Multifunctional high-simulation 3D-printed hydrogel model manufacturing engineering for surgical training
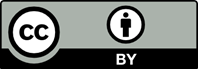
Advanced bionic organ models with vivid biological structures and wetness and softness are essential for medical-surgical training. Still, there are many challenges in the preparation process, such as matching mechanical properties, good feedback on surgical instruments, reproducibility of specific surgical scenarios, and distinguishability between structural levels. In this paper, we achieved tissuemimicking dual-network (DN) hydrogels with customizable stiffness by adjusting the composition of the hydrogel matrix and the immersion time of the ionic solution to match different biological soft tissues precisely. Combined with advanced threedimensional (3D) printing fabrication techniques, various performance-tunable bionic hydrogel organ models with structural complexity and fidelity, including kidney, liver, pancreas, and vascular tissues, were perfectly fabricated. The simulation and applicability of the model were also simulated for the forced change of the suture needle in the puncture and suture of a single tissue and between different tissues, the cutting of substantive organs by ultrasonic scalpel, the coagulation and hemostasis of blood vessels, the visualization of the internal structure under ultrasound, and the microwave ablation of liver tumors. By constructing advanced biomimetic organ models based on hydrogel with specific and tunable properties, the development of surgical training, medical device testing, and medical education reform will be significantly promoted.
Valdivieso RF, Zorn KC, 2014, Urological laparoscopic training—practice makes perfect. Nat Rev Urol, 11(3): 138–139. https://doi.org/10.1038/nrurol.2014.6
Panda N, Morse CR, 2020, Commentary: Practice makes perfect in cervical esophagogastric anastomosis. J Thorac Cardiovasc Surg, 160(6): 1611–1612. https://doi.org/10.1016/j.jtcvs.2020.04.018
Goldhamer MEJ, Pusic MV, Co JPT, et al., 2020, Can covid catalyze an educational transformation? Competency-based advancement in a crisis. N Engl J Med, 383(11): 1003–1005. https://doi.org/10.1056/NEJMp2018570
Reznick RK, MacRae H, 2006, Medical education - teaching surgical skills - changes in the wind. N Engl J Med, 355(25): 2664–2669. https://doi.org/10.1056/NEJMra054785
The Lancet, 2016, The best science for achieving healthy china 2030. Lancet, 388(10054): 1851. https://doi.org/10.1016/S0140-6736(16)31842-6
Rudarakanchana N, Van Herzeele I, Desender L, et al., 2015, Virtual reality simulation for the optimization of endovascular procedures: Current perspectives. Vasc Health Risk Manag, 11: 195–202. https://doi.org/10.2147/VHRM.S46194
Meola A, Cutolo F, Carbone M, et al., 2017, Augmented reality in neurosurgery: A systematic review. Neurosurg Rev, 40(4): 537–548. https://doi.org/10.1007/s10143-016-0732-9
Chevallier C, Willaert W, Kawa E, et al., 2014, Postmortem circulation: a new model for testing endovascular devices and training clinicians in their use. Clin Anat, 27(4): 556–562. https://doi.org/10.1002/ca.22357
Sandmann J, Mueschenich FS, Riabikin A, et al., 2019, Can silicone models replace animal models in hands-on training for endovascular stroke therapy? Interv Neuroradiol, 25(4): 397–402. https://doi.org/10.1177/1591019919833843
Nagassa RG, McMenamin PG, Adams JW, et al., 2019, Advanced 3D printed model of middle cerebral artery aneurysms for neurosurgery simulation. 3D Print Med, 5(1): 11. https://doi.org/10.1186/s41205-019-0048-9
Giannopoulos AA, Mitsouras D, Yoo SJ, et al., 2016, Applications of 3D printing in cardiovascular diseases. Nat Rev Cardiol, 13(12): 701–718.https://doi.org/10.1038/nrcardio.2016.170
Kaneko N, Mashiko T, Ohnishi T, et al., 2016, Manufacture of patient-specific vascular replicas for endovascular simulation using fast, low-cost method. Sci Rep, 6(1): 39168. https://doi.org/10.1038/srep39168
Zhang Y, Xia J, Zhang J, et al., 2022, Validity of a soft and flexible 3D-printed nissen fundoplication model in surgical training. Int J Bioprint, 8(2): 546. https://doi.org/10.18063/ijb.v8i2.546
