3D printing of mechanically tough and self-healing hydrogels with carbon nanotube fillers
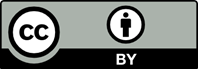
Hydrogels have the potential to play a crucial role in bioelectronics, as they share many properties with human tissues. However, to effectively bridge the gap between electronics and biological systems, hydrogels must possess multiple functionalities, including toughness, stretchability, self-healing ability, three-dimensional (3D) printability, and electrical conductivity. Fabricating such tough and self-healing materials has been reported, but it still remains a challenge to fulfill all of those features, and in particular, 3D printing of hydrogel is in the early stage of the research. In this paper, we present a 3D printable, tough, and self-healing multi-functional hydrogel in one platform made from a blend of poly(vinyl alcohol) (PVA), tannic acid (TA), and poly(acrylic acid) (PAA) hydrogel ink (PVA/TA/PAA hydrogel ink). Based on a reversible hydrogen-bond (H-bond)-based double network, the developed 3D printable hydrogel ink showed excellent printability via shear-thinning behavior, allowing high printing resolution (~100 μm) and successful fabrication of 3D-printed structure by layer-by-layer printing. Moreover, the PVA/TA/PAA hydrogel ink exhibited high toughness (tensile loading of up to ~45.6 kPa), stretchability (elongation of approximately 650%), tissue-like Young’s modulus (~15 kPa), and self-healing ability within 5 min. Furthermore, carbon nanotube (CNT) fillers were successfully added to enhance the electrical conductivity of the hydrogel. We confirmed the practicality of the hydrogel inks for bioelectronics by demonstrating biocompatibility, tissue adhesiveness, and strain sensing ability through PVA/TA/PAA/CNT hydrogel ink.
Stanford V, 2004, Biosignals offer potential for direct interfaces and health monitoring. IEEE Pervasive Comput, 3(1): 99–103. https://doi.org/10.1109/MPRV.2004.1269140
Swapna M, Viswanadhula UM, Aluvalu R, et al., 2022, Bio-signals in medical applications and challenges using artificial intelligence. J Sens Actuator Netw, 11(1): 17. https://doi.org/10.3390/jsan11010017
Dash A, Cudworth II G, 1998, Therapeutic applications of implantable drug delivery systems. J Pharmacol Toxicol Methods, 40: 1–12. https://doi.org/10.1002/adma.201902783
Liu Y, Yang T, Zhang Y, et al., 2019, Ultrastretchable and wireless bioelectronics based on all-hydrogel microfluidics. Adv Mat, 31(39): 1902783. https://doi.org/10.1002/adma.201902783
Webb RC, Bonifas AP, Behnaz A, et al., 2013, Ultrathin conformal devices for precise and continuous thermal characterization of human skin. Nat Mater, 12(10): 938–44. http://doi.org/10.1038/nmat3755
Gao G, Yang F, Zhou F, et al., 2020, Bioinspired self‐healing human–machine interactive touch pad with pressure‐sensitive adhesiveness on targeted substrates. Adv Mat, 32(50): 2004290. https://doi.org/10.1002/adma.202004290
Yuk H, Lu B, Zhao X, 2019, Hydrogel bioelectronics. Chem Soc Rev, 48(6): 1642–1667. http://doi.org/10.1039/C8CS00595H
Appel EA, del Barrio J, Loh XJ, et al., 2012, Supramolecular polymeric hydrogels. Chem Soc Rev, 41(18): 6195–6214. http://doi.org/10.1039/C2CS35264H
Kifaro EG, Kim MJ, Jung S, et al., 2022, Direct reverse transcription real-time PCR of viral RNA from saliva samples using hydrogel microparticles. Biochip J, 16(4): 409–421. http://doi.org/10.1007/s13206-022-00065-0
Zhang YS, Khademhosseini A, 2017, Advances in engineering hydrogels. Science, 356(6337): eaaf3627. http://doi.org/10.1126/science.aaf3627
Liu Y, He W, Zhang Z, et al., 2018, Recent developments in tough hydrogels for biomedical applications. Gels, 4(2): 46. https://doi.org/10.3390/gels4020046
