Blood-derived biomaterials for tissue graft biofabrication by solvent-based extrusion bioprinting
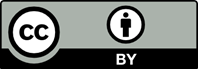
This article provides an overview of the different types of blood-derived biomaterials that can be used as solvent additives in the formulation of inks/bioinks for use in solvent extrusion printing/bioprinting. We discuss the properties of various blood sub-products obtained after blood fractionation in terms of their use in tailoring ink/bioink to produce functional constructs designed to improve tissue repair. Blood-derived additives include platelets and/or their secretome, including signaling proteins and microvesicles, which can drive cell migration, inflammation, angiogenesis, and synthesis of extracellular matrix proteins. The contribution of plasma to ink/bioink functionalization relies not only on growth factors, such as hepatocyte growth factor and insulin growth factors, but also on adhesive proteins, such as fibrinogen/fibrin, vitronectin, and fibronectin. We review the current developments and progress in solvent-based extrusion printing/bioprinting with inks/bioinks functionalized with different blood-derived products, leading toward the development of more advanced patient-specific 3D constructs in multiple medical fields, including but not limited to oral tissues and cartilage, bone, skin, liver, and neural tissues. This information will assist researchers in identifying the most suitable blood-derived product for their ink/bioink formulation based on the intended regenerative functionality of the target tissue.
Groll J, Boland T, Blunk T, et al., 2016, Biofabrication: Reappraising the definition of an evolving field. Biofabrication, 8(1): 013001. https://doi.org/10.1088/1758-5090/8/1/013001
Mironov V, Trusk T, Kasyanov V, et al., 2009, Biofabrication: A 21st century manufacturing paradigm. Biofabrication, 1: 022001. https://doi.org/10.1088/1758-5082/1/2/022001
Zhang B, Cristescu R, Chrisey DB, et al., 2020, Solvent-based extrusion 3D printing for the fabrication of tissue engineering scaffolds. Int J Bioprint, 6: 28–42. https://doi.org/10.18063/IJB.V6I1.211
De¸bski T, Kurzyk A, Ostrowska B, et al., 2020, Scaffold vascularization method using an adipose-derived stem cell (ASC)-seeded scaffold prefabricated with a flow-through pedicle. Stem Cell Res Ther, 11: 1–14. https://doi.org/10.1186/S13287-019-1535-Z/FIGURES/7
Lee J, Park D, Seo Y, et al., 2020, Organ-level functional 3D tissue constructs with complex compartments and their preclinical applications. Adv Mater, 32: 2002096. https://doi.org/10.1002/ADMA.202002096
Deo KA, Singh KA, Peak CW, et al., 2020, Bioprinting 101: Design, fabrication, and evaluation of cell-laden 3D bioprinted scaffolds. Tissue Eng - Part A, 26: 318–338. https://doi.org/10.1089/TEN.TEA.2019.0298/ASSET/ IMAGES/LARGE/TEN.TEA.2019.0298_FIGURE6.JPEG
Supernat A, Popęda M, Pastuszak K, et al., 2021, Transcriptomic landscape of blood platelets in healthy donors. Sci Rep, 11: 15679. https://doi.org/10.1038/s41598-021-94003-z
Nieto D, Jiménez G, Moroni L, et al., 2022, Biofabrication approaches and regulatory framework of metastatic tumor‐on‐a‐chip models for precision oncology. Med Res Rev, 42: 1978–2001 https://doi.org/10.1002/MED.21914
Burdis R, Chariyev-Prinz F, Browe DC, et al., 2022, Spatial patterning of phenotypically distinct microtissues to engineer osteochondral grafts for biological joint resurfacing. Biomaterials, 289: 121750. https://doi.org/10.1016/J.BIOMATERIALS.2022.121750
Zhang Q, Nguyen PD, Shi S, et al., 2018, 3D bio-printed scaffold-free nerve constructs with human gingiva-derived mesenchymal stem cells promote rat facial nerve regeneration. Sci Rep, 8: 1–11. https://doi.org/10.1038/s41598-018-24888-w
