Hybrid solid mesh structure for electron beam melting customized implant to treat bone cancer
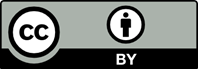
Bone replacement implants manufactured by electron beam melting have been widely studied for use in bone tumor treatment. In this application, a hybrid structure implant with a combination of solid and lattice structures guarantees strong adhesion between bone and soft tissues. This hybrid implant must exhibit adequate mechanical performance so as to satisfy the safety criteria considering repeated weight loading during the patient’s lifetime. With a low volume of a clinical case, various shape and volume combinations, including both solid and lattice structures, should be evaluated to provide guidelines for implant design. This study examined the mechanical performance of the hybrid lattice by investigating two shapes of the hybrid implant and volume fractions of the solid and lattice structures, along with microstructural, mechanical, and computational analyses. These results demonstrate how hybrid implants may be designed to improve clinical outcomes by using patientspecific orthopedic implants with optimized volume fraction of the lattice structure, allowing for effective enhancement of mechanical performance as well as optimized design for bone cell ingrowth.
1. Mankin H, Gebhardt M, Jennings L, et al., 1996, Long-term results of allograft replacement in the management of bone tumors. Relat Res, 324:86–97.
2. Wunder J, Leitch K, Griffin A, et al., 2001, Comparison of two methods of reconstruction for primary malignant tumors at the knee: A sequential cohort study. J Surg Oncol, 77:89–99. https://doi.org/10.1002/jso.1076
3. Jeon DG, Kim MS, Cho WH, et al., 2007, Pasteurized autograft for intercalary reconstruction. Clin Orthop Relat Res, 456:203–210. https://doi.org/10.1097/BLO.0b013e31802e7ec8
4. Jeon DG, Song WS, Cho WH, et al., 2014, Overlapping allograft for primary or salvage bone tumor reconstruction. J Surg Oncol, 110:366–371. https://doi.org/10.1002/jso.23669
5. Shusteff M, Browar AEM, Kelly EW, et al., 2017, One-step volumetric additive manufacturing of complex polymer structures. Sci Adv, 3:eaao5496. https://doi.org/10.1126/sciadv.aao5496
6. Zhang D, Qiu D, Gibson MA, et al., 2019, Additive manufacturing of ultrafine-grained high-strength titanium alloys. Nature, 576:91–95. https://doi.org/10.1038/s41586-019-1783-1
7. Jung ID, Choe J, Yun J, et al., 2019, Dual speed laser re-melting for high densification in H13 tool steel metal 3D printing. Arch Metall Mater, 64(2):571–578. https://doi.org/10.24425/amm.2021.136388
8. Sing SL, 2022, Perspectives on additive manufacturing enabled beta-titanium alloys for biomedical applications. Int J Bioprint, 8(1):478. http://dx.doi.org/10.18063/ijb.v8i1.478
9. Lee MS, Kim H, Koo YT, et al., 2022, Selective laser melting process for sensor embedding into SUS316L with heat dissipative inner cavity design. Met Mater Int, 28:297–305. https://doi.org/10.1007/s12540-021-01106-3
10. Wong KC, Kumta SM, Geel NV, et al., 2015, One-step reconstruction with a 3D-printed, biomechanically evaluated custom implant after complex pelvic tumor resection. Aided Surg, 20:14–23. https://doi.org/10.3109/10929088.2015.1076039
11. Liang H, Ji T, Zhang Y, et al., 2017, Reconstruction with 3D-printed pelvic endoprostheses after resection of a pelvic tumour. Bone Jt J, 99-B:267–275. https://doi.org/10.1302/0301-620X.99B2.BJJ-2016-0654.R1
12. Wei R, Guo W, Ji T, et al., 2017, One-step reconstruction with a 3D-printed, custom-made prosthesis after total en bloc sacrectomy: A technical note. Eur Spine J, 26:1902–1909. https://doi.org/10.1007/s00586-016-4871-z
13. Park JW, Kang HG, Lim KM, et al., 2018, Three-dimensionally printed personalized implant design and reconstructive surgery for a bone tumor of the calcaneus. JBJS Case Connect, 8:e25. https://doi.org/10.2106/JBJS.CC.17.00212