Tee BCK, Wang C, Allen R, et al., 2012, An electrically and mechanically self-healing composite with pressure- and flexion-sensitive properties for electronic skin applications. Nat Nanotechnol, 7(12): 825–832. http://doi.org/10.1038/nnano.2012.192
Chen Z, Luo J, Hu Y, et al., 2022, Fabrication of lignin reinforced hybrid hydrogels with antimicrobial and self-adhesion for strain sensors. Int J Biol Macromol, 222 (Part A): 487–496. https://doi.org/10.1016/j.ijbiomac.2022.09.197
Guo Y, Bae J, Fang Z, et al., 2020, Hydrogels and hydrogel-derived materials for energy and water sustainability. Chem Rev, 120(15): 7642–7707. http://doi.org/10.1021/acs.chemrev.0c00345
Lu B, Lin F, Jiang X, et al., 2017, One-pot assembly of microfibrillated cellulose reinforced PVA–borax hydrogels with self-healing and pH-responsive properties. ACS Sustain Chem Eng, 5(1): 948–956. http://doi.org/10.1021/acssuschemeng.6b02279
Billiet T, Vandenhaute M, Schelfhout J, et al., 2012, A review of trends and limitations in hydrogel-rapid prototyping for tissue engineering. Biomaterials, 33(26): 6020–6041. https://doi.org/10.1016/j.biomaterials.2012.04.050
Namgung H, Kaba AM, Oh H, et al., 2022, Quantitative determination of 3D-printing and surface-treatment conditions for direct-printed microfluidic devices. BioChip J, 16(1): 82–98. http://doi.org/10.1007/s13206-022-00048-1
Tan HW, Choong YYC, Kuo CN, et al., 2022, 3D printed electronics: Processes, materials and future trends. Prog Mater Sci, 127: 100945. https://doi.org/10.1016/j.pmatsci.2022.100945
Chen J, Peng Q, Thundat T, et al., 2019, Stretchable, injectable, and self-healing conductive hydrogel enabled by multiple hydrogen bonding toward wearable electronics. Chem Mater, 31(12): 4553–4563. http://doi.org/10.1021/acs.chemmater.9b01239
Jin S, Kim Y, Son D, et al., 2022, Tissue adhesive, conductive, and injectable cellulose hydrogel ink for on-skin direct writing of electronics. Gels, 8(6): 336.
Wei J, Wang J, Su S, et al., 2015, 3D printing of an extremely tough hydrogel. RSC Adv, 5(99): 81324–81329. http://doi.org/10.1039/C5RA16362E
Song PA, Xu Z, Guo Q, 2013, Bioinspired strategy to reinforce PVA with improved toughness and thermal properties via hydrogen-bond self-assembly. ACS Macro Lett, 2(12): 1100–1104. http://doi.org/10.1021/mz4005265
George J, Sabapathi SN, Siddaramaiah, 2015, Water soluble polymer-based nanocomposites containing cellulose nanocrystals, in Eco-friendly Polymer Nanocomposites: Processing and Properties, Thakur VK, Thakur MK (Eds), Springer India, New Delhi, 259–293.
Meng Y, Cao J, Chen Y, et al., 2020, 3D printing of a poly(vinyl alcohol)-based nano-composite hydrogel as an artificial cartilage replacement and the improvement mechanism of printing accuracy. J Mater Chem B, 8(4): 677–690. http://doi.org/10.1039/C9TB02278C
Chen W, Li N, Ma Y, et al., 2019, Superstrong and tough hydrogel through physical cross-linking and molecular alignment. Biomacromolecules, 20(12): 4476–4484. http://doi.org/10.1021/acs.biomac.9b01223
Fan H, Wang J, Jin Z, 2018, Tough, swelling-resistant, self-healing, and adhesive dual-cross-linked hydrogels based on polymer–tannic acid multiple hydrogen bonds. Macromolecules, 51(5): 1696–1705. http://doi.org/10.1021/acs.macromol.7b02653
Jafari H, Ghaffari-Bohlouli P, Niknezhad SV, et al., 2022, Tannic acid: A versatile polyphenol for design of biomedical hydrogels. J Mater Chem B, 10(31): 5873–5912. http://doi.org/10.1039/D2TB01056A
Shi S, Peng X, Liu T, et al., 2017, Facile preparation of hydrogen-bonded supramolecular polyvinyl alcohol-glycerol gels with excellent thermoplasticity and mechanical properties. Polymer, 111: 168–176.