Chen EP, Toksoy Z, Davis BA, et al., 2021, 3D bioprinting of vascularized tissues for in vitro and in vivo applications. Front Bioeng Biotechnol, 9, 1–22. https://doi.org/10.3389/fbioe.2021.664188
Kandi R, Sachdeva K, Choudhury SD, et al., 2023, A facile 3D bio-fabrication of customized tubular scaffolds using solvent-based extrusion printing for tissue-engineered tracheal grafts. J Biomed Mater Res Part A, 111: 278–293. https://doi.org/10.1002/JBM.A.37458
Moss SM, Schilp J, Yaakov M, et al., 2022, Point-of-use, automated fabrication of a 3D human liver model supplemented with human adipose microvessels. SLAS Discov, 27: 358–68 https://doi.org/10.1016/j.slasd.2022.06.003
Nulty J, Freeman FE, Browe DC, et al., 2021, 3D bioprinting of prevascularised implants for the repair of critically-sized bone defects. Acta Biomater, 126: 154–169. https://doi.org/10.1016/J.ACTBIO.2021.03.003
Liang Q, Ma Y, Yao X, et al., 2022, Advanced 3D-printing bioinks for articular cartilage repair. Int J Bioprint, 8: 15–30. https://doi.org/10.18063/ijb.v8i3.511
Yeung E, Inoue T, Matsushita H, et al., 2020, In vivo implantation of 3-dimensional printed customized branched tissue engineered vascular graft in a porcine model. J Thorac Cardiovasc Surg, 159: 1971–1981.e1. https://doi.org/10.1016/J.JTCVS.2019.09.138
Yu HW, Kim BS, Lee JY, et al., 2021, Tissue printing for engineering transplantable human parathyroid patch to improve parathyroid engraftment, integration, and hormone secretion in vivo. Biofabrication, 13(3): 1-10. https://doi.org/10.1088/1758-5090/ABF740
Del Amo C, Perez-valle A, Atilano L, et al., 2022, Unraveling the signaling secretome of platelet-rich plasma: Towards a better understanding of its therapeutic potential in knee osteoarthritis. J Clin Med, 11(3): 473.
Sun B, Lian M, Han Y, et al., 2021, A 3D-bioprinted dual growth factor-releasing intervertebral disc scaffold induces nucleus pulposus and annulus fibrosus reconstruction. Bioact Mater, 6: 179. https://doi.org/10.1016/J.BIOACTMAT.2020.06.022
Lee J, Seok JM, Huh SJ, et al., 2020, 3D printed micro-chambers carrying stem cell spheroids and pro-proliferative growth factors for bone tissue regeneration. Biofabrication, 13: 015011. https://doi.org/10.1088/1758-5090/ABC39C
Ngo TB, Spearman BS, Hlavac N, et al., 2020, Three-dimensional bioprinted hyaluronic acid hydrogel test beds for assessing neural cell responses to competitive growth stimuli. ACS Biomater Sci Eng, 6: 6819–6830. https://doi.org/10.1021/ACSBIOMATERIALS.0C00940/ SUPPL_FILE/AB0C00940_SI_001.PDF
Horstman EE, Tormey CA, 2022, Plasma products for transfusion: An overview. Ann Blood, 7: 1–13. https://doi.org/10.21037/AOB-22-7
Dohan Ehrenfest DM, Andia I, Zumstein MA, et al., 2014, Classification of platelet concentrates (platelet-rich plasma- PRP, platelet-rich fibrin-PRF) for topical and infiltrative use in orthopedic and sports medicine: current consensus, clinical implications and perspectives. Ligaments Tendons J, 4: 3–9.
Burnouf T, Agrahari V, Agrahari V, 2019, Extracellular vesicles as nanomedicine: Hopes and hurdles in clinical translation. Int J Nanomedicine, 14: 8847–8859. https://doi.org/10.2147/IJN.S225453
Ebner-Peking P, Krisch L, Wolf M, et al., 2021, Self-assembly of differentiated progenitor cells facilitates spheroid human skin organoid formation and planar skin regeneration. Theranostics, 11: 8430–8447. https://doi.org/10.7150/THNO.59661
Hermida-Nogueira L, Barrachina MN, Morán LA, et al., 2020, Deciphering the secretome of leukocyte-platelet rich fibrin: Towards a better understanding of its wound healing properties. Sci Rep, 10: 1–11. https://doi.org/10.1038/s41598-020-71419-7
Kardos D, Hornyák I, Simon M, et al., 2018, Biological and mechanical properties of platelet-rich fibrin membranes after thermal manipulation and preparation in a single-syringe closed system. Int J Mol Sci, 19(11): 1–14. https://doi.org/10.3390/ijms19113433
