3D bioprinting of corneal decellularized extracellular matrix: GelMA composite hydrogel for corneal stroma engineering
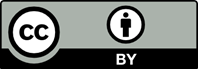
Millions of individuals across the world suffer from corneal stromal diseases that impair vision. Fortunately, three-dimensional (3D) bioprinting technology which has revolutionized the field of regenerative tissue engineering makes it feasible to create personalized corneas. In this study, an artificial cornea with a high degree of precision, smoothness, and programmable curvature was prepared by using digital light processing (DLP) 3D bioprinting in one piece with no support structure, and the construct was then confirmed by optical coherence tomography (OCT). On the basis of this approach, we developed a novel corneal decellularized extracellular matrix/gelatin methacryloyl (CECM-GelMA) bioink that can produce complex microenvironments with highly tunable mechanical properties while retaining high optical transmittance. Furthermore, the composite hydrogel was loaded with human corneal fibroblasts (hCFs), and in vitro experiments showed that the hydrogel maintained high cell viability and expressed core proteins. In vivo tests revealed that the hydrogel might promote epithelial regeneration, keep the matrix aligned, and restore clarity. This demonstrates how crucial a role CECM plays in establishing a favorable environment that encourages the transformation of cell function. Therefore, artificial corneas that can be rapidly customized have a huge potential in the development of in vitro corneal matrix analogs.
de By T, 2003, Shortage in the face of plenty: Improving the allocation of corneas for transplantation. Dev Ophthalmol, 36: 56–61.
Gain P, Jullienne R, He Z, et al., 2016, Global survey of corneal transplantation and eye banking. JAMA Ophthalmol, 134(2): 167–173.
Ruiz-Alonso S, Villate-Beitia I, Gallego I, et al., 2021, Current insights into 3D bioprinting: An advanced approach for eye tissue regeneration. Pharmaceutics, 13(3): 308.
Weng T, Zhang W, Xia Y, et al., 2021, 3D bioprinting for skin tissue engineering: Current status and perspectives. J Tissue Eng, 12: 20417314211028574.
Zhang X, Liu Y, Luo C, et al., 2021, Crosslinker-free silk/ decellularized extracellular matrix porous bioink for 3D bioprinting-based cartilage tissue engineering. Mater Sci Eng C, 118: 111388.
Auger FA, Gibot L, Lacroix D, 2013, The pivotal role of vascularization in tissue engineering. Annu Rev Biomed Eng, 15(1): 177–200.
Mao Q, Wang Y, Li Y, et al., 2020, Fabrication of liver microtissue with liver decellularized extracellular matrix (dECM) bioink by digital light processing (DLP) bioprinting. Mater Sci Eng C, 109: 110625.
Duarte Campos DF, Rohde M, Ross M, et al., 2019, Corneal bioprinting utilizing collagen-based bioinks and primary human keratocytes. J Biomed Mater Res A, 107(9): 1945–1953. https://doi.org/10.1002/jbm.a.36702.
Zhang B, Xue Q, Hu HY, et al., 2019, Integrated 3D bioprinting-based geometry-control strategy for fabricating corneal substitutes. J Zhejiang Univ Sci B, 20(12): 945–959. https://doi.org/10.1631/jzus.B1900190.
Li L, Lu C, Wang L, et al., 2018, Gelatin-based photocurable hydrogels for corneal wound repair. ACS Appl Mater Interfaces, 10(16): 13283–13292.
Yu C, Ma X, Zhu W, et al., 2019, Scanningless and continuous 3D bioprinting of human tissues with decellularized extracellular matrix. Biomaterials, 194: 1–13.
Zhang AP, Qu X, Soman P, et al., 2012, Rapid fabrication of complex 3D extracellular microenvironments by dynamic optical projection stereolithography. Adv Mater, 24(31): 4266–4270.
Miotto M, Gouveia RM, Ionescu AM, et al., 2019, 4D Corneal tissue engineering: achieving time-dependent tissue self-curvature through localized control of cell actuators. Adv Funct Mater, 29(8).10.1002/adfm.201807334. https://doi.org/10.1002/adfm.201807334.
Das S, Jang J, 2018, 3D bioprinting and decellularized ECM-based biomaterials for in vitro CV tissue engineering. J 3D Print Med, 2(2): 69–87.
Crapo PM, Gilbert TW, Badylak SF, 2011, An overview of tissue and whole organ decellularization processes. Biomaterials, 32(12): 3233–3243.
