Multimaterial and multiscale scaffold for engineering enthesis organ
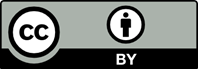
Tendon and ligament injuries are relevant clinical problems in modern society, and the current medical approaches do not guarantee complete recovery of the physiological functionalities. Moreover, they present a non-negligible failure rate after surgery. Failures often occur at the enthesis, which is the area of tendons and ligaments insertion to bones. This area is highly anisotropic and composed of four distinct zones: tendon or ligament, non-mineralized fibrocartilage, mineralized fibrocartilage, and bone. The organization of these regions provides a gradient in mechanical properties, biochemical composition, cellular phenotype, and extracellular matrix organization. Tissue engineering represents an alternative to traditional medical approaches. This work presents a novel biofabrication approach for engineering the enthesis. Gradient-based scaffolds were fabricated by exploiting the combination of electrospinning and three-dimensional (3D) bioprinting technologies. Studies were conducted to evaluate scaffold biocompatibility by seeding bone marrow-derived mesenchymal stem cells (BM-MSCs). Then, the scaffold’s ability to promote cellular adhesion, growth, proliferation, and differentiation in both tenogenic and osteogenic phenotypes was evaluated. Fabricated scaffolds were also morphologically and mechanically characterized, showing optimal properties comparable to literature data. The versatility and potentiality of this novel biofabrication approach were demonstrated by fabricating clinical-size 3D enthesis scaffolds. The mechanical characterization highlighted their behavior during a tensile test was comparable to tendons and ligaments in vivo.
Benjamin M, Kumai T, Milz S, et al., 2002, The skeletal attachment of tendons - Tendon ‘entheses’. Comp Biochem Physiol, 133(4): 931–945. https://doi.org/10.1016/S1095-6433(02)00138-1
Paxton JZ, Baar K, Grover LM, 2013, Current progress in enthesis repair: Strategies for interfacial tissue engineering. Orthop Muscular Syst, 01(S1): 1–13. https://doi.org/10.4172/2161-0533.s1-003
Golman M, Birman V, Thomopoulos S, 2021, Enthesis strength, toughness and stiffness: An image-based model comparing tendon insertions with varying bony attachment geometries. J R Soc Interface, 18(185). https://doi.org/10.1098/rsif.2021.0421
Benjamin M, Ralphs JR, 1998, Fibrocartilage in tendons and ligaments - An adaptation to compressive load. J Anat, 193(4): 481–494. https://doi.org/10.1017/S0021878298004300
Buenzli PR, Sims NA, 2015, Quantifying the osteocyte network in the human skeleton. Bone, 75: 144–150. https://doi.org/10.1016/j.bone.2015.02.016
Schwartz AG, Long F, Thomopoulos S, 2015, Enthesis fibrocartilage cells originate from a population of hedgehog-responsive cells modulated by the loading environment. Development, 142(1): 196–206. https://doi.org/10.1242/dev.112714
Benjamin M, McGonagle D, 2009, The enthesis organ concept and its relevance to the spondyloarthropathies. Adv Exp Med Biol, 649: 57–70. https://doi.org/10.1007/978-1-4419-0298-6_4
Foolen J, Wunderli SL, Loerakker S, et al., 2018, Tissue alignment enhances remodeling potential of tendon-derived cells - Lessons from a novel microtissue model of tendon scarring. Matrix Biol, 65: 14–29. https://doi.org/10.1016/j.matbio.2017.06.002
Nowlin J, Bismi MA, Delpech B, et al., 2018, Engineering the hard–soft tissue interface with random-to-aligned nanofiber scaffolds. Nanobiomedicine, 5: 184954351880353. https://doi.org/10.1177/1849543518803538
Xie J, Ma B, Michael PL, et al., 2012, Fabrication of nanofiber scaffolds with gradations in fiber organization and their potential applications. Macromol Biosci, 12(10): 1336–1341. https://doi.org/10.1002/mabi.201200115
Xiong J, Wang H, Lan X, et al., 2022, Fabrication of bioinspired grid-crimp micropatterns by melt electrospinning writing for bone-ligament interface study. Biofabrication, 14(2): 025008–025008. https://doi.org/10.1088/1758-5090/ac4ac8
Criscenti G, Longoni A, Di Luca A, et al., 2016, Triphasic scaffolds for the regeneration of the bone-ligament interface. Biofabrication, 8(1): 15009. https://doi.org/10.1088/1758-5090/8/1/015009
