Smart implants: 4D-printed shape-morphing scaffolds for medical implantation
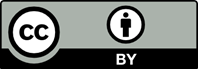
Biomedical implants have recently shown excellent application potential in tissue repair and replacement. Applying three-dimensional (3D) printing to implant scaffold fabrication can help to address individual needs more precisely. Fourdimensional (4D) printing emerges rapidly based on the development of shaperesponsive materials and design methods, which makes the production of dynamic functional implants possible. Smart implants can be pre-designed to respond to endogenous or exogenous stimuli and perform seamless integration with regular/ irregular tissue defects, defect-luminal organs, or curved structures via programmed shape morphing. At the same time, they offer great advantages in minimally invasive surgery due to the small-to-large volume transition. In addition, 4D-printed cellular scaffolds can generate extracellular matrix (ECM)-mimetic structures that interact with the contacting cells, expanding the possible sources of tissue/organ grafts and substitutes. This review summarizes the typical technologies and materials of 4D-printed scaffolds, and the programming designs and applications of these scaffolds are further highlighted. Finally, we propose the prospects and outlook of 4D-printed shape-morphing implants.
Liu G, He Y, Liu P, et al., 2020, Development of bioimplants with 2D, 3D, and 4D additive manufacturing materials. Engineering, 6(11): 1232–1243. https://doi.org/10.1016/j.eng.2020.04.015
Dixon DT, Gomillion CT, 2022, Conductive scaffolds for bone tissue engineering: Current state and future outlook. J Funct Biomater, 13(1): 1. https://doi.org/10.3390/jfb13010001
Cockerill I, See CW, Young ML, et al., 2021, Designing better cardiovascular stent materials: A learning curve. Adv Funct Mater, 31(1): 2005361. https://doi.org/10.1002/adfm.202005361
Teo AJT, Mishra A, Park I, et al., 2016, Polymeric biomaterials for medical implants and devices. ACS Biomater Sci Eng, 2(4): 454–472. https://doi.org/10.1021/acsbiomaterials.5b00429
Azlin MNM, Ilyas RA, Zuhri MYM, et al., 2022, 3D printing and shaping polymers, composites, and nanocomposites: A review. Polymers, 14(1): 180. https://doi.org/10.3390/polym14010180
Sonatkar J, Kandasubramanian B, Ismail SO, 2022, 4D printing: Pragmatic progression in biofabrication. Eur Polym J, 169: 111128. https://doi.org/10.1016/j.eurpolymj.2022.111128
Osouli-Bostanabad K, Masalehdan T, Kapsa RMI, et al., 2022, Traction of 3D and 4D printing in the healthcare industry: From drug delivery and analysis to regenerative medicine. ACS BiomaterSci Eng, 8(7): 2764–2797. https://doi.org/10.1021/acsbiomaterials.2c000942764
Li Y-C, Zhang YS, Akpek A, et al., 2017, 4D bioprinting: The next-generation technology for biofabrication enabled by stimuli-responsive materials. Biofabrication, 9(1): 012001. https://doi.org/10.1088/1758-5090/9/1/012001
Huang J, Xia S, Li Z, et al., 2021, Applications of four-dimensional printing in emerging directions: Review and prospects. J Mater Sci Technol, 91: 105–120. https://doi.org/10.1016/j.jmst.2021.02.040
Gao B, Yang Q, Zhao X, et al., 2016, 4D bioprinting for biomedical applications. Trends Biotechnol, 34(9): 746–756. https://doi.org/10.1016/j.tibtech.2016.03.004
Yang Q, Gao B, Xu F, 2020, Recent advances in 4D bioprinting. Biotechnol J, 15(1): 1900086. https://doi.org/10.1002/biot.201900086
Kirillova A, Maxson R, Stoychev G, et al., 2017, 4D biofabrication using shape-morphing hydrogels. Adv Mater, 29(46): 1703443. https://doi.org/10.1002/adma.201703443
Yang GH, Yeo M, Koo YW, et al., 2019, 4D bioprinting: Technological advances in biofabrication. Macromol Biosci, 19(5): 1800441. https://doi.org/10.1002/mabi.201800441
Constante G, Apsite I, Alkhamis H, et al., 2021, 4D biofabrication using a combination of 3D printing and melt-electrowriting of shape-morphing polymers. ACS Appl Mater Interfaces, 13(11): 12767–12776. https://doi.org/10.1021/acsami.0c18608
Li J, Wu C, Chu PK, et al., 2020, 3D printing of hydrogels: Rational design strategies and emerging biomedical applications. Mater Sci Eng, 140: 100543. https://doi.org/10.1016/j.mser.2020.100543