Widyaningrum R, Burnouf T, Nebie O, et al., 2021, A purified human platelet pellet lysate rich in neurotrophic factors and antioxidants repairs and protects corneal endothelial cells from oxidative stress. Biomed Pharmacother, 142: 112046. https://doi.org/10.1016/j.biopha.2021.112046
Andia I, Perez‐valle A, Amo Del C, et al., 2020, Freeze‐drying of platelet‐rich plasma: The quest for standardization. Int J Mol Sci, 21: 1–20. https://doi.org/10.3390/ijms21186904
Nurden AT, Nurden P, Sanchez M, et al., 2008, Platelets and wound healing. Front Biosci, 13: 3532–3548. https://doi.org/10.2741/2947/PDF
Lee HM, Shen EC, Shen JT, et al., 2020, Tensile strength, growth factor content and proliferation activities for two platelet concentrates of platelet-rich fibrin and concentrated growth factor. J Dent Sci, 15: 141–146. https://doi.org/10.1016/J.JDS.2020.03.011
Battinelli EM, Thon JN, Okazaki R, et al., 2019, Megakaryocytes package contents into separate α-granules that are differentially distributed in platelets. Blood Adv, 3: 3092–3098. https://doi.org/10.1182/BLOODADVANCES.2018020834
Ambrosio AL, Di Pietro SM, 2016, Storage pool diseases illuminate platelet dense granule biogenesis. Platelets, 28: 138–146. https://doi.org/10.1080/09537104.2016.1243789
Miroshnychenko O, Chalkley RJ, Leib RD, et al., 2020, Proteomic analysis of platelet-rich and platelet-poor plasma. Regen Ther, 15: 226–235. https://doi.org/10.1016/j.reth.2020.09.004
Johnson J, Wu YW, Blyth C, et al., 2021, Prospective therapeutic applications of platelet extracellular vesicles. Trends Biotechnol, 39: 598–612.
Burnouf T, Goubran HA, 2022, Regenerative effect of expired platelet concentrates in human therapy: An update. Transfus Apher Sci, 61(1): 103363. https://doi.org/10.1016/J.TRANSCI.2022.103363
Vajen T, Benedikter BJ, Heinzmann ACA, et al., 2017, Platelet extracellular vesicles induce a pro-inflammatory smooth muscle cell phenotype. J Extracell Vesicles, 6: 1322454. https://doi.org/10.1080/20013078.2017.1322454/SUPPL_ FILE/ZJEV_A_1322454_SM7826.PDF
Saumell-Esnaola M, Delgado D, García Del Caño G, et al., 2022, Isolation of platelet-derived exosomes from human platelet-rich plasma: biochemical and morphological characterization. Int J Mol Sci, 23: 2861. https://doi.org/10.3390/IJMS23052861
Del Amo C, Fernández-San Argimiro X, Cascajo-Castresana M, et al., 2022, Wound-microenvironment engineering through advanced-dressing bioprinting. Int J Mol Sci, 23(5), 1–19. https://doi.org/10.3390/ijms23052836
Delila L, Wu YW, Nebie O, et al., 2020, Extensive characterization of the composition and functional activities of five preparations of human platelet lysates for dedicated clinical uses. Platelets, 32(2): 259–272. https://doi.org/101080/0953710420201849603
Uchiyama R, Toyoda E, Maehara M, et al., 2021, Effect of platelet-rich plasma on M1/M2 macrophage polarization. Int J Mol Sci, 22: 2336. https://doi.org/10.3390/IJMS22052336
Perez-Valle A, Del Amo C, Andia I, 2020, Overview of current advances in extrusion bioprinting for skin applications. Int J Mol Sci, 21: 1–28. https://doi.org/10.3390/ijms21186679
de Melo BAG, Jodat YA, Mehrotra S, et al., 2019, 3D printed cartilage-like tissue constructs with spatially controlled mechanical properties. Adv Funct Mater, 29: 1–26. https://doi.org/10.1002/adfm.201906330
Zhao M, Wang J, Zhang J, et al., 2022, Functionalizing multi-component bioink with platelet-rich plasma for customized in-situ bilayer bioprinting for wound healing. Mater Today Bio, 16: 100334. https://doi.org/10.1016/J.MTBIO.2022.100334
Zhu B, Wang D, Pan H, et al., 2023, Three-in-one customized bioink for islet organoid: GelMA/ECM/PRP orchestrate pro-angiogenic and immunoregulatory function. Colloids Surf B Biointerfaces, 221: 113017. https://doi.org/10.1016/J.COLSURFB.2022.113017