Silva M, Ferreira FN, Alves NM, et al., 2020, Biodegradable polymer nanocomposites for ligament/tendon tissue engineering. J Nanobiotechnol, 18(1): 1–33. https://doi.org/10.1186/s12951-019-0556-1
Barber JG, Handorf AM, Allee TJ, et al., 2013, Braided nanofibrous scaffold for tendon and ligament tissue engineering. Tissue Eng Part A, 19(11–12): 1265–1274. https://doi.org/10.1089/ten.tea.2010.0538
Sahoo S, Ouyang H, James CH, et al., 2006, Characterization of a novel polymeric scaffold for potential application in tendon/ligament tissue engineering. Tissue Eng, 12(1): 91–99. https://doi.org/10.1089/ten.2006.12.91
Jayasree A, Thankappan SK, Ramachandran R, et al., 2019, Bioengineered braided micro-nano (multiscale) fibrous scaffolds for tendon reconstruction. ACS Biomater Sci Eng, 5(3): 1476–1486. https://doi.org/10.1021/acsbiomaterials.8b01328
Tsutsumi H, Kurimoto R, Nakamichi R, et al., 2022, Generation of a tendon-like tissue from human iPS cells. J Tissue Eng, 13: 1–5. https://doi.org/10.1177/20417314221074018
Costa-Almeida R, Calejo I, Gomes ME, 2019, Mesenchymal stem cells empowering tendon regenerative therapies. Int J Mol Sci, 20(12): 3002–3002. https://doi.org/10.3390/ijms20123002
Trincavelli ML, Daniele S, Giacomelli C, et al., 2014, Osteoblast differentiation and survival: A role for A2B adenosine receptor allosteric modulators. Biochim Biophys Acta Mol Cell Res, 1843(12): 2957–2966. https://doi.org/10.1016/j.bbamcr.2014.09.013
Luo W, Wang Y, Han Q, et al., 2022, Advanced strategies for constructing interfacial tissues of bone and tendon/ ligament. J Tissue Eng, 13: 1–31. https://doi.org/10.1177/20417314221144714
Yang G, Lin H, Rothrauff BB, et al., 2016, Multilayered polycaprolactone/gelatin fiber-hydrogel composite for tendon tissue engineering. Acta Biomater, 35: 68–76. https://doi.org/10.1016/j.actbio.2016.03.004
Cao Y, Yang G, Lin H, et al., 2020, Three-dimensional printed multiphasic scaffolds with stratified cell-laden gelatin methacrylate hydrogels for biomimetic tendon-to-bone interface engineering. J Orthop Transl, 23(January): 89–100. https://doi.org/10.1016/j.jot.2020.01.004
Yin Z, Chen X, Chen JL, et al., 2010, The regulation of tendon stem cell differentiation by the alignment of nanofibers. Biomaterials, 31(8): 2163–2175. https://doi.org/10.1016/j.biomaterials.2009.11.083
Chen YC, Lin R-Z, Qi H, et al., 2012, Functional human vascular network generated in photocrosslinkable gelatin methacrylate hydrogels. Adv Funct Mater, 22(10): 2027– 2039. https://doi.org/10.1002/adfm.201101662
Pulidori E, Micalizzi S, Bramanti E, et al., 2021, One-pot process: Microwave-assisted keratin extraction and direct electrospinning to obtain keratin-based bioplastic. Int J Mol Sci, 22(17): 9597. https://doi.org/10.3390/ijms22179597
Nichol JW, Koshy ST, Bae H, et al., 2010, Cell-laden microengineered gelatin methacrylate hydrogels. Biomaterials, 31(21): 5536–5544. https://doi.org/10.1016/j.biomaterials.2010.03.064
Maniruzzaman M, Boateng JS, Snowden MJ, et al., 2012, A review of hot-melt extrusion: process technology to pharmaceutical products. ISRN Pharm, 2012: 1–9. https://doi.org/10.5402/2012/436763
Temple JP, Hutton DL, Hung BP, et al., 2014, Engineering anatomically shaped vascularized bone grafts with hASCs and 3D-printed PCL scaffolds. J Biomed Mater Res Part A, 102(12): 4317–4325. https://doi.org/10.1002/jbm.a.35107
Amiel D, Frank C, Harwood F, et al., 1984, Tendons and ligaments: A morphological and biochemical comparison. J Orthop Res, 1: 251–265.
You Y, Lee SJ, Min BM, et al., 2006, Effect of solution properties on nanofibrous structure of electrospun poly(lactic-co-glycolic acid). J Appl Polym Sci, 99(3): 1214– 1221. https://doi.org/10.1002/app.22602
Sato K, Yu Q, Hiramoto J, et al., 2015, A method to investigate strain rate effects on necking and fracture behaviors of advanced high-strength steels using digital imaging strain analysis. Int J Impact Eng, 75: 11–26. https://doi.org/10.1016/j.ijimpeng.2014.07.001
Peters WH, Ranson WF, 1982, Digital imaging techniques in experimental stress analysis. Opt Eng, 21(3): 213427.