Kang J, Son D, Wang G-JN, et al., 2018, Tough and water-insensitive self-healing elastomer for robust electronic skin. Adv Mater, 30(13): 1706846. https://doi.org/10.1002/adma.201706846
Liu T, Jiao C, Peng X, et al., 2018, Super-strong and tough poly(vinyl alcohol)/poly(acrylic acid) hydrogels reinforced by hydrogen bonding. J Mater Chem B, 6(48): 8105–8114. http://doi.org/10.1039/C8TB02556H
Qin H, Zhang T, Li N, et al., 2019, Anisotropic and self-healing hydrogels with multi-responsive actuating capability. Nat Commun, 10(1): 2202. http://doi.org/10.1038/s41467-019-10243-8
Canadell J, Goossens H, Klumperman B, 2011, Self-healing materials based on disulfide links. Macromolecules, 44(8): 2536–2541. http://doi.org/10.1021/ma2001492
Sun TL, Luo F, Hong W, et al., 2017, Bulk energy dissipation mechanism for the fracture of tough and self-healing hydrogels. Macromolecules, 50(7): 2923–2931. http://doi.org/10.1021/acs.macromol.7b00162
Zhou J, Vijayavenkataraman S, 2021, 3D-printable conductive materials for tissue engineering and biomedical applications. Bioprinting, 24: e00166. https://doi.org/10.1016/j.bprint.2021.e00166
Smith PT, Basu A, Saha A, et al., 2018, Chemical modification and printability of shear-thinning hydrogel inks for direct-write 3D printing. Polymer, 152: 42–50. https://doi.org/10.1016/j.polymer.2018.01.070
Zhou J, Yan H, Wang C, et al., 2022, 3D printing highly stretchable conductors for flexible electronics with low signal hysteresis. Virtual Phys Prototyp, 17(1): 19–32. http://doi.org/10.1080/17452759.2021.1980283
Karolina Pierchala M, Kadumudi FB, Mehrali M, et al., 2021, Soft electronic materials with combinatorial properties generated via mussel-inspired chemistry and halloysite nanotube reinforcement. ACS Nano, 15(6): 9531–9549. http://doi.org/10.1021/acsnano.0c09204
Liu X, Liu J, Lin S, et al., 2020, Hydrogel machines. Mater Today, 36: 102–124. https://doi.org/10.1016/j.mattod.2019.12.026
Lu Y, Biswas MC, Guo Z, et al., 2019, Recent developments in bio-monitoring via advanced polymer nanocomposite-based wearable strain sensors. Biosens Bioelectron, 123: 167–177. https://doi.org/10.1016/j.bios.2018.08.037
Dannert C, Stokke BT, Dias RS, 2019, Nanoparticle-hydrogel composites: From molecular interactions to macroscopic behavior. Polymers, 11(2): 275. http://doi.org/ 10.3390/polym11020275
Sawicki K, Czajka M, Matysiak-Kucharek M, et al., 2019, Toxicity of metallic nanoparticles in the central nervous system. Nanotechnol Rev, 8(1): 175–200. http://doi.org/doi:10.1515/ntrev-2019-0017
Zheng W, Li Y, Xu L, et al., 2020, Highly stretchable, healable, sensitive double-network conductive hydrogel for wearable sensor. Polymer, 211: 123095. https://doi.org/10.1016/j.polymer.2020.123095
Kim T, Park J, Sohn J, et al., 2016, Bioinspired, highly stretchable, and conductive dry adhesives based on 1D– 2D hybrid carbon nanocomposites for all-in-one ECG electrodes. ACS Nano, 10(4): 4770–4778. http://doi.org/10.1021/acsnano.6b01355
Iversen M, Monisha M, Agarwala S, 2022, Flexible, wearable and fully-printed smart patch for pH and hydration sensing in wounds. Int J Bioprint, 8(1): 447. http://doi.org/10.18063/ijb.v8i1.447
Yuk H, Varela CE, Nabzdyk CS, et al., 2019, Dry double-sided tape for adhesion of wet tissues and devices. Nature, 575(7781): 169–174. http://doi.org/10.1038/s41586-019-1710-5
Zhao W, Huang B, Zhu L, et al., 2022, Printable hydrogels based on starch and natural rubber latex with high toughness and self-healing capability. Int J Biol Macromol, 218: 580–587. https://doi.org/10.1016/j.ijbiomac.2022.07.148
Park J, Kim JY, Heo JH, et al., 2023, Intrinsically nonswellable multifunctional hydrogel with dynamic nanoconfinement networks for robust tissue-adaptable bioelectronics. Adv Sci, 10: 2207237. https://doi.org/10.1002/advs.202207237