Derakhshanfar S, Mbeleck R, Xu K, et al., 2018, 3D bioprinting for biomedical devices and tissue engineering: A review of recent trends and advances. Bioact Mater, 3(2): 144–156. https://doi.org/10.1016/j.bioactmat.2017.11.008
Lee H, Ahn S, Bonassar LJ, et al., 2013, Cell-laden poly(epsilon-caprolactone)/alginate hybrid scaffolds fabricated by an aerosol cross-linking process for obtaining homogeneous cell distribution: Fabrication, seeding efficiency, and cell proliferation and distribution. Tissue Eng Part C Methods, 19(10), 784–793. https://doi.org/10.1089/ten.tec.2012.0651
Kundu J, Shim J-H, Jang J, et al., 2015, An additive manufacturing-based PCL-alginate-chondrocyte bioprinted scaffold for cartilage tissue engineering. J Tissue Eng Regen Med, 9(11): 1286–1297. https://doi.org/10.1002/term.1682
Kuang X, Roach DJ, Wu J, et al., 2019, Advances in 4D printing: Materials and applications. Adv Funct Mater, 29(2): 1805290. https://doi.org/10.1002/adfm.201805290
Bittner SM, Guo JL, Melchiorri A, et al., 2018, Three-dimensional printing of multilayered tissue engineering scaffolds. Mater Today, 21(8): 861–874. https://doi.org/10.1016/j.mattod.2018.02.006
Alshahrani HA, 2021, Review of 4D printing materials and reinforced composites: Behaviors, applications and challenges. J Sci, 6(2): 167–185. https://doi.org/10.1016/j.jsamd.2021.03.006
Joshi S, Rawat K, Karunakaran C, et al., 2020, 4D printing of materials for the future: Opportunities and challenges. Appl Mater Today, 18: 100490. https://doi.org/10.1016/j.apmt.2019.100490
Wang Y, Cui H, Esworthy T, et al., 2022, Emerging 4D printing strategies for next-generation tissue regeneration and medical devices. Adv Mater, 34(20): 2109198. https://doi.org/10.1002/adma.202109198
Xia Y, He Y, Zhang F, et al., 2021, 2021, A review of shape memory polymers and composites: Mechanisms, materials, and applications. Adv Mater, 33(6): 2000713. https://doi.org/10.1002/adma.202000713
Wang L, Zhang F, Liu Y, et al., 2022, Shape memory polymer fibers: Materials, structures, and applications. Adv Fiber Mater, 4(1): 5–23. https://doi.org/10.1007/s42765-021-00073-z
Zhang J, Yin Z, Ren L, et al., 2022, Advances in 4D printed shape memory polymers: From 3D printing, smart excitation, and response to applications. Adv Mater Technolog, 7(9): 2101568. https://doi.org/10.1002/admt.202101568
Khalid MY, Arif ZU, Ahmed W, 2022, 4D printing: Technological and manufacturing renaissance. Macromol Mater Eng, 307(8): 2200003. https://doi.org/10.1002/mame.202200003
Alief NA, Supriadi S, Whulanza Y, 2019, Modelling the shape memory properties of 4D printed polylactic acid (PLA) for application of disk spacer in minimally invasive spinal fusion. Proceedings of the 3rd International Symposium of Biomedical Engineering (ISBE) on Recent Progress in Biomaterials, Drugs Development, and Medical Devices: 2019, 020005. https://doi.org/10.1063/1.5096673
Kravtcova A, Toncheva A, Rantataro S, et al., 2021, Shape memory polymer-based insertable electrode array towards minimally invasive subdural implantation. IEEE Sens J, 21(15): 17282–17289. https://doi.org/10.1109/jsen.2021.3078358
Lin C, Zhang L, Liu Y, et al., 2020, 4D printing of personalized shape memory polymer vascular stents with negative Poisson’s ratio structure: A preliminary study. Sci China Technol Sci, 63(4): 578–588. https://doi.org/10.1007/s11431-019-1468-2
Miao S, Nowicki M, Cui H, et al, 2019, 4D anisotropic skeletal muscle tissue constructs fabricated by staircase effect strategy. Biofabrication, 11(3): 035030. https://doi.org/10.1088/1758-5090/ab1d07
Miao S, Cui H, Nowicki M, et al., 2018, Photolithographic-stereolithographic-tandem fabrication of 4D smart scaffolds for improved stem cell cardiomyogenic differentiation. Biofabrication, 10(3): 035007. https://doi.org/10.1088/1758-5090/aabe0b
Miao S, Cui H, Nowicki M, et al., 2018, Stereolithographic 4D bioprinting of multiresponsive architectures for neural engineering. Adv Biosys, 2(9): 1800101. https://doi.org/10.1002/adbi.201800101
Miao S, Zhu W, Castro NJ, et al., 2016, 4D printing smart biomedical scaffolds with novel soybean oil epoxidized acrylate. Sci Rep, 6: 27226. https://doi.org/10.1038/srep27226
Zhao W, Liu L, Zhang F, et al., 2019, Shape memory polymers and their composites in biomedical applications. Mater Sci Eng C, 97: 864–883. https://doi.org/10.1016/j.msec.2018.12.054