Zou Q, Grottkau BE, He Z, et al., 2020, Biofabrication of valentine-shaped heart with a composite hydrogel and sacrificial material. Mater Sci Eng C, 108: 110205. https://doi.org/10.1016/j.msec.2019.110205
Ahlfeld T, Cubo-Mateo N, Cometta S, et al., 2020, A novel plasma-based bioink stimulates cell proliferation and differentiation in bioprinted, mineralized constructs. ACS Appl Mater Interfaces, 12: 12557–12572. https://doi.org/10.1021/acsami.0c00710
Cao B, Lin J, Tan J, et al., 2023, 3D-printed vascularized biofunctional scaffold for bone regeneration. Int J Bioprint, 9(3): 702. https://doi.org/10.18063/IJB.702
Hao Y, Cao B, Deng L, et al., 2023, The first 3D-bioprinted personalized active bone to repair bone defects: A case report. Int J Bioprint, 9: 654. https://doi.org/10.18063/IJB.V9I2.654
Wei L, Wu S, Kuss M, et al., 2019, 3D printing of silk fibroin-based hybrid scaffold treated with platelet rich plasma for bone tissue engineering. Bioact Mater, 4: 256–260. https://doi.org/10.1016/j.bioactmat.2019.09.001
Jiang G, Li S, Yu K, et al., 2021, A 3D-printed PRP-GelMA hydrogel promotes osteochondral regeneration through M2 macrophage polarization in a rabbit model. Acta Biomater, 128: 150–162. https://doi.org/10.1016/J.ACTBIO.2021.04.010
Irmak G, Gümüşderelioglu M, 2020, Photo-activated platelet-rich plasma (PRP)-based patient-specific bio-ink for cartilage tissue engineering. Biomed Mater, 15: 065010. https://doi.org/10.1088/1748-605X/ab9e46
Li Z, Zhang X, Yuan T, et al., 2020, Addition of platelet-rich plasma to silk fibroin hydrogel bioprinting for cartilage regeneration. Tissue Eng - Part A, 26: 886–895. https://doi.org/10.1089/ten.tea.2019.0304
Anil Kumar S, Alonzo M, Allen SC, et al., 2019, A visible light-cross-linkable, fibrin-gelatin-based bioprinted construct with human cardiomyocytes and fibroblasts. ACS Biomater Sci Eng, 5: 4551–4563. https://doi.org/10.1021/acsbiomaterials.9b00505
Freeman S, Ramos R, Alexis Chando P, et al., 2019, A bioink blend for rotary 3D bioprinting tissue engineered small-diameter vascular constructs. Acta Biomater, 95: 152–164. https://doi.org/10.1016/J.ACTBIO.2019.06.052
Maiullari F, Costantini M, Milan M, et al., 2018, A multi-cellular 3D bioprinting approach for vascularized heart tissue engineering based on HUVECs and iPSC-derived cardiomyocytes. Sci Rep, 8: 1–15. https://doi.org/10.1038/s41598-018-31848-x
Wang Z, Lee SJ, Cheng H-J, et al., 2018, 3D bioprinted functional and contractile cardiac tissue constructs. Acta Biomater, 70: 48–56. https://doi.org/10.1016/j.actbio.2018.02.007
Frazer H, You J, Chen Z, et al., 2020, Development of a platelet lysate–based printable, transparent biomaterial with regenerative potential for epithelial corneal injuries. Transl Vis Sci Technol, 9: 1–12. https://doi.org/10.1167/TVST.9.13.40
You J, Frazer H, Sayyar S, et al., 2022, Development of an in situ printing system with human platelet lysate-based bio-adhesive to treat corneal perforations. Transl Vis Sci Technol, 11(6): 26. https://doi.org/10.1167/tvst.11.6.26
Albanna M, Binder KW, Murphy SV, et al., 2019, In situ bioprinting of autologous skin cells accelerates wound healing of extensive excisional full-thickness wounds. Sci Rep, 9(1): 1856. https://doi.org/10.1038/s41598-018-38366-w
Cubo N, Garcia M, Del Cañizo JF, et al., 2017, 3D bioprinting of functional human skin: Production and in vivo analysis. Biofabrication, 9: 1–12. https://doi.org/10.1088/1758-5090/9/1/015006
Cheng RY, Eylert G, Gariepy J-M, et al., 2021, Handheld instrument for wound-conformal delivery of skin precursor sheets improves healing in full-thickness burns. Biofabrication, 12(2): 025002. https://doi.org/10.1088/1758-5090/ab6413
Del Amo C, Perez-Valle A, Perez-Garrastachu M, et al., 2021, Plasma-based bioinks for extrusion bioprinting of advanced dressings. Biomedicines, 9: 1023. https://doi.org/10.3390/biomedicines9081023