14. Park JW, Kang HG, Lim KM, et al., 2018, Bone tumor resection guide using three-dimensional printing for limb salvage surgery. J Surg Oncol, 118:898–905. https://doi.org/10.1002/jso.25236
15. Angelini A, Trovarelli G, Berizzi A, et al., 2019, Three-dimension-printed custom-made prosthetic reconstructions: From revision surgery to oncologic reconstructions. Int Orthop, 43:123–132. https://doi.org/10.1007/s00264-018-4232-0
16. Park JW, Kang HG, Kim JH, et al., 2020, New 3-dimensional implant application as an alternative to allograft in limb salvage surgery: A technical note on 10 cases. Acta Orthop, 91:489–496. https://doi.org/10.1080/17453674.2020.1755543
17. Eleutério SJP, Senerchia AA, Almeida, et al., 2015, Osteosarcoma in patients younger than 12 years old without metastases have similar prognosis as adolescent and young adults. Pediatr Blood Cancer, 62:1209–1213. https://doi.org/10.1002/pbc.25459
18. Kim Y, Jang WY, Park JW, et al., 2020, Transepiphyseal resection for osteosarcoma in patients with open physes using MRI assessment. Bone Jt J, 102-B:772–778. https://doi.org/10.1302/0301-620X.102B6.BJJ-2019-1141.R2
19. Park JW, Kang HG, Kim JH, et al., 2021, The application of 3D-printing technology in pelvic bone tumor surgery. J Orthop Sci, 26(2):276–283. https://doi.org/10.1016/j.jos.2020.03.004
20. Mumith A, Coathup M, Chimutengwende-Gordon M, et al., 2017, Augmenting the osseointegration of endoprostheses using laser-sintered porous collars. Bone Jt J, 99-B:276–282. https://doi.org/10.1302/0301-620X.99B2.BJJ-2016-0584.R1
21. McGilvray KC, Easley J, Seim HB, et al., 2018, Bony ingrowth potential of 3D-printed porous titanium alloy: A direct comparison of interbody cage materials in an in vivo ovine lumbar fusion model. Spine J, 18(7):1250–1260. https://doi.org/10.1016/j.spinee.2018.02.018
22. Wu SH, Li Y, Zhang YQ, et al., 2013, Porous titanium-6 aluminum-4 vanadium cage has better osseointegration and less micromotion than a poly-ether-ether-ketone cage in sheep vertebral fusion. Artif Organs, 37:E191–E201. https://doi.org/10.1111/aor.12153
23. Li JP, Habibovic P, Van Den Doel M, et al., 2007, Bone ingrowth in porous titanium implants produced by 3D fiber deposition. Biomaterials, 28:2810–2820. https://doi.org/10.1016/j.biomaterials.2007.02.020
24. De Wild M, Zimmermann S, Rüegg J, et al., 2016, Influence of microarchitecture on osteoconduction and mechanics of porous titanium scaffolds generated by selective laser melting. 3D Print Addit Manuf, 3(3):142–151. https://doi.org/10.1089/3dp.2016.0004
25. Park JW, Song CA, Kang HG, et al., 2020, Integration of a three-dimensional-printed titanium implant in human tissues: Case study. Appl Sci, 10:553. https://doi.org/10.3390/app10020553
26. Park JW, Shin YC, Kang HG, et al., 2021, In vivo analysis of post-joint-preserving surgery fracture of 3D-printed Ti-6Al-4V implant to treat bone cancer. Bio-des Manuf, 4:879–888. https://doi.org/10.1007/s42242-021-00147-2
27. Limmahakhun S, Oloyede A, Sitthiseripratip K, et al., 2017, Stiffness and strength tailoring of cobalt chromium graded cellular structures for stress-shielding reduction. Mater Des, 114:633–641. https://doi.org/10.1016/j.matdes.2016.11.090
28. Mahmoud D, Elbestawi MA, 2019, Selective laser melting of porosity graded lattice structures for bone implants. Int J Adv Manuf, 100:2915–2927. https://doi.org/10.1007/s00170-018-2886-9
29. Leong KF, Chua SC, Sudarmadji N, et al., 2008, Engineering functionally graded tissue engineering scaffolds. J Mech Behav Biomed Mater, 1(2):140–152. https://doi.org/10.1016/j.jmbbm.2007.11.002
30. Fousová M, Vojtěch D, Kubásek J, et al., 2017, Promising characteristics of gradient porosity Ti-6Al-4V alloy prepared by SLM process. Mech Behav Biomed Mater, 69: 368–376. https://doi.org/10.1016/j.jmbbm.2017.01.043
31. Sing SL, An J, Yeong WY, et al., 2016, Laser and electron-beam powder-bed additive manufacturing of metallic implants: A review on processes, materials and designs. J Orthop Res, 34:369–385. https://doi.org/10.1002/jor.23075