Pfau MR, McKinzey KG, Roth AA, et al., 2021, Shape memory polymer (SMP) scaffolds with improved self-fitting properties. J Mater Chem B, 9(18): 3826–3837. https://doi.org/10.1039/d0tb02987d
Zeng X, Wang L, Chen X, et al., 2022, 3D biocompatible bone engineering foams with tunable mechanical properties and porous structures. J Appl Polym Sci, 139(21): e52228. https://doi.org/10.1002/app.52228
Huang X, Panahi-Sarmad M, Dong K, et al., 4D printed TPU/PLA/CNT wave structural composite with intelligent thermal-induced shape memory effect and synergistically enhanced mechanical properties. Compos Part A Appl Sci Manuf, 158: 106946. https://doi.org/10.1016/j.compositesa.2022.106946
Wan X, He Y, Liu Y, et al., 2022, 4D printing of multiple shape memory polymer and nanocomposites with biocompatible, programmable and selectively actuated properties. Addit Manuf, 53: 102689. https://doi.org/10.1016/j.addma.2022.102689
El-Husseiny HM, Mady EA, Hamabe L, et al., 2022, Smart/ stimuli-responsive hydrogels: Cutting-edge platforms for tissue engineering and other biomedical applications. Mater Today Bio, 13: 100186. https://doi.org/10.1016/j.mtbio.2021.100186
Yesilyurt V, Webber MJ, Appel EA, et al., 2016, Injectable self-healing glucose-responsive hydrogels with pH-regulated mechanical properties. Adv Mater, 28(1): 86–91. https://doi.org/10.1002/adma.201502902
Shafranek RT, Millik SC, Smith PT, et al., 2019, Stimuli-responsive materials in additive manufacturing. Prog Polym Sci, 93: 36–67. https://doi.org/10.1016/j.progpolymsci.2019.03.002
Willner I, 2017, Stimuli-controlled hydrogels and their applications. Acc Chem Res, 50(4): 657–658. https://doi.org/10.1021/acs.accounts.7b00142
Nagase K, 2021, Thermoresponsive interfaces obtained using poly (N-isopropylacrylamide)-based copolymer for bioseparation and tissue engineering applications. Adv Colloid Interface Sci, 295: 102487. https://doi.org/10.1016/j.cis.2021.102487
Tang L, Wang L, Yang X, et al., 2021, Poly(N-isopropylacrylamide)-based smart hydrogels: Design, properties and applications. Prog Mater Sci, 115: 100702. https://doi.org/10.1016/j.pmatsci.2020.100702
Ma Y, Hua M, Wu S, et al., 2020, Bioinspired high-power-density strong contractile hydrogel by programmable elastic recoil. Sci Adv, 6(47): eabd2520. https://doi.org/10.1126/sciadv.abd2520
Khoo ZX, Liu Y, An J, et al., 2018, A review of selective laser melted NiTi shape memory alloy. Materials, 11(4): 519. https://doi.org/10.3390/ma11040519
Sahafnejad-Mohammadi I, Karamimoghadam M, Zolfagharian A, et al., 2022, 4D printing technology in medical engineering: A narrative review. J Braz Soc Mech Sci Eng, 44(6): 233. https://doi.org/10.1007/s40430-022-03514-x
Yuritsa Paez-Pidiache I, Luviano-Juarez A, Lozada-Castillo N, et al., 2021, Design, characterization and construction of an actuator based on shape memory alloys. MRS Adv, 6(39– 40): 907–912. https://doi.org/10.1557/s43580-021-00158-2
Sarraf M, Rezvani Ghomi E, Alipour S, et al., 2022, A state-of-the-art review of the fabrication and characteristics of titanium and its alloys for biomedical applications. Bio-Des Manuf, 5(2): 371–395. https://doi.org/10.1007/s42242-021-00170-3
Guan Z, Wang L, Bae J, 2022, Advances in 4D printing of liquid crystalline elastomers: Materials, techniques, and applications. Mater Horiz, 9(7): 1825–1849. https://doi.org/10.1039/d2mh00232a
Wu J, Yao S, Zhang H, et al., 2021, Liquid crystal elastomer metamaterials with giant biaxial thermal shrinkage for enhancing skin regeneration. Adv Mater, 33(45): 2106175. https://doi.org/10.1002/adma.202106175
Javadzadeh M, del Barrio J, Sanchez-Somolinos C, 2023, Melt electrowriting of liquid crystal elastomer scaffolds with programmed mechanical response. Adv Mater, 35(14): 2209244. https://doi.org/10.1002/adma.202209244
Lee J-H, Bae J, Hwang JH, et al., 2022, Robust and reprocessable artificial muscles based on liquid crystal elastomers with dynamic thiourea bonds. Adv Funct Mater, 32(13): 2110360. https://doi.org/10.1002/adfm.202110360
Hou W, Wang J, Lv J-a, 2023, Bioinspired liquid crystalline spinning enables scalable fabrication of high-performing fibrous artificial muscles. Adv Mater, 35(16): 2211800. https://doi.org/10.1002/adma.202211800