Diaz-Gomez L, Gonzalez-Prada I, Millan R, et al., 2022, 3D printed carboxymethyl cellulose scaffolds for autologous growth factors delivery in wound healing. Carbohydr Polym, 278: 118924. https://doi.org/10.1016/J.CARBPOL.2021.118924
Taymour R, Kilian D, Ahlfeld T, et al., 2021, 3D bioprinting of hepatocytes: Core-shell structured co-cultures with fibroblasts for enhanced functionality. Sci Rep, 11: 5130. https://doi.org/10.1038/S41598-021-84384-6
de Melo BAG, Cruz EM, Ribeiro TN, et al., 2021, 3D bioprinting of murine cortical astrocytes for engineering neural-like tissue. J Vis Exp., 173: e62691 https://doi.org/10.3791/62691
England S, Rajaram A, Schreyer DJ, et al., 2017, Bioprinted fibrin-factor XIII-hyaluronate hydrogel scaffolds with encapsulated Schwann cells and their in vitro characterization for use in nerve regeneration. Bioprinting, 5: 1–9. https://doi.org/10.1016/J.BPRINT.2016.12.001
Tao J, Liu H, Wu W, et al., 2020, 3D-printed nerve conduits with live platelets for effective peripheral nerve repair. Adv Funct Mater, 30: 2004272. https://doi.org/10.1002/ADFM.202004272
Han J, Kim DS, Jang H, et al., 2019, Bioprinting of three-dimensional dentin–pulp complex with local differentiation of human dental pulp stem cells. J Tissue Eng, 10: 1–10. https://doi.org/10.1177/2041731419845849
Yi K, Li Q, Lian X, et al., 2022, Utilizing 3D bioprinted platelet-rich fibrin-based materials to promote the regeneration of oral soft tissue. Regen Biomater, 9: rbac021. https://doi.org/10.1093/rb/rbac021
Duin S, Bhandarkar S, Lehmann S, et al., 2022, Viability and functionality of neonatal porcine islet-like cell clusters bioprinted in alginate-based bioinks. Biomedicines, 10: 1420. https://doi.org/10.3390/biomedicines10061420
Dani S, Ahlfeld T, Albrecht F, et al., 2021, Homogeneous and reproducible mixing of highly viscous biomaterial inks and cell suspensions to create bioinks. Gels, 7: 227. https://doi.org/10.3390/GELS7040227
Mendes BB, Gómez-Florit M, Hamilton AG, et al., 2020, Human platelet lysate-based nanocomposite bioink for bioprinting hierarchical fibrillar structures. Biofabrication, 12: 015012. https://doi.org/10.1088/1758-5090/ab33e8
Min SJ, Lee JS, Nah H, et al., 2021, Development of photo-crosslinkable platelet lysate-based hydrogels for 3D printing and tissue engineering. Biofabrication, 13: 044102. https://doi.org/10.1088/1758-5090/AC1993
Somasekharan LT, Kasoju N, Raju R, et al., 2020, Formulation and characterization of alginate dialdehyde, gelatin, and platelet-rich plasma-based bioink for bioprinting applications. Bioengineering, 7: 1–12. https://doi.org/10.3390/bioengineering7030108
Kirsch M, Birnstein L, Pepelanova I, et al., 2019, Gelatin-methacryloyl (GelMA) formulated with human platelet lysate supports mesenchymal stem cell proliferation and differentiation and enhances the hydrogel’s mechanical properties. Bioeng, 6: 76. https://doi.org/10.3390/BIOENGINEERING6030076
Wendel JS, Ye L, Tao R, et al., 2015, Functional effects of a tissue-engineered cardiac patch from human induced pluripotent stem cell-derived cardiomyocytes in a rat infarct model. Stem Cells Transl Med, 4: 1324–1332. https://doi.org/10.5966/SCTM.2015-0044
Roura S, Soler-Botija C, Bagó JR, et al., 2015, Postinfarction functional recovery driven by a three-dimensional engineered fibrin patch composed of human umbilical cord blood-derived mesenchymal stem cells. Stem Cells Transl Med, 4: 956–966. https://doi.org/10.5966/SCTM.2014-0259/-/DC1
Bellamy V, Vanneaux V, Bel A, et al., 2015, Long-term functional benefits of human embryonic stem cell-derived cardiac progenitors embedded into a fibrin scaffold. J Hear Lung Transplant, 34: 1198–1207. https://doi.org/10.1016/j.healun.2014.10.008