Designing an anatomical contour titanium 3D-printed oblique lumbar interbody fusion cage with porous structure and embedded fixation screws for patients with osteoporosis
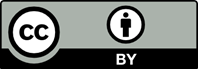
This study aimed to design an anatomical contour metal three-dimensional (3D)- printed oblique lateral lumbar interbody fusion (OLIF) cage with porous (lattices) structure and embedded screw fixation to enhance bone ingrowth to reduce the risk of cage subsidence and avoid the stress-shielding effect. Finite element (FE) analysis and weight topology optimization (WTO) were used to optimize the structural design of the OLIF cage based on the anatomical contour morphology of patients with osteoporosis. Two oblique embedded fixation screws and lattice design with 65% porosity and average pore size of 750 μm were equipped with the cage structure. The cage was fabricated via metal 3D printing, and static/dynamic compression and compressive-shear tests were performed in accordance with the ASTM F2077-14 standard to evaluate its mechanical resistance. On FE analysis, the OLIF cage with embedded screw model had the most stability, lowest stress values on the endplate, and uniform stress distribution versus standalone cage and fixed with lateral plate under extension, lateral flexion, and rotation. The fatigue test showed that the stiffnesses/endurance limits (pass 5 million dynamic test) were 16,658 N/mm/6000 N for axial load and 19,643 N/mm/2700 N for compression shear. In conclusion, an OLIF cage with embedded fixation screws can be designed by integrating FE and WTO analysis based on the statistical results of endplate morphology. This improves the stability of the OLIF cage to decrease endplate destruction. The complex contour and lattice design of the OLIF cage need to be manufactured via metal 3D printing; the dynamic axial compression and compressive-shear strengths are greater than that of the U.S. Food and Drug Administration (FDA) standard.
Orita S, Inage K, Furuya T, et al., 2017, Oblique lateral interbody fusion (OLIF): Indications and techniques. Oper Tech Orthop, 27(4): 223–230.
Zeng ZY, Xu ZW, He DW, et al., 2018, Complications and prevention strategies of oblique lateral interbody fusion technique. Orthop Surg, 10(2): 98–106.
He W, He D, Sun Y, et al., 2020, Standalone oblique lateral interbody fusion vs. combined with percutaneous pedicle screw in spondylolisthesis. BMC Musculoskelet Disord, 21(1): 1–9.
Choi YH, Kwon SW, Moon JH, et al., 2017, Lateral lumbar interbody fusion and in situ screw fixation for rostral adjacent segment stenosis of the lumbar spine. J Korean Neurosurg Soc, 60(6): 755.
Xie T, Wang C, Yang Z, et al., 2020, Minimally invasive oblique lateral lumbar interbody fusion combined with anterolateral screw fixation for lumbar degenerative disc disease. World Neurosurg, 135: e671–e678.
Hu Z, He D, Gao J, et al., 2021, The influence of endplate morphology on cage subsidence in patients with stand-alone oblique lateral lumbar interbody fusion (OLIF). Global Spine J, 13(1): 97–103.
Siu TL, Rogers JM, Lin K, et al., 2018, Custom-made titanium 3-dimensional printed interbody cages for treatment of osteoporotic fracture–related spinal deformity. World Neurosurg, 111: 1–5.
Huiskes R, Ruimerman R, Van Lenthe GH, et al., 2000, Effects of mechanical forces on maintenance and adaptation of form in trabecular bone. Nature, 405(6787): 704–706.
Tartara F, Bongetta D, Pilloni G, et al. 2020, Custom-made trabecular titanium implants for the treatment of lumbar degenerative discopathy via ALIF/XLIF techniques: rationale for use and preliminary results. Eur Spine J, 29(2): 314–320.
Taniguchi N, Fujibayashi S, Takemoto M, et al., 2016, Effect of pore size on bone ingrowth into porous titanium implants fabricated by additive manufacturing: An in vivo experiment. Mater Sci Eng C, 59: 690–701.
Li F, Li J, Xu G, et al., 2015, Fabrication, pore structure and compressive behavior of anisotropic porous titanium for human trabecular bone implant applications. J Mech Behav Biomed Mater, 46: 104–114.