Nakayama KH, Batchelder CA, Lee CI, et al., 2010, Decellularized rhesus monkey kidney as a three-dimensional scaffold for renal tissue engineering. Tissue Eng Part A, 16(7): 2207–2216.
Badylak SF, Freytes DO, Gilbert TW, 2009, Extracellular matrix as a biological scaffold material: Structure and function. Acta Biomater, 5(1): 1–13.
Lawrence BD, Marchant JK, Pindrus MA, et al., 2009, Silk film biomaterials for cornea tissue engineering. Biomaterials, 30(7): 1299–1308.
Kilic Bektas C, Hasirci V, 2020, Cell loaded GelMA: HEMA IPN hydrogels for corneal stroma engineering. J Mater Sc, 31(1): 1–15.
He B, Wang J, Xie M, et al., 2022, 3D printed biomimetic epithelium/stroma bilayer hydrogel implant for corneal regeneration. Bioact Mater, 17: 234–247.
Badylak SF, 2007, The extracellular matrix as a biologic scaffold material. Biomaterials, 28(25): 3587–3593.
Uyanıklar M, Gunal G, Tevlek A, et al., 2019, Hybrid cornea: Cell laden hydrogel incorporated decellularized matrix. ACS Biomater Sci Eng, 6(1): 122–133.
Kim H, Park M-N, Kim J, et al., 2019, Characterization of cornea-specific bioink: High transparency, improved in vivo safety. J Tissue Eng, 10: 2041731418823382.
Yazdanpanah G, Shen X, Nguyen T, et al., 2022, A light‐curable and tunable extracellular matrix hydrogel for in situ suture‐free corneal repair. Adv Funct Mater, 32(24): 2113383.
Kim H, Jang J-H, Han W, et al., 2023, Extracellular matrix-based sticky sealants for scar-free corneal tissue reconstruction. Biomaterials, 292: 121941.
Shen X, Li S, Zhao X, et al., 2023, Dual-crosslinked regenerative hydrogel for sutureless long-term repair of corneal defect. Bioact Mater, 20(2023): 434–448.
Chen F, Le P, Lai K, et al., 2020, Simultaneous interpenetrating polymer network of collagen and hyaluronic acid as an in situ-forming corneal defect filler. Chem Mater, 32(12): 5208–5216.
Rafat M, Jabbarvand M, Sharma N, et al., 2023, Bioengineered corneal tissue for minimally invasive vision restoration in advanced keratoconus in two clinical cohorts. Nat Biotechnol, 41(1): 70–81.
Mao Q, Wang Y, Li Y, et al., 2020, Fabrication of liver microtissue with liver decellularized extracellular matrix (dECM) bioink by digital light processing (DLP) bioprinting. Mater Sci Eng C Mater Biol Appl, 109: 110625. https://doi.org/10.1016/j.msec.2020.110625
Basara G, Ozcebe SG, Ellis BW, et al., 2021, Tunable human myocardium derived decellularized extracellular matrix for 3D bioprinting and cardiac tissue engineering. Gels, 7(2): 70.
Petroll WM, Miron-Mendoza M, 2015, Mechanical interactions and crosstalk between corneal keratocytes and the extracellular matrix. Exp Eye Res, 133: 49–57.
Fernandez-Perez J, Ahearne M, 2019, Influence of biochemical cues in human corneal stromal cell phenotype. Curr Eye Res, 44(2): 135–146. 10.1080/02713683.2018.1536216
Harley BA, Leung JH, Silva EC, et al., 2007, Mechanical characterization of collagen–glycosaminoglycan scaffolds. Acta Biomater, 3(4): 463–474.
Gan Y, 2012, Continuum mechanics: Progress in fundamentals and engineering applications, 10.5772/2103(Chapter 4).
Daxer A, Misof K, Grabner B, et al., 1998, Collagen fibrils in the human corneal stroma: Structure and aging. Investig Ophthalmol Vis Sci, 39(3): 644–647.
Boettner EA, Wolter JR, 1962, Transmission of the ocular media. Investig Ophthalmol Vis Sci, 1: 776–783.
Peris-Martínez C, García-Domene MC, Penadés M, et al., 2021, Spectral transmission of the human corneal layers. J Clin Med, 10(19): 4490.
Kong B, Chen Y, Liu R, et al., 2020, Fiber reinforced GelMA hydrogel to induce the regeneration of corneal stroma. Nat Commun, 11(1): 1–12.