Ula SW, Traugutt NA, Volpe RH, et al., 2018, Liquid crystal elastomers: An introduction and review of emerging technologies. Liq Cryst Rev, 6(1): 78–107. https://doi.org/10.1080/21680396.2018.1530155
Lai A, Du Z, Gan CL, et al., 2013, Shape memory and superelastic ceramics at small scales. Science, 341(6153): 1505–1508. https://doi.org/10.1126/science.1239745
Wang F, Liu C, Yang H, et al., 2023, 4D printing of ceramic structures. Addit Manuf, 63, 103411. https://doi.org/10.1016/j.addma.2023.103411
Chen S, Li J, Shi H, et al., 2023, Lightweight and geometrically complex ceramics derived from 4D printed shape memory precursor with reconfigurability and programmability for sensing and actuation applications. Chem Eng J, 455: 140655. https://doi.org/10.1016/j.cej.2022.140655
Wang X, Zhang Y, Shen P, et al., 2022, Preparation of 4D printed peripheral vascular stent and its degradation behavior under fluid shear stress after deployment. Biomater Sci, 10(9): 2302–2314. https://doi.org/10.1039/d2bm00088a
Lee S, Bang D, Park J-O, et al., 2022, Programmed shape-morphing material using single-layer 4D printing system. Micromachines, 13(2): 243. https://doi.org/10.3390/mi13020243
Kuhnt T, Camarero-Espinosa S, Ghahfarokhi MT, et al., 2022, 4D printed shape morphing biocompatible materials based on anisotropic ferromagnetic nanoparticles. Adv Funct Mate, 32(50): 2202539. https://doi.org/10.1002/adfm.202202539
Siminska-Stanny J, Niziol M, Szymczyk-Ziolkowska P, et al., 2022, 4D printing of patterned multimaterial magnetic hydrogel actuators. Addit Manuf, 49: 102506. https://doi.org/10.1016/j.addma.2021.102506
Wang Z, Wu Y, Wu D, et al., 2022, Soft magnetic composites for highly deformable actuators by four-dimensional electrohydrodynamic printing. Compos Part B Eng, 231: 109596. https://doi.org/10.1016/j.compositesb.2021.109596
Dong X, Zhang F, Wang L, et al., 2022, 4D printing of electroactive shape-changing composite structures and their programmable behaviors. Compos Part A Appl Sci Manuf, 157: 106925. https://doi.org/10.1016/j.compositesa.2022.106925
Pineda-Castillo SA, Luo J, Lee H, et al., 2021, Effects of carbon nanotube infiltration on a shape memory polymer-based device for brain aneurysm therapeutics: Design and characterization of a joule-heating triggering mechanism. Adv Eng Mater, 23(6): 2100322. https://doi.org/10.1002/adem.202100322
Wang F, Wang W, Zhang C, et al., 2021, Scalable manufactured bio-based polymer nanocomposite with instantaneous near-infrared light-actuated targeted shape memory and remote-controlled accurate self-healing. Compos Part B Eng, 219: 108927. https://doi.org/10.1016/j.compositesb.2021.108927
Chen Y, Zhao X, Luo C, et al., 2020, A facile fabrication of shape memory polymer nanocomposites with fast light-response and self-healing performance. Compos Part A Appl Sci Manuf, 135: 105931. https://doi.org/10.1016/j.compositesa.2020.105931
Chen Y, Zhao X, Li Y, et al., 2021, Light- and magnetic-responsive synergy controlled reconfiguration of polymer nanocomposites with shape memory assisted self-healing performance for soft robotics. J Mater Chem C, 9(16): 5515– 5527. https://doi.org/10.1039/d1tc00468a
Deng Y, Zhang F, Jiang M, et al., 2022, Programmable 4D printing of photoactive shape memory composite structures. ACS Appl Mater Interfaces, 14(37): 42568–42577. https://doi.org/10.1021/acsami.2c13982
Li M, Fu S, Lucia LA, et al., 2020, Ultra-efficient photo-triggerable healing and shape-memory nanocomposite materials doped with copper sulfide nanoparticles. Compos Sci Technol, 199: 108371. https://doi.org/10.1016/j.compscitech.2020.108371
Koh TY, Sutradhar A, 2022, Untethered selectively actuated microwave 4D printing through ferromagnetic PLA. Addit Manuf, 56: 102866. https://doi.org/10.1016/j.addma.2022.102866
Jamal M, Kadam SS, Xiao R, et al., 2013, Bio-origami hydrogel scaffolds composed of photocrosslinked PEG bilayers. Adv Healthc Mater, 2(8): 1142–1150. https://doi.org/10.1002/adhm.201200458
Kwag HR, Serbo JV, Korangath P, et al., 2016, A self-folding hydrogel in vitro model for ductal carcinoma. Tissue Eng Part C Methods, 22(4): 398–407. https://doi.org/10.1089/ten.tec.2015.0442
