Development and characterization of an automated active mixing platform for hydrogel bioink preparation
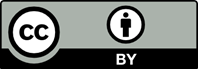
Bioink preparation is an important yet challenging step for bioprinting with hydrogels, as it involves fast and homogeneous mixing of various viscous components. In this study, we have developed an automated active mixing platform (AAMP), which allows for high-quality preparation of hydrogel bioinks. The design of AAMP, adapted from syringe pumps, provides many advantages, including low cost, automated control, high precision, customizability, and great cytocompatibility, as well as the potential to intelligently detect the homogeneity. To demonstrate the capability of AAMP, mixing of different hydrogel components, including alginate and xanthan gum with and without Ca2+, alginate and Laponite, PEGDMA and xanthan gum, was performed to investigate an alginate hydrogel preparation process. Colorimetric analyses were carried out to evaluate the mixing outcome with AAMP. Result showed that AAMP can prepare homogeneous hydrogel mixing in a fast and automated fashion. A multiphysics COMSOL simulation is carried out to further validate the results. Moreover, cell viability and proliferation study were performed in a cell encapsulation mixing experiment to validate the cytocompatibility of the AAMP. The AAMP has demonstrated great capability in hydrogel bioink preparation and could therefore holds great promise and wide applications in bioprinting and tissue engineering