Nguyen AK, Goering PL, Reipa V, et al., 2019, Toxicity and photosensitizing assessment of gelatin methacryloyl-based hydrogels photoinitiated with lithium phenyl-2, 4, 6-trimethylbenzoylphosphinate in human primary renal proximal tubule epithelial cells. Biointerphases, 14(2): 021007.
Sun Y, Yu K, Nie J, et al., 2021, Modeling the printability of photocuring and strength adjustable hydrogel bioink during projection-based 3D bioprinting. Biofabrication, 13(3): 035032.
Duarte Campos DF, Rohde M, Ross M, et al., 2019, Corneal bioprinting utilizing collagen‐based bioinks and primary human keratocytes. J Biomed Mater Res Part A, 107(9): 1945–1953.
Kim BS, Das S, Jang J, et al., 2020, Decellularized extracellular matrix-based bioinks for engineering tissue-and organ-specific microenvironments. Chem Rev, 120(19): 10608–10661.
Ranjan R, Rawat K, Bohidar H, 2016, Interface versus bulk gelation and UCST in hydrophobically assembled TX-100 molecular gels. Colloids Surf, 499: 113–122.
Hatami-Marbini H, Etebu E, 2013, Hydration dependent biomechanical properties of the corneal stroma. Exp Eye Res, 116: 47–54.
Sharifi S, Sharifi H, Guild C, et al., 2021, Toward electron-beam sterilization of a pre-assembled Boston keratoprosthesis. Ocul Surf, 20: 176–184.
Petsche SJ, Chernyak D, Martiz J, et al., 2012, Depth-dependent transverse shear properties of the human corneal stroma. Investig Ophthalmol Vis Sci, 53(2): 873–880.
Formisano N, van der Putten C, Grant R, et al., 2021, Mechanical properties of bioengineered corneal stroma. Adv Healthc Mater, 10(20): 2100972.
Xu CC, Chan RW, Weinberger DG, et al., 2010, A bovine acellular scaffold for vocal fold reconstruction in a rat model. J Biomed Mater Res Part A, 92(1): 18–32.
Parekh A, Mantle B, Banks J, et al., 2009, Repair of the tympanic membrane with urinary bladder matrix. Laryngoscope, 119(6): 1206–1213.
Valentin JE, Turner NJ, Gilbert TW, et al., 2010, Functional skeletal muscle formation with a biologic scaffold. Biomaterials, 31(29): 7475–7484.
Bron A, 2001, The architecture of the corneal stroma. BMJ Publishing Group Ltd, London, 379–381.
Harrington DJ, 1996, Bacterial collagenases and collagen-degrading enzymes and their potential role in human disease. Infect Immun, 64(6): 1885–1891.
Aldave AJ, Kamal KM, Vo RC, et al., 2009, The Boston type I keratoprosthesis: Improving outcomes and expanding indications. Ophthalmology, 116(4): 640–651.
Ulag S, Uysal E, Bedir T, et al., 2021, Recent developments and characterization techniques in 3D printing of corneal stroma tissue. Polym Adv Technol, 32(8): 3287–3296.
Ahearne M, Fernández-Pérez J, 2020, Fabrication of Corneal Extracellular Matrix-Derived Hydrogels. Methods Mol Biol 2145: 159-168.10.1007/978-1-0716-0599-8_11.
Chakravarti S, Petroll WM, Hassell JR, et al., 2000, Corneal opacity in lumican-null mice: defects in collagen fibril structure and packing in the posterior stroma. Invest Ophthalmol Vis Sci, 41(11): 3365–3373.
Yamamoto M, Quantock AJ, Young RD, et al., 2012, A selective inhibitor of the Rho kinase pathway, Y-27632, and its influence on wound healing in the corneal stroma. Mol Vis, 18: 1727–1739.
Jester JV, Barry-Lane PA, Cavanagh HD, et al., 1996, Induction of α-smooth muscle actin expression and myofibroblast transformation in cultured corneal keratocytes. Cornea, 15(5): 505–516.
Wang F, Shi W, Li H, et al., 2020, Decellularized porcine cornea-derived hydrogels for the regeneration of epithelium and stroma in focal corneal defects. Ocul Surf, 18(4): 748–760.
Scott S-G, Jun AS, Chakravarti S, 2011, Sphere formation from corneal keratocytes and phenotype specific markers. Exp Eye Res, 93(6): 898–905.