Photocurable 3D-printed PMBG/TCP biphasic scaffold mimicking vasculature for bone regeneration
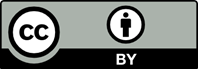
Mesoporous bioglass (MBG) with excellent osteointegration, osteoinduction, and biodegradability is a promising material for bone regeneration. However, its clinical application is hindered by complex processing and a lack of personalization, low mechanical strength, and uncontrollable degradation rate. In this study, we developed a double-bond-functionalized photocurable mesoporous bioglass (PMBG) sol that enabled ultrafast photopolymerization within 5 s. By further integrating nanosized tricalcium phosphate (TCP) particles through three-dimensional (3D) printing technology, we fabricated personalized and highly porous PMBG/TCP biphasic scaffolds. The mechanical properties and degradation behavior of the scaffolds were regulated by varying the amount of TCP doping. In vitro and in vivo experiments verified that PMBG/TCP scaffolds slowly released SiO4 4- and Ca2+, forming a vascularized bone regeneration microenvironment within the fully interconnected pore channels of the scaffold. This microenvironment promoted angiogenesis and accelerated bone tissue regeneration. Overall, this work demonstrates the solution to the problem of complex processing and lack of personalization in bioglass scaffolds, and the developed PMBG/TCP biphasic scaffold is an ideal material for bone regeneration applications with broad clinical prospects.
Petersen A, Princ A, Korus G, et al., 2018, A biomaterial with a channel-like pore architecture induces endochondral healing of bone defects. Nat Commun, 9(1): 4430. https://doi.org/10.1038/s41467-018-06504-7
Okuchi Y, Reeves J, Ng SS, et al., 2021, Wnt-modified materials mediate asymmetric stem cell division to direct human osteogenic tissue formation for bone repair. Nat Mater, 20(1): 108–118. https://doi.org/10.1038/s41563-020-0786-5
Salhotra A, Shah HN, Levi B, et al., 2020, Mechanisms of bone development and repair. Nat Rev Mol Cell Biol, 21(11): 696–711. https://doi.org/10.1038/s41580-020-00279-w
Zhao R, Shang T, Yuan B, et al., 2022, Osteoporotic bone recovery by a bamboo-structured bioceramic with controlled release of hydroxyapatite nanoparticles. Bioact Mater, 17: 379–393. https://doi.org/10.1016/j.bioactmat.2022.01.007
Hao J, Bai B, Ci Z, et al., 2022, Large-sized bone defect repair by combining a decalcified bone matrix framework and bone regeneration units based on photo-crosslinkable osteogenic microgels. Bioact Mater, 14: 97–109. https://doi.org/10.1016/j.bioactmat.2021.12.013
Feng B, Zhang M, Qin C, et al., 2023, 3D printing of conch-like scaffolds for guiding cell migration and directional bone growth. Bioact Mater, 22: 127–140. https://doi.org/10.1016/j.bioactmat.2022.09.014
Liu H, Du Y, St-Pierre J-P, et al., 2020, Bioenergetic-active materials enhance tissue regeneration by modulating cellular metabolic state. Sci Adv, 6(13): eaay7608. https://doi.org/10.1126/sciadv.aay7608
Yu M, Du Y, Han Y, et al., 2020, Biomimetic elastomeric bioactive siloxane-based hybrid nanofibrous scaffolds with miRNA activation: A joint physico-chemical-biological strategy for promoting bone regeneration. Adv Funct Mater, 30(4): 1906013. https://doi.org/10.1002/adfm.201906013
Chen Q, Yu S, Zhang D, et al., 2019, Impact of antifouling PEG layer on the performance of functional peptides in regulating cell behaviors. J Am Chem Soc, 141(42): 16772–16780. https://doi.org/10.1021/jacs.9b07105