Mulakkal MC, Trask RS, Ting VP, et al., 2018, Responsive cellulose-hydrogel composite ink for 4D printing. Mater Des, 160: 108–118. https://doi.org/10.1016/j.matdes.2018.09.009
Kim SH, Seo YB, Yeon YK, et al., 2020, 4D-bioprinted silk hydrogels for tissue engineering. Biomaterials, 260: 120281. https://doi.org/10.1016/j.biomaterials.2020.120281
Wu C, Chen J, Su C, 2022, 4D-printed pH sensing claw. Anal Chim Acta, 1204: 339733. https://doi.org/10.1016/j.aca.2022.339733
Cao P, Yang J, Gong J, et al., 2022, 4D printing of bilayer tubular structure with dual-stimuli responsive based on self-rolling behavior. J Appl Polym Sci, 140(1): e53241. https://doi.org/10.1002/app.53241
Rivera-Tarazona LK, Shukla T, Singh KA, et al., 2022, 4D printing of engineered living materials. Adv Funct Mater, 32(4): 2106843. https://doi.org/10.1002/adfm.202106843
Grassi G, Sparrman B, Paoletti I, et al., 2021, 4D soft material systems. Proceedings of the 3rd International Conference on Computational Design and Robotic Fabrication (CDRF) (DigitalFUTURES): 2021 2021; Tongji Univ, Coll Architecture & Urban Planning, Shanghai, PEOPLES R CHINA, 201–210. https://doi.org/10.1007/978-981-16-5983-6_19
Zhou LY, Ye JH, Fu JZ, et al., 2020, 4D printing of high-performance thermal-responsive liquid metal elastomers driven by embedded microliquid chambers. ACS Appl Mater Interfaces, 12(10): 12068–12074. https://doi.org/10.1021/acsami.9b22433
Tang ZH, Gao ZW, Jia SH, et al., 2017, Graphene-based polymer bilayers with superior light-driven properties for remote construction of 3D structures. Adv Sci, 4(5): 1600437. https://doi.org/10.1002/advs.201600437
Lee AY, Zhou A, An J, et al., 2020, Contactless reversible 4D-printing for 3D-to-3D shape morphing. Virtual Phys Prototyp, 15(4): 481–495. https://doi.org/10.1080/17452759.2020.1822189
Deng H, Zhang C, Sattari K, et al., 2021, 4D printing elastic composites for strain-tailored multistable shape morphing. ACS Appl Mater Interfaces, 13(11): 12719–12725. https://doi.org/10.1021/acsami.0c17618
Qu G, Huang J, Li Z, et al., 2022, 4D-printed bilayer hydrogel with adjustable bending degree for enteroatmospheric fistula closure. Mater Today Bio, 16: 100363. https://doi.org/10.1016/j.mtbio.2022.100363
Hagaman DE, Leist S, Zhou J, et al., 2018, Photoactivated polymeric bilayer actuators fabricated via 3D printing. ACS Appl Mater Interfaces, 10(32): 27308–27315. https://doi.org/10.1021/acsami.8b08503
Liu Y, Lei Y, Hua L, et al., 2021, Biomimetic self-deformation of polymer interpenetrating network with stretch-induced anisotropicity. Chem Mater, 33(21): 8351–8359. https://doi.org/10.1021/acs.chemmater.1c02639
Athanasopoulos N, Siakavellas NJ, 2018, Bioinspired temperature-responsive multilayer films and their performance under thermal fatigue. Biomimetics, 3(3): 20. https://doi.org/10.3390/biomimetics3030020
Ding A, Jeon O, Tang R, et al., 2021, Cell-laden multiple-step and reversible 4D hydrogel actuators to mimic dynamic tissue morphogenesis. Adv Sci, 8(9): 2004616. https://doi.org/10.1002/advs.202004616
Ding AX, Jeon O, Cleveland D, et al., 2022, Jammed micro-flake hydrogel for four-dimensional living cell bioprinting. Adv Mater, 34(15): 2109394. https://doi.org/10.1002/adma.202109394
Ding AX, Lee SJ, Ayyagari S, et al., 4D biofabrication via instantly generated graded hydrogel scaffolds. Bioact Mater, 7: 324–332. https://doi.org/10.1016/j.bioactmat.2021.05.021
Ren L, Li B, He Y, et al., 2020, Programming shape-morphing behavior of liquid crystal elastomers via parameter-encoded 4D printing. ACS Appl Mater Interfaces, 12(13): 15562–15572. https://doi.org/10.1021/acsami.0c00027
Wu P, Yu T, Chen M, et al., 2022, Effect of printing speed and part geometry on the self-deformation behaviors of 4D printed shape memory PLA using FDM. J Manuf Process, 84: 1507–1518. https://doi.org/10.1016/j.jmapro.2022.11.007
Zhao W, Li N, Liu LW, et al., 2022, Origami derived self-assembly stents fabricated via 4D printing. Compos Struct, 293: 115669. https://doi.org/10.1016/j.compstruct.2022.115669
Tao R, Ji LT, Li Y, et al., 2020, 4D printed origami metamaterials with tunable compression twist behavior and stress-strain curves. Compos Part B Eng, 201: 108344. https://doi.org/10.1016/j.compositesb.2020.108344