de Jong TL, Pluymen LH, van Gerwen DJ, et al., 2017, PVA matches human liver in needle-tissue interaction. J Mech Behav Biomed Mater, 69: 223–228. https://doi.org/10.1016/j.jmbbm.2017.01.014
Earle M, Portu GD, DeVos E, 2016, Agar ultrasound phantoms for low-cost training without refrigeration. Afr J Emerg Med Rev Afr Med Urgence, 6(1): 18–23. https://doi.org/10.1016/j.afjem.2015.09.003
Ratinam R, Quayle M, Crock J, et al., 2019, Challenges in creating dissectible anatomical 3D prints for surgical teaching. J Anat, 234(4): 419–437. https://doi.org/10.1111/joa.12934
Liu X, Liu J, Lin S, et al., 2020, Hydrogel machines. Mater Today, 36: 102–124. https://doi.org/10.1016/j.mattod.2019.12.026
Ligon SC, Liska R, Stampfl J, et al., 2017, Polymers for 3D printing and customized additive manufacturing. Chem Rev, 117(15): 10212–10290. https://doi.org/10.1021/acs.chemrev.7b00074
Jin Z, Li Y, Yu K, et al., 2021, 3D printing of physical organ models: recent developments and challenges. Adv Sci, 8(17): 2101394. https://doi.org/10.1002/advs.202101394
Ng WL, Chua CK, Shen YF, 2019, Print me an organ! Why we are not there yet. Prog Polym Sci, 97: 101145. https://doi.org/10.1016/j.progpolymsci.2019.101145
Jiang P, Ji Z, Liu D, et al., 2022, Growing hydrogel organ mannequins with interconnected cavity structures. Adv Funct Mater, 32(13): 2108845. https://doi.org/10.1002/adfm.202108845
Wang M, Li W, Hao J, et al., 2022, Molecularly cleavable bioinks facilitate high-performance digital light processing-based bioprinting of functional volumetric soft tissues. Nat Commun, 13(1): 3317. https://doi.org/10.1038/s41467-022-31002-2
Yang H, Ji M, Yang M, et al., 2021, Fabricating hydrogels to mimic biological tissues of complex shapes and high fatigue resistance. Matter, 4(6): 1935–1946. https://doi.org/10.1016/j.matt.2021.03.011
Zhao Z, Vizetto DC, Moay ZK, et al., 2020, Composite hydrogels in three-dimensional in vitro models. Front Bioeng Biotechnol, 8: 611. https://doi.org/10.3389/fbioe.2020.00611
Zhong M, Liu YT, Xie XM, 2015, Self-healable, super tough graphene oxide-poly(acrylic acid) nanocomposite hydrogels facilitated by dual cross-linking effects through dynamic ionic interactions. J Mater Chem B, 3(19): 4001–4008. https://doi.org/10.1039/c5tb00075k
Guimarães CF, Gasperini L, Marques AP, et al., 2020, The stiffness of living tissues and its implications for tissue engineering. Nat Rev Mater, 5(5): 351–370. https://doi.org/10.1038/s41578-019-0169-1
Golberg A, Bruinsma BG, Uygun BE, et al., 2015, Tissue heterogeneity in structure and conductivity contribute to cell survival during irreversible electroporation ablation by “electric field sinks’’. Sci Rep, 5(1): 8485. https://doi.org/10.1038/srep08485
Joines W, Zhang Y, Li C, et al., 1994, The measured electrical-properties of normal and malignant human tissues from 50 to 900 MHz. Med Phys, 21(4): 547–550. https://doi.org/10.1118/1.597312
Yue K, Cheng L, Yang L, et al., 2017, Thermal conductivity measurement of anisotropic biological tissue in vitro. Int J Thermophys, 38(6): 92. https://doi.org/10.1007/s10765-017-2214-x
Liu D, Jiang P, Wang Y, et al., 2023, Engineering tridimensional hydrogel tissue and organ phantoms with tunable springiness. Adv Funct Mater, 33(17): 2214885. https://doi.org/10.1002/adfm.202214885
van Gerwen DJ, Dankelman J, van den Dobbelsteen JJ, 2012, Needle-tissue interaction forces - a survey of experimental data. Med Eng Phys, 34(6): 665–680. https://doi.org/10.1016/j.medengphy.2012.04.007
Bao X, Li W, Lu M, et al., 2016, Experiment study on puncture force between MIS suture needle and soft tissue. Biosurface Biotribology, 2(2): 49–58. https://doi.org/10.1016/j.bsbt.2016.05.001
Grochola LF, Vonlanthen R, 2016, Surgical energy devices or devices for hemostasis. Atlas Up Gastrointest Hepato- Pancreato-Biliary Surg, 37–44. https://doi.org/10.1007/978-3-662-46546-2_6
Chan YC, Li WF, Lin TL, et al., 2013, Lin’s clamp revisited: A safe model for training in liver resection. Formos J Surg, 46(2): 42–47. https://doi.org/10.1016/j.fjs.2013.02.001
Liang P, Yu J, Lu MD, et al., 2013, Practice guidelines for ultrasound-guided percutaneous microwave ablation for hepatic malignancy. World J Gastroenterol, 19(33): 5430–5438. https://doi.org/10.3748/wjg.v19.i33.5430