Chang B, Song W, Han T, et al., 2016, Influence of pore size of porous titanium fabricated by vacuum diffusion bonding of titanium meshes on cell penetration and bone ingrowth. Acta Biomater, 33: 311–321.
Zhang Z, Fogel GR, Liao Z, et al., 2018, Biomechanical analysis of lumbar interbody fusion cages with various lordotic angles: A finite element study. Comput Methods Biomech Biomed Eng, 21(3): 247–254.
Xiao Z, Wang L, Gong H, et al., 2011, A non-linear finite element model of human L4-L5 lumbar spinal segment with three-dimensional solid element ligaments. Theor App Mech Lett, 1(6): 064001.
Guo HZ, Tang YC, Guo DQ, et al., 2020, Stability evaluation of oblique lumbar interbody fusion constructs with various fixation options: A finite element analysis based on three-dimensional scanning models. World Neurosurg, 138: e530– e538.
Huang SF, Chang CM, Liao CY, et al., 2022, Biomechanical evaluation of an osteoporotic anatomical 3D printing posterior lumbar interbody fusion cage with internal lattice design based on weighted topology optimization. Int J Bioprint, 9(3): 410–421.
Chazal J, Tanguy A, Bourges M, et al., 1985, Biomechanical properties of spinal ligaments and a histological study of the supraspinal ligament in traction. J Biomech, 18(3): 167–176.
Zhong ZC, Wei SH, Wang JP, et al., 2006, Finite element analysis of the lumbar spine with a new cage using a topology optimization method. Med Eng Phys, 28(1): 90–98.
Yamamoto ISAO, Panjabi MM, Crisco TREY, et al., 1989, Three dimensional movements of the whole lumbar spine and lumbosacral joint. Spine, 14(11): 1256–1260.
Schmoelz W, Huber JF, Nydegger T, et al., 2003, Dynamic stabilization of the lumbar spine and its effects on adjacent segments: an in vitro experiment. Clin Spine Surg, 16(4): 418–423.
Chen SH, Tai CL, Lin CY, et al., 2008, Biomechanical comparison of a new stand-alone anterior lumbar interbody fusion cage with established fixation techniques – a three-dimensional finite element analysis. BMC Musculoskelet Disord, 9(1): 1–10.
Liao CY, Chien CL, Pu TW, et al., 2022, Assessment of lumbar vertebrae morphology by computed tomography in older adults with osteoporosis. Curr Med Imaging, 18(11): 1195–1203.
Cobian D, Heiderscheit B, Daehn N, et al., 2011, Comparison of daily motion of the cervical and lumbar spine to ASTM F2423-11 and ISO 18192-1.2011 standard testing. J ASTM Int, 9(1): 1–10.
Li CH, Wu CH, Lin CL, 2020, Design of a patient-specific mandible reconstruction implant with dental prosthesis for metal 3D printing using integrated weighted topology optimization and finite element analysis. J Mech Behav Biomed Mater, 105: 103700.
Taniguchi N, Fujibayashi S, Takemoto M, et al., 2016, Effect of pore size on bone ingrowth into porous titanium implants fabricated by additive manufacturing: an in vivo experiment. Mater Sci Eng C, 59: 690–701.
Implants for surgery — Preclinical mechanical assessment of spinal implants and particular requirements —Part 2: Spinal intervertebral body fusion devices. ISO 23089–2:2021.
Yang J, Cai H, Lv J, et al., 2014, In vivo study of a self-stabilizing artificial vertebral body fabricated by electron beam melting. Spine, 39(8): e486–e492.
Wu SH, Li Y, Zhang YQ, et al., 2013, Porous titanium-6 aluminum-4 vanadium cage has better osseointegration and less micromotion than a poly-ether-ether-ketone cage in sheep vertebral fusion. Artif Organs, 37(12): e191–e201.
Chon CS, Ko CW, Kim HS, 2015, Development of anterior lumbar interbody fusion (ALIF) PEEK cage based on the Korean lumbar anatomical information. Int J Biomed Biol Eng, 9(2): 151–154.
Lim KM, Park TH, Lee SJ, et al., 2019, Design and biomechanical verification of additive manufactured composite spinal cage composed of porous titanium cover and PEEK body. Appl Sci, 9(20): 4258.