Zhang YJ, Wang LC, Song WL, et al., 2020, Hexagon-twist frequency reconfigurable antennas via multi-material printed thermo-responsive origami structures. Front Mater, 7: 600863. https://doi.org/10.3389/fmats.2020.600863
Xin XZ, Liu LW, Liu YJ, et al., 2020, Origami-inspired self-deployment 4D printed honeycomb sandwich structure with large shape transformation. Smart Mater Struct, 29(6): 065015. https://doi.org/10.1088/1361-665X/ab85a4
Yamamura S, Iwase E, 2021, Hybrid hinge structure with elastic hinge on self-folding of 4D printing using a fused deposition modeling 3D printer. Mater Des, 203: 109605. https://doi.org/10.1016/j.matdes.2021.109605
Van Manen T, Janbaz S, Jansen KMB, et al., 2021, 4D printing of reconfigurable metamaterials and devices. Commun Mater, 2(1): 56. https://doi.org/10.1038/s43246-021-00165-8
Ge Q, Dunn CK, Qi HJ, et al., 2014, Active origami by 4D printing. Smart Mater Struct, 23(9): 094007. https://doi.org/10.1088/0964-1726/23/9/094007
Yin JC, Fan WX, Xu ZH, et al., 2022, Precisely defining local gradients of stimuli-responsive hydrogels for complex 2D-to-4D shape evolutions. Small, 18(2): 2104440. https://doi.org/10.1002/smll.202104440
Liu G, Zhao Y, Wu G, et al., 2018, Origami and 4D printing of elastomer-derived ceramic structures. Sci Adv, 4(8): eaat0641. https://doi.org/10.1126/sciadv.aat0641
Langford T, Mohammed A, Essa K, et al., 2021, 4D printing of origami structures for minimally invasive surgeries using functional scaffold. Appl Sci Basel, 11(1): 332. https://doi.org/10.3390/app11010332
Kim D, Kim T, Lee YG, 2019, 4D printed bifurcated stents with Kirigami-inspired structures. J Vis Exp, 149: e59746. https://doi.org/10.3791/59746
Ge Q, Qi HJ, Dunn ML, 2013, Active materials by four-dimension printing. Appl Phys Lett, 103(13): 131901. https://doi.org/10.1063/1.4819837
Varga LG, Takacs JD, Domotor FD, et al., 2018, Tailor-made 3D model design of human implants for additive technologies. Proceedings of the 23rd International Conference on Manufacturing (Manufacturing). https://doi.org/10.1088/1757-899x/448/1/012060
Lee MY, Chang CC, Lin CC, et al., 2002, Custom implant design for patients with cranial defects. IEEE Eng Med Biol Mag, 21(2): 38–44. https://doi.org/10.1109/memb.2002.1000184
You D, Chen G, Liu C, et al., 2021, 4D printing of multi-responsive membrane for accelerated in vivo bone healing via remote regulation of stem cell fate. Adv Funct Mater, 31(40): 2103920. https://doi.org/10.1002/adfm.202103920
Cui C, Kim D-O, Pack MY, et al., 2020, 4D printing of self-folding and cell-encapsulating 3D microstructures as scaffolds for tissue-engineering applications. Biofabrication, 12(4): 045018. https://doi.org/10.1088/1758-5090/aba502
Wang Y, Cui H, Wang Y, et al., 4D printed cardiac construct with aligned myofibers and adjustable curvature for myocardial regeneration. ACS Appl Mater Interfaces, 13(11): 12746–12758. https://doi.org/10.1021/acsami.0c17610
Zhang C, Cai D, Liao P, et al., 2021, 4D printing of shape-memory polymeric scaffolds for adaptive biomedical implantation. Acta Biomater, 122: 101–110. https://doi.org/10.1016/j.actbio.2020.12.042
Lin C, Lv J, Li Y, et al., 2019, 4D-printed biodegradable and remotely controllable shape memory occlusion devices. Adv Funct Mater, 29(51): 1906569. https://doi.org/10.1002/adfm.201906569
Hearon K, Wierzbicki MA, Nash LD, et al., 2015, A processable shape memory polymer system for biomedical applications. Adv Healthc Mater, 4(9): 1386–1398. https://doi.org/10.1002/adhm.201500156
Hu Y, Wang Z, Jin D, et al., 2020, Botanical-inspired 4D printing of hydrogel at the microscale. Adv Funct Mater, 30(4): 1907377. https://doi.org/10.1002/adfm.201907377
Zhou W, Duan Z, Zhao J, et al., 2022, Glucose and MMP- 9 dual-responsive hydrogel with temperature sensitive self-adaptive shape and controlled drug release accelerates diabetic wound healing. Bioact Mater, 17: 1–17. https://doi.org/10.1016/j.bioactmat.2024.01.004
Zhang F, Wang L, Zheng Z, et al., 2019, Magnetic programming of 4D printed shape memory composite structures. Compos Part A Appl Sci Manuf, 125: 105571. https://doi.org/10.1016/j.compositesa.2019.105571
Zheng Y, Han MKL, Jiang Q, et al., 2020, 4D hydrogel for dynamic cell culture with orthogonal, wavelength-dependent mechanical and biochemical cues. Mater Horiz, 7(1): 111–116. https://doi.org/10.1039/c9mh00665f