Zhang X, Chen X, Hong H, et al., 2022, Decellularized extracellular matrix scaffolds: Recent trends and emerging strategies in tissue engineering. Bioact Mater, 10: 15–31. https://doi.org/10.1016/j.bioactmat.2021.09.014
Chen X, Wang M, Chen F, et al., 2020, Correlations between macrophage polarization and osteoinduction of porous calcium phosphate ceramics. Acta Biomater, 103: 318–332. https://doi.org/10.1016/j.actbio.2019.12.019
Zhao F, Yang Z, Xiong H, et al., 2023, A bioactive glass functional hydrogel enhances bone augmentation via synergistic angiogenesis, self-swelling and osteogenesis. Bioact Mater, 22: 201–210. https://doi.org/10.1016/j.bioactmat.2022.09.007
Aleman J, Kilic T, Mille LS, et al., 2021, Microfluidic integration of regeneratable electrochemical affinity-based biosensors for continual monitoring of organ-on-a-chip devices. Nat Protoc, 16(5): 2564–2593. https://doi.org/10.1038/s41596-021-00511-7
Zhao H, Liang G, Liang W, et al., 2020, In vitro and in vivo evaluation of the pH-neutral bioactive glass as high performance bone grafts. Mater Sc Eng C, 116: 111249. https://doi.org/10.1016/j.msec.2020.111249
Zhao F, Xie W, Zhang W, et al., 2018, 3D printing nanoscale bioactive glass scaffolds enhance osteoblast migration and extramembranous osteogenesis through stimulating immunomodulation. Adv Healthc Mater, 7(16): e1800361. https://doi.org/10.1002/adhm.201800361
Zheng X, Zhang X, Wang Y, et al., 2021, Hypoxia-mimicking 3D bioglass-nanoclay scaffolds promote endogenous bone regeneration. Bioact Mater, 6(10): 3485–3495. https://doi.org/10.1016/j.bioactmat.2021.03.011
Zhi W, Wang X, Sun D, et al., 2022, Optimal regenerative repair of large segmental bone defect in a goat model with osteoinductive calcium phosphate bioceramic implants. Bioact Mater, 11: 240–253. https://doi.org/10.1016/j.bioactmat.2021.09.024
Koons GL, Diba Mand Mikos AG, 2020, Materials design for bone-tissue engineering. Nat Rev Mater, 5(8): 584–603. https://doi.org/10.1038/s41578-020-0204-2
Richter RF, Ahlfeld T, Gelinsky M, et al., 2023, Composites consisting of calcium phosphate cements and mesoporous bioactive glasses as a 3D plottable drug delivery system. Acta Biomater, 156: 146–157. https://doi.org/10.1016/j.actbio.2022.01.034
Wu L, Pei X, Zhang B, et al., 2023, 3D-printed HAp bone regeneration scaffolds enable nano-scale manipulation of cellular mechanotransduction signals. Chem Eng J, 455: 140699. https://doi.org/10.1016/j.cej.2022.140699
Autefage H, Allen F, Tang HM, et al., 2019, Multiscale analyses reveal native-like lamellar bone repair and near perfect bone-contact with porous strontium-loaded bioactive glass. Biomaterials, 209: 152–162. https://doi.org/10.1016/j.biomaterials.2019.03.035
Zhang M, Zhai X, Ma T, et al., 2023, Sequential therapy for bone regeneration by cerium oxide-reinforced 3D-printed bioactive glass scaffolds. ACS Nano, 17(5): 4433–4444. https://doi.org/10.1021/acsnano.2c09855
Li S, Zhang L, Liu C, et al., 2023, Spontaneous immunomodulation and regulation of angiogenesis and osteogenesis by Sr/Cu-borosilicate glass (BSG) bone cement to repair critical bone defects. Bioact Mater, 23: 101–117. https://doi.org/10.1016/j.bioactmat.2022.10.021
Zhu H, Zheng K, Boccaccini AR, 2021, Multi-functional silica-based mesoporous materials for simultaneous delivery of biologically active ions and therapeutic biomolecules. Acta Biomater, 129: 1–17. https://doi.org/10.1016/j.actbio.2021.05.007
Zhu H, Monavari M, Zheng K, et al., 2022, 3D bioprinting of multifunctional dynamic nanocomposite bioinks incorporating Cu-doped mesoporous bioactive glass nanoparticles for bone tissue engineering. Small, 18(12): e2104996. https://doi.org/10.1002/smll.202104996