Barresi E, Giacomelli C, Marchetti L, et al., 2021, Novel positive allosteric modulators of A2B adenosine receptor acting as bone mineralisation promoters. J Enzyme Inhib Med Chem, 36(1): 286–294. 10.1080/14756366.2020.1862103
Sry V, Mizutani Y, Endo G, et al., 2018, Estimation of the longitudinal elasticity modulus of braided synthetic fiber rope utilizing classical laminate theory with the unit N/tex. Appl Sci, 8(7): 1096–1096. https://doi.org/10.3390/app8071096
Kempfert M, Willbold E, Loewner S, et al., 2022, Polycaprolactone-based 3D-printed scaffolds as potential implant materials for tendon-defect repair. J Funct Biomater, 13(4): 160. https://doi.org/10.3390/jfb13040160
Guedes F, Branquinho MV, Biscaia S, et al., 2022, Gamma irradiation processing on 3D PCL devices: A preliminary biocompatibility assessment. Int J Mol Sci, 23(24): 15916–15916. https://doi.org/10.3390/ijms232415916
Giacomelli C, Natali L, Nisi M, et al., 2018, Negative effects of a high tumour necrosis factor-α concentration on human gingival mesenchymal stem cell trophism: The use of natural compounds as modulatory agents. Stem Cell Res Ther, 9(1): 1–21. https://doi.org/10.1186/s13287-018-0880-7
Bowers K, Amelse L, Bow A, et al., 2022, Mesenchymal stem cell use in acute tendon injury: In vitro tenogenic potential vs. in vivo dose response. Bioengineering, 9(8): 407–407. https://doi.org/10.3390/bioengineering9080407
Zhao Y, Sun Q, Wang S, et al., 2019, Spreading shape and area regulate the osteogenesis of mesenchymal stem cells. Tissue Eng Regen Med, 16(6): 573–583. https://doi.org/10.1007/s13770-019-00213-y
Khan AU, Qu R, Fan T, et al., 2020, A glance on the role of actin in osteogenic and adipogenic differentiation of mesenchymal stem cells. Stem Cell Res Ther, 11(1): 1–14. https://doi.org/10.1186/s13287-020-01789-2
Siadat SM, Silverman AA, DiMarzio CA, et al., 2021, Measuring collagen fibril diameter with differential interference contrast microscopy. J Struct Biol, 213(1): 1–17. https://doi.org/10.1016/j.jsb.2021.107697
Nazeri N, Derakhshan MA, Faridi-Majidi R, et al., 2018, Novel electro-conductive nanocomposites based on electrospun PLGA/CNT for biomedical applications. J Mater Sci Mater Med, 29(11): 1–9. https://doi.org/10.1007/s10856-018-6176-8
Chou SF,. Woodrow KA, 2017, Relationships between mechanical properties and drug release from electrospun fibers of PCL and PLGA blends. J Mech Behav Biomed Mater, 65(September 2016): 724–733. https://doi.org/10.1016/j.jmbbm.2016.09.004
Górecka Ż, Idaszek J, Kołbuk D, et al., 2020, The effect of diameter of fibre on formation of hydrogen bonds and mechanical properties of 3D-printed PCL. Mater Sci Eng C, 114(April): 111072. https://doi.org/10.1016/j.msec.2020.111072
Brennan DA, Conte AA, Kanski G, et al., 2018, Mechanical considerations for electrospun nanofibers in tendon and ligament repair. Adv Healthc Mater, 7(12): 1–31. https://doi.org/10.1002/adhm.201701277
Shin HJ, Lee CH, Cho IH, et al., 2006, Electrospun PLGA nanofiber scaffolds for articular cartilage reconstruction: Mechanical stability, degradation and cellular responses under mechanical stimulation in vitro. J Biomater Sci Polym Ed, 17(1): 103–119. https://doi.org/10.1163/156856206774879126
Sperling LE, Reis KP, Pozzobon LG, et al., 2017, Influence of random and oriented electrospun fibrous poly(lactic-co-glycolic acid) scaffolds on neural differentiation of mouse embryonic stem cells. J Biomed Mater Res Part A, 105(5): 1333–1345. https://doi.org/10.1002/jbm.a.36012
Balestri W, Hickman GJ, Morris RH, et al., 2023, Triphasic 3D in vitro model of bone-tendon-muscle interfaces to study their regeneration. Cells, 12(2): 313–313. https://doi.org/10.3390/cells12020313
Fazeli N, Arefian E, Irani S, et al., 2021, 3D-printed PCL scaffolds coated with nanobioceramics enhance osteogenic differentiation of stem cells. ACS Omega, 6(51): 35284– 35296. https://doi.org/10.1021/acsomega.1c04015
Ramakrishna H, Li T, He T, et al., 2019, Tissue engineering a tendon-bone junction with biodegradable braided scaffolds. Biomater Res, 23(1): 1–12. https://doi.org/10.1186/s40824-019-0160-3
Rinoldi C, Fallahi A, Yazdi IK, et al., 2019, Mechanical and biochemical stimulation of 3D multilayered scaffolds for tendon tissue engineering. ACS Biomater Sci Eng, 5(6): 2953–2964. https://doi.org/10.1021/acsbiomaterials.8b01647
De Maria C, Giusti S, Mazzei D, et al., 2011, Squeeze pressure bioreactor: A hydrodynamic bioreactor for noncontact stimulation of cartilage constructs. Tissue Eng Part C Methods, 17(7): 757–764. https://doi.org/10.1089/ten.tec.2011.0002
Banik BL., Brown JL, 2020, 3D-printed bioreactor enhances potential for tendon tissue engineering. Regen Eng Transl Med, 6(4): 419–428. https://doi.org/10.1007/s40883-019-00145-y