Rosales AM, Anseth KS, 2016, The design of reversible hydrogels to capture extracellular matrix dynamics. Nat Rev Mater, 1(2): 15012. https://doi.org/10.1038/natrevmats.2015.12
Farrukh A, Paez JI, del Campo A, 2019, 4D biomaterials for light-guided angiogenesis. Adv Funct Mater, 29(6): 1807734. https://doi.org/10.1002/adfm.201807734
Liu L, Shadish JA, Arakawa CK, et al., 2018, Cyclic stiffness modulation of cell-laden protein-polymer hydrogels in response to user-specified stimuli including light. Adv Biosyst, 2(12): 1800240. https://doi.org/10.1002/adbi.201800240
Douillet C, Nicodeme M, Hermant L, et al., 2022, From local to global matrix organization by fibroblasts: A 4D laser-assisted bioprinting approach. Biofabrication, 14(2): 025006. https://doi.org/10.1088/1758-5090/ac40ed
Diaz-Payno PJ, Kalogeropoulou M, Muntz I, et al., 2022, Swelling-dependent shape-based transformation of a human mesenchymal stromal cells-laden 4D bioprinted construct for cartilage tissue engineering. Adv Healthc Mater, 12(2): 2201891. https://doi.org/10.1002/adhm.202201891
Arakawa CK, Badeau BA, Zheng Y, et al., 2017, Multicellular vascularized engineered tissues through user-programmable biomaterial photodegradation. Adv Mater, 29(37): 1703156. https://doi.org/10.1002/adma.201703156
Hendrikson WJ, Rouwkema J, Clementi F, et al., 2017, Towards 4D printed scaffolds for tissue engineering: Exploiting 3D shape memory polymers to deliver time-controlled stimulus on cultured cells. Biofabrication, 9(3): 031001. https://doi.org/10.1088/1758-5090/aa8114
Chen M, Li L, Xia L, et al., 2020, Temperature responsive shape-memory scaffolds with circumferentially aligned nanofibers for guiding smooth muscle cell behavior. Macromol Biosci, 20(2): 1900312. https://doi.org/10.1002/mabi.201900312
Miao S, Cui H, Esworthy T, et al., 2020, 4D self-morphing culture substrate for modulating cell differentiation. Adv Sci, 7(6): 1902403. https://doi.org/10.1002/advs.201902403
Zhao Q, Wang J, Wang Y, et al., 2020, A stage-specific cell-manipulation platform for inducing endothelialization on demand. Natl Sci Rev, 7(3): 629–643. https://doi.org/10.1093/nsr/nwz188
Zhao W, Huang Z, Liu L, et al., 2021, Porous bone tissue scaffold concept based on shape memory PLA/Fe3O4. Compos Sci Technol, 203: 108563. https://doi.org/10.1016/j.compscitech.2020.108563
Pandey A, Singh G, Singh S, et al., 2020, 3D printed biodegradable functional temperature-stimuli shape memory polymer for customized scaffoldings. J Mech Behav Biomed Mater, 108: 103781. https://doi.org/10.1016/j.jmbbm.2020.103781
Wang J, Gao H, Hu Y, et al., 2021, 3D printing of pickering emulsion inks to construct poly(D,L-lactide-co-trimethylene carbonate)-based porous bioactive scaffolds with shape memory effect. J Mater Sci, 56(1): 731–745. https://doi.org/10.1007/s10853-020-05318-7
Hwangbo H, Lee H, Roh EJ, et al., 2021, Bone tissue engineering via application of a collagen/hydroxyapatite 4D-printed biomimetic scaffold for spinal fusion. Appl Phys Rev, 8(2): 021403. https://doi.org/10.1063/5.0035601
Liverani L, Liguori A, Zezza P, et al., 2022, Nanocomposite electrospun fibers of poly(epsilon-caprolactone)/bioactive glass with shape memory properties. Bioact Mater, 11: 230–239. https://doi.org/10.1016/j.bioactmat.2021.09.020
Kabirian F, Mela P, Heying R, 2022, 4D Printing applications in the development of smart cardiovascular implants. Front Bioeng Biotechnol, 10: 873453. https://doi.org/10.3389/fbioe.2022.873453
Briggs S, Herting S, Fletcher G, et al., 2022, Mechanical and shape memory properties of electrospun polyurethane with thiol-ene crosslinking. Nanomaterials, 12(3): 406. https://doi.org/10.3390/nano12030406
Zhou Y, Zhou D, Cao P, et al., 2021, 4D printing of shape memory vascular stent based on beta CD-g-polycaprolactone. Macromol Rapid Commun, 42(14): 2100176. https://doi.org/10.1002/marc.202100176
Kuang X, Chen K, Dunn CK, et al., 2018, 3D printing of highly stretchable, shape-memory, and self-healing elastomer toward novel 4D printing. ACS Appl Mater Interfaces, 10(8): 7381–7388. https://doi.org/10.1021/acsami.7b18265
Lee Y, Ceylan H, Yasa IC, et al., 2021, 3D-printed multi-stimuli-responsive mobile micromachines. ACS Appl Mater Interfaces, 13(11): 12759–12766. https://doi.org/10.1021/acsami.0c18221
Feng J, Shi H, Yang X, et al., 2021, Self-adhesion conductive sub-micron fiber cardiac patch from shape memory polymers to promote electrical signal transduction function. ACS Appl Mater Interfaces, 13(17): 19593–19602. https://doi.org/10.1021/acsami.0c22844
Lin C, Liu L, Liu Y, et al., 2021, 4D printing of bioinspired absorbable left atrial appendage occluders: A proof-of-concept study. ACS Appl Mater Interfaces, 13(11): 12668– 12678. https://doi.org/10.1021/acsami.0c17192
Lin C, Huang Z, Wang Q, et al., 2022, 4D printing of overall radiopaque customized bionic occlusion devices. Adv Healthc Mater, 12(4): 2201999. https://doi.org/10.1002/adhm.202201999
Haykal S, Salna M, Waddell TK, et al., 2014, Advances in tracheal reconstruction. Plast Reconstruct Surg Glob Open, 2(7): e178. https://doi.org/10.1097/gox.0000000000000097
Murgu SD, Colt HG, 2006, Tracheobronchomalacia and excessive dynamic airway collapse. Respirology, 11(4): 388–406. https://doi.org/10.1111/j.1440-1843.2006.00862.x
Freitag L, Goerdes M, Zarogoulidis P, et al., 2017, Towards individualized tracheobronchial stents: Technical, practical and legal considerations. Respiration, 94(5): 442–456. https://doi.org/10.1159/000479164
Maity N, Mansour N, Chakraborty P, et al., 2021, A personalized multifunctional 3D printed shape memory-displaying, drug releasing tracheal stent. Adv Funct Mater, 31(50): 2108436. https://doi.org/10.1002/adfm.202108436
Zarek M, Mansour N, Shapira S, et al., 2017, 4D printing of shape memory-based personalized endoluminal medical devices. Macromol Rapid Commun, 38(2): 1600628. https://doi.org/10.1002/marc.201600628
Zhang F, Wen N, Wang L, et al., 2021, Design of 4D printed shape-changing tracheal stent and remote controlling actuation. Int J Smart Nano Mater, 12(4): 375–389. https://doi.org/10.1080/19475411.2021.1974972
Zhao W, Zhang F, Leng J, et al., 2019, Personalized 4D printing of bioinspired tracheal scaffold concept based on magnetic stimulated shape memory composites. Compos Sci Technol, 184: 107866. https://doi.org/10.1016/j.compscitech.2019.107866
Pandey H, Mohol SS, Kandi R, 2022, 4D printing of tracheal scaffold using shape-memory polymer composite. Mater Lett, 329: 133238. https://doi.org/10.1016/j.matlet.2022.133238
Yang GH, Kim W, Kim J, et al., 2021, A skeleton muscle model using GelMA-based cell-aligned bioink processed with an electric-field assisted 3D/4D bioprinting. Theranostics, 11(1): 48–63. https://doi.org/10.7150/thno.50794
Cui H, Miao S, Esworthy T, et al., 2019, A novel near-infrared light responsive 4D printed nanoarchitecture with dynamically and remotely controllable transformation. Nano Res, 12(6): 1381–1388. https://doi.org/10.1007/s12274-019-2340-9
Esworthy TJ, Miao S, Lee S-J, et al., 2019, Advanced 4D-bioprinting technologies for brain tissue modeling and study. Int J Smart Nano Mater, 10(3): 177–204. https://doi.org/10.1080/19475411.2019.1631899
Fang J-H, Hsu H-H, Hsu R-S, et al., 2020, 4D printing of stretchable nanocookie@conduit material hosting biocues and magnetoelectric stimulation for neurite sprouting. NPG Asia Mater, 12(1): 61. https://doi.org/10.1038/s41427-020-00244-1
Deng Y, Yang B, Zhang F, et al., 2022, 4D printed orbital stent for the treatment of enophthalmic invagination. Biomaterials, 291: 121886. https://doi.org/10.1016/j.biomaterials.2022.121886
Miao S, Castro N, Nowicki M, et al., 2017, 4D printing of polymeric materials for tissue and organ regeneration. Mater Today, 20(10): 577–591. https://doi.org/10.1016/j.mattod.2017.06.005
Zu S, Wang Z, Zhang S, et al., 2022, A bioinspired 4D printed hydrogel capsule for smart controlled drug release. Mater Today Chem, 24: 100789. https://doi.org/10.1016/j.mtchem.2022.100789
Zu S, Zhang Z, Liu Q, et al., 2022, 4D printing of core-shell hydrogel capsules for smart controlled drug release. Bio-Des Manuf, 5(2): 294–304. https://doi.org/10.1007/s42242-021-00175-y
Zhang Y, Yang G, Hayat U, et al., 2023, Water-responsive 4D printing based on self-assembly of hydrophobic protein “Zein” for the control of degradation rate and drug release. Bioact Mater, 23: 343–352. https://doi.org/10.1016/j.bioactmat.2022.11.009