A sturgeon cartilage extracellular matrix-derived bioactive bioink for tissue engineering applications
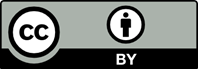
Three-dimensional (3D) bioprinting provides a promising strategy for tissue and organ engineering, and extracellular matrix (ECM)-derived bioinks greatly facilitate its applications in these areas. Decellularized sturgeon cartilage ECM (dSC-ECM)- derived bioinks for cartilage tissue engineering were fabricated with methacrylatemodified dSC-ECM (dSC-ECMMA) and sericin methacrylate (SerMA), which optimizedthe mechanical properties of their solidified hydrogels.dSC-ECM induces chondrocytes to form cell clusters and subsequently reduces their proliferation, but the proliferation of encapsulated chondrocytes was normal in solidified dSCECM-5 bioink samples, which contain 5 mg/mL dSC-ECMMA. Hence, this bioink was selected for further investigation. Lyophilized dSC-ECM-5 hydrogels showed connected pore microstructure, which is suitable for cell migration and nutrients transportation. ThisdSC-ECM-5 bioink exhibited high fidelity and good printability by testing via a 3D bioprinting system, and the chondrocytes loaded in printed hydrogel products were viable and able to grow, following incubation, in the cell culture medium. Solidified dSC-ECM-5 and SerMA bioinks loaded with chondrocytes were subcutaneously implanted into nude mice for 4 weeks to test the suitability of the bioink for cartilage tissue engineering. Compared to the SerMA bioink, the dSCECM-5 bioink significantly enhanced cartilage tissue regeneration and maturation in vivo, suggesting the potential of this bioink to be applied in cartilage tissue engineering in the future.
Daly AC, Prendergast ME, Hughes AJ, et al., 2021, Bioprinting for the biologist. Cell, 184(1):18–32. https://doi.org/10.1016/j.cell.2020.12.002
Spencer AR, Shirzaei Sani E, Soucy JR, et al., 2019, Bioprinting of a cell-laden conductive hydrogel composite. ACS Appl Mater Interfaces, 11(34):30518–30533. https://doi.org/10.1021/acsami.9b07353
Chen N, Zhu K, Zhang YS, et al., 2019, Hydrogel bioink with multilayered interfaces improves dispersibility of encapsulated cells in extrusion bioprinting.ACS Appl Mater Interfaces, 11(34):30585–30595. https://doi.org/10.1021/acsami.9b09782
Yang H, Yang KH, Narayan RJ, et al., 2021, Laser-based bioprinting for multilayer cell patterning in tissue engineering and cancer research. Essays Biochem, 65(3): 409–416. https://doi.org/10.1042/EBC20200093
Moroni L, Burdick JA, Highley C, et al., 2018, Biofabrication strategies for 3D in vitro models and regenerative medicine. Nat Rev Mater, 3(5):21–37. https://doi.org/10.1038/s41578-018-0006-y
Valot L, Martinez J, Mehdi A, et al., 2019, Chemical insights into bioinks for 3D printing. Chem Soc Rev, 48(15): 4049–4086. https://doi.org/10.1039/c7cs00718c
Chimene D, Kaunas R, Gaharwar AK, 2020, Hydrogel bioink reinforcement for additive manufacturing: A focused review of emerging strategies. Adv Mater, 32(1):1902026. https://doi.org/10.1002/adma.201902026
Ahlfeld T, Guduric V, Duin S, et al., 2020, Methylcellulose: Aversatile printing material that enables biofabrication of tissue equivalents with high shape fidelity. Biomater Sci-UK, 8(8):2102–2110. https://doi.org/10.1039/d0bm00027b
Bandyopadhyay A, Ghosh S, Boccaccini AR, et al., 2021, 3D printing of biomedical materials and devices. J Mater Res, 36(19):3713–3724. https://doi.org/10.1557/s43578-021-00407-y
Oliveira EP, Malysz-Cymborska I, Golubczyk D, et al., 2019, Advances in bioinks and in vivo imaging of biomaterials for CNS applications. Acta Biomater, 95: 60–72. https://doi.org/10.1016/j.actbio.2019.05.006
Luo C, Xie R, Zhang J, et al., 2020, Low-temperature three-dimensional printing of tissue cartilage engineered with gelatin methacrylamide. Tissue Eng Part C Methods, 26(6):306–316. https://doi.org/10.1089/ten.TEC.2020.0053
Beck EC, Barragan M, Tadros MH, et al., 2016, Approaching the compressive modulus of articular cartilage with a decellularized cartilage-based hydrogel. Acta Biomater, 38: 94–105. https://doi.org/10.1016/j.actbio.2016.04.019
Masaeli E, Nasr-Esfahani MH, 2021, An in vivo evaluation of induced chondrogenesis by decellularized extracellular matrix particles. J Biomed Mater Res A, 109(5):627–636. https://doi.org/10.1002/jbm.a.37047
Sun WY, Yang YY, Wang L, et al., 2022, Utilization of an acellular cartilage matrix-based photocrosslinking hydrogel for tracheal cartilage regeneration and circumferential tracheal repair. Adv Funct Mater, 32(31):202201257. https://doi.org/10.1002/adfm.
Yang Z, Shi Y, Wei X, et al., 2010, Fabrication and repair of cartilage defects with a novel acellular cartilage matrix scaffold. Tissue Eng Part C Methods, 16(5):865–876. https://doi.org/10.1089/ten.TEC.2009.0444
Pati F, Jang J, Ha DH, et al., 2014, Printing three-dimensional tissue analogues with decellularized extracellular matrix bioink. Nat Commun, 5: 3935. https://doi.org/10.1038/ncomms4935
Bhattacharya R, Das P, Joardar SN, et al., 2019, Novel decellularized animal conchal cartilage graft for application in human patient. J Tissue Eng Regen Med, 13(1):46–57. https://doi.org/10.1002/term.2767
Das P, Singh YP, Joardar SN, et al., 2019, Decellularized caprine conchal cartilage toward repair and regeneration of damaged cartilage. ACS Appl Bio Mater, 2(5):2037–2049. https://doi.org/10.1021/acsabm.9b00078
Viegas CSB, Conceicao N, Fazenda C, et al., 2010, Expression of Gla-rich protein (GRP) in newly developed cartilage-derived cell cultures from sturgeon (Acipenser naccarii). J Appl Ichthyol, 26(2):214–218. https://doi.org/10.1111/j.1439-0426.2010.01408.x
Li Y, Chen W, Dai Y, et al., 2021, Decellularized sturgeon cartilage extracellular matrix scaffold inhibits chondrocyte hypertrophy in vitro and in vivo. J Tissue Eng Regen Med, 15(8):732–744. https://doi.org/10.1002/term.3222
Qi C, Liu J, Jin Y, et al., 2018, Photo-crosslinkable, injectable sericin hydrogel as 3D biomimetic extracellular matrix for minimally invasive repairing cartilage. Biomaterials, 163: 89–104. https://doi.org/10.1016/j.biomaterials.2018.02.016
Chen W, Xu Y, Li H, et al., 2020, Tanshinone IIA delivery silk fibroin scaffolds significantly enhance articular cartilage defect repairing via promoting cartilage regeneration. ACS Appl Mater Interfaces, 12(19):21470–21480. https://doi.org/10.1021/acsami.0c03822
Kim H, Kang B, Cui XL, et al., 2021, Light-activated decellularized extracellular matrix-based bioinks for volumetric tissue analogs at the centimeter scale. Adv Funct Mater, 31(32). https://doi.org/10.1002/adfm.202011252
Zhao H, He LY, 2022, Fabrication of neuroprotective silk-sericin hydrogel: Potential neuronal carrier for the treatment and care of ischemic stroke. J Exp Nanosci, 17(1):362–376. https://doi.org/10.1080/17458080.2022.2075545
Xue H, ZhangZH, Lin Z, et al., 2022, Enhanced tissue regeneration through immunomodulation of angiogenesis and osteogenesis with a multifaceted nanohybrid modified bioactive scaffold. Bioact Mater, 18: 552–568. https://doi.org/10.1016/j.bioactmat.2022.05.023
Huang Y, Meng X, Zhou Z, et al., 2022, A naringin-derived bioink enhances the shape fidelity of 3D bioprinting and efficiency of cartilage defect repair. J Mater Chem B, 10(36):7030–7044. https://doi.org/10.1039/d2tb01247b
Du Z, Li N, Hua Y, et al., 2017, Physiological pH-dependent gelation for 3D printing based on the phase separation of gelatin and oxidized dextran. Chem Commun (Camb), 53(97):13023–13026. https://doi.org/10.1039/c7cc08225h
Bae M, Hwang D, Ko MK, et al., 2021, Neural stem cell delivery using brain-derived tissue-specific bioink for recovering from traumatic brain injury. Biofabrication, 13(4):044110. https://doi.org/10.1088/1758-5090/ac293f
Wei L, Qin S, Ye Y, et al., 2022, Chondrogenic potential of manganese-loaded composite scaffold combined with chondrocytes for articular cartilage defect. J Mater Sci Mater Med, 33(10):74. https://doi.org/10.1007/s10856-022-06695-y
Schuckert KH, Jopp S, Osadnik M, 2010, Modern bone regeneration instead of bone transplantation: Acombination of recombinant human bone morphogenetic protein-2