Niu HY, Ma YF, Wu GY, et al., 2021, Multicellularity-interweaved bone regeneration of BMP-2-loaded scaffold with orchestrated kinetics of resorption and osteogenesis (vol 216, 119216, 2019). Biomaterials, 216: 119216. https://doi.org/10.1016/j.biomaterials.2020.120376
Zhou Y, Wu C, Chang J, 2019, Bioceramics to regulate stem cells and their microenvironment for tissue regeneration. Mater Today, 24(C): 41–56. https://doi.org/10.1016/j.mattod.2018.07.016
Ma H, Feng C, Chang J, et al., 2018, 3D-printed bioceramic scaffolds: From bone tissue engineering to tumor therapy. Acta Biomater, 79: 37–59. https://doi.org/10.1016/j.actbio.2018.08.026
Tang F, Li J, Xie W, et al., 2021, Bioactive glass promotes the barrier functional behaviors of keratinocytes and improves the Re-epithelialization in wound healing in diabetic rats. Bioact Mater, 6(10): 3496–3506. https://doi.org/10.1016/j.bioactmat.2021.02.041
Schumacher M, Habibovic P, van Rijt S, 2021, Mesoporous bioactive glass composition effects on degradation and bioactivity. Bioact Mater, 6(7): 1921–1931. https://doi.org/10.1016/j.bioactmat.2020.12.007
Chai Y, Lin D, Ma Y, et al., 2017, RhBMP-2 loaded MBG/ PEGylated poly(glycerol sebacate) composite scaffolds for rapid bone regeneration. J Mater Chem B, 5(24): 4633– 4647. https://doi.org/10.1039/c7tb00505a
Ding X, Shi J, Wei J, et al., 2021, A biopolymer hydrogel electrostatically reinforced by amino-functionalized bioactive glass for accelerated bone regeneration. Sci Adv, 7(50): eabj7857. https://doi.org/10.1126/sciadv.abj7857
Lin D, Cai B, Wang L, et al., 2021, A viscoelastic PEGylated poly(glycerol sebacate)-based bilayer scaffold for cartilage regeneration in full-thickness osteochondral defect (vol 253, 120095, 2020). Biomaterials, 253: 120095. https://doi.org/10.1016/j.biomaterials.2020.120618
Ma LL, Zhou YL, Zhang ZWB, et al., 2020, Multifunctional bioactive Nd-Ca-Si glasses for fluorescence thermometry, photothermal therapy, and burn tissue repair. Sci Adv, 6(32): eabb1311. https://doi.org/10.1126/sciadv.abb1311
Liu Y, Ma Y, Zhang J, et al., 2017, MBG-modified beta-TCP scaffold promotes mesenchymal stem cells adhesion and osteogenic differentiation via a FAK/MAPK signaling pathway. ACS Appl Mater Interfaces, 9(36): 30283–30296. https://doi.org/10.1021/acsami.7b02466
Zhou M, Li B, Li N, et al., 2022, Regulation of Ca2+ for cancer cell apoptosis through photothermal conjugated nanoparticles. ACS Appl Bio Mater, 5(6): 2834–2842. https://doi.org/10.1021/acsabm.2c00236
Gu J, Zhang Q, Geng M, et al., 2021, Construction of nanofibrous scaffolds with interconnected perfusable microchannel networks for engineering of vascularized bone tissue. Bioact Mater, 6(10): 3254–3268. https://doi.org/10.1016/j.bioactmat.2021.02.033
Zhang C, Li T, Yin S, et al., 2022, Monocytes deposit migrasomes to promote embryonic angiogenesis. Nat Cell Biol, 24(12): 1726. https://doi.org/10.1038/s41556-022-01026-3
Wu J, Lu Z, Jiang D, et al., 2021, Iterative tomography with digital adaptive optics permits hour-long intravital observation of 3D subcellular dynamics at millisecond scale. Cell, 184(12): 3318. https://doi.org/10.1016/j.cell.2021.04.029
Ma W, Ma H, Qiu P, et al., 2021, Sprayable beta-FeSi2 composite hydrogel for portable skin tumor treatment and wound healing. Biomaterials, 279: 121225. https://doi.org/10.1016/j.biomaterials.2021.121225
Ma Y-X, Jiao K, Wan Q-Q, et al., 2022, Silicified collagen scaffold induces semaphorin 3A secretion by sensory nerves to improve in-situ bone regeneration. Bioact Mater, 9: 475–490. https://doi.org/10.1016/j.bioactmat.2021.07.016