges in the management of chronic wound infections. J Global Antimicrob Resist, 26: 140–147.
Kathawala MH, Ng WL, Liu D, et al., 2019, Healing of chronic wounds: An update of recent developments and future possibilities. Tissue Eng Part B Rev, 25: 429–444.
Simoes D, Miguel SP, Ribeiro MP, et al., 2018, Recent advances on antimicrobial wound dressing: A review. Eur J Pharm Biopharm, 127: 130–141.
Yang N, Zhu M, Xu G, et al., 2020, A near-infrared light-responsive multifunctional nanocomposite hydrogel for efficient and synergistic antibacterial wound therapy and healing promotion. J Mater Chem B, 8: 3908–3917.
White RJ, Cutting K, Kingsley A, 2006, Topical antimicrobials in the control of wound bioburden. Ostomy/Wound Manage, 52: 26–58.
Zhao G, Hochwalt PC, Usui ML, et al., 2010, Delayed wound healing in diabetic (db/db) mice with Pseudomonas aeruginosa biofilm challenge: A model for the study of chronic wounds. Wound Repair Regen, 18: 467–477.
Li S, Dong S, Xu W, et al., 2018, Antibacterial hydrogels. Adv Sci, 5: 1700527.
Dai X, Zhao Y, Yu Y, et al., 2017, Single continuous near-infrared laser-triggered photodynamic and photothermal ablation of antibiotic-resistant bacteria using effective targeted copper sulfide nanoclusters. ACS Appl Mater Interfaces, 9: 30470–30479.
Van Vlierberghe S, Dubruel P, Schacht E, 2011, Biopolymer-based hydrogels as scaffolds for tissue engineering applications: A review. Biomacromolecules, 12: 1387–1408.
Bilici C, Can V, Nöchel U, et al., 2016, Melt-processable shape-memory hydrogels with self-healing ability of high mechanical strength. Macromolecules, 49: 7442–7449.
Tavakoli S, Klar AS, 2020, Advanced hydrogels as wound dressings. Biomolecules, 10: 1169.
Koehler J, Brandl FP, Goepferich AM, 2018, Hydrogel wound dressings for bioactive treatment of acute and chronic wounds. Eur Polym J, 100: 1–11.
Gupta P, Vermani K, Garg S, 2002, Hydrogels: From controlled release to pH-responsive drug delivery. Drug Discov Today, 7: 569–579.
Augustine R, 2018, Skin bioprinting: A novel approach for creating artificial skin from synthetic and natural building blocks. Prog Biomater, 7: 77–92.
Ng WL, Huang X, Shkolnikov V, et al., 2022, Controlling droplet impact velocity and droplet volume: Key factors to achieving high cell viability in sub-nanoliter droplet-based bioprinting. Int J Bioprint, 8: 17, 424.
Ng WL, Lee JM, Zhou MM, et al., 2020, Vat polymerization-based bioprinting-process, materials, applications and regulatory challenges. Biofabrication, 12: 22, 022001.
Jiang T, Munguia-Lopez JG, Flores-Torres S, et al., 2019, Extrusion bioprinting of soft materials: An emerging technique for biological model fabrication. Appl Phys Rev, 6: 30, 011310.
Chocarro‐Wrona C, de Vicente J, Antich C, et al., 2021, Validation of the 1, 4‐butanediol thermoplastic polyurethane as a novel material for 3D bioprinting applications. Bioeng Transl Med, 6: e10192.
Vijayavenkataraman S, Lu W, Fuh J, 2016, 3D bioprinting of skin: A state-of-the-art review on modelling, materials, and processes. Biofabrication, 8: 032001.
Mandrycky C, Wang Z, Kim K, et al., 2016, 3D bioprinting for engineering complex tissues. Biotechnol Adv, 34: 422–434.
Axpe E, Oyen ML, 2016, Applications of alginate-based bioinks in 3D bioprinting. Int J Mol Sci, 17: 1976.
Shao Z, Vollrath F, 2002, Surprising strength of silkworm silk. Nature, 418: 741–741.
Müller M, Becher J, Schnabelrauch M, et al., 2015, Nanostructured pluronic hydrogels as bioinks for 3D bioprinting. Biofabrication, 7: 035006.
Wang Z, Abdulla R, Parker B, et al., 2015, A simple and high-resolution stereolithography-based 3D bioprinting system using visible light crosslinkable bioinks. Biofabrication, 7: 045009.
Melke J, Midha S, Ghosh S, et al., 2016, Silk fibroin as biomaterial for bone tissue engineering. Acta Biomater, 31: 1–16.
Kim SH, Kim DY, Lim TH, et al., 2020, Silk fibroin bioinks for digital light processing (DLP) 3D bioprinting. Bioinspired Biomater Adv Tissue Eng Regen Med, 1249: 53–66.
Kambe Y, Mizoguchi Y, Kuwahara K, et al., 2020, Beta-sheet content significantly correlates with the biodegradation time of silk fibroin hydrogels showing a wide range of compressive modulus. Polym Degrad Stab, 179: 109240.
Zheng A, Wang X, Xin X, et al., 2023, Promoting lacunar bone regeneration with an injectable hydrogel adaptive to the microenvironment. Bioact Mater, 21: 403–421.
Piluso S, Gomez DF, Dokter I, et al., 2020, Rapid and cytocompatible cell-laden silk hydrogel formation via riboflavin-mediated crosslinking. J Mater Chem B, 8: 9566– 9575.
Lee H, Shin D, Shin S, et al., 2020, Effect of gelatin on dimensional stability of silk fibroin hydrogel structures fabricated by digital light processing 3D printing. J Ind Eng Chem, 89: 119–127.
Billiet T, Gevaert E, De Schryver T, et al., 2014, The 3D printing of gelatin methacrylamide cell-laden tissue-engineered constructs with high cell viability. Biomaterials, 35: 49–62.
Planas O, Bresolí-Obach R, Nos J, et al., 2015, Synthesis, photophysical characterization, and photoinduced antibacterial activity of methylene blue-loaded amino-and mannose-targeted mesoporous silica nanoparticles. Molecules, 20: 6284–6298.
Lucky SS, Soo KC, Zhang Y, 2015, Nanoparticles in photodynamic therapy. Chem Rev, 115: 1990–2042.
Chen QW, Liu XH, Fan JX, et al., 2020, Self‐mineralized photothermal bacteria hybridizing with mitochondria‐targeted metal–organic frameworks for augmenting photothermal tumor therapy. Adv Funct Mater, 30: 1909806.
Tummers QR, Boonstra MC, Frangioni JV, et al., 2015, Intraoperative near-infrared fluorescence imaging of a paraganglioma using methylene blue: A case report. Int J Surg Case Rep, 6: 150–153.
Wang Z, Hu S, Yang J, et al., 2018, Nanoscale zr‐based MOFs with tailorable size and introduced mesopore for protein delivery. Adv Funct Mater, 28: 1707356.
Dhakshinamoorthy A, Navalón S, Asiri AM, et al., 2020, Gold‐nanoparticle‐decorated metal‐organic frameworks for anticancer therapy. ChemMedChem, 15: 2236–2256.
Gupta V, Mohiyuddin S, Sachdev A, et al., 2019, PEG functionalized zirconium dicarboxylate MOFs for docetaxel drug delivery in vitro. J Drug Deliv Sci Technol, 52: 846–855.
Kaur N, Tiwari P, Kapoor KS, et al., 2020, Metal–organic framework based antibiotic release and antimicrobial response: An overview. CrystEngComm, 22: 7513–7527.
Liu Y, Zhou L, Dong Y, et al., 2021, Recent developments on MOF-based platforms for antibacterial therapy. RSC Med Chem, 12: 915–928.
Lázaro IA, Forgan RS, 2019, Application of zirconium MOFs in drug delivery and biomedicine. Coord Chem Rev, 380: 230–259.
Ding Q, Xu Z, Zhou L, et al., 2022, A multimodal metal-organic framework based on unsaturated metal site for enhancing antitumor cytotoxicity through chemo-photodynamic therapy. J Colloid Interface Sci, 621: 180–194.
Zhang XY, Kang XN, Jin LJ, et al., 2018, Stimulation of wound healing using bioinspired hydrogels with basic fibroblast growth factor (bFGF). Int J Nanomed, 13: 3897– 3906.
Huang K, Li FP, Yuan K, et al., 2022, A MOF-armored zinc-peroxide nanotheranostic platform for eradicating drug resistant bacteria via image-guided and in situ activated photodynamic therapy. Appl Mater Today, 28: 12, 101513.
Xu ZQ, Qi XY, Bao MY, et al., 2023, Biomineralization inspired 3D printed bioactive glass nanocomposite scaffolds orchestrate diabetic bone regeneration by remodeling micromilieu. Bioact Mater, 25: 239–255.
Yoshinari M, Hayakawa T, Matsuzaka K, et al., 2006, Oxygen plasma surface modification enhances immobilization of simvastatin acid. Biomed Res, 27: 29–36.
Sun X, Song W, Teng L, et al., 2023, MiRNA 24-3p-rich exosomes functionalized DEGMA-modified hyaluronic acid hydrogels for corneal epithelial healing. Bioact Mater, 25: 640–656.
Zhang Z, Li W, Liu Y, et al., 2021, Design of a biofluid-absorbing bioactive sandwich-structured Zn–Si bioceramic composite wound dressing for hair follicle regeneration and skin burn wound healing. Bioact Mater, 6: 1910–1920.
Liang Y, He J, Guo B, 2021, Functional hydrogels as wound dressing to enhance wound healing. ACS Nano, 15: 12687– 12722.
Liang Y, Liang Y, Zhang H, et al., 2022, Antibacterial biomaterials for skin wound dressing. Asian J Pharm Sci, 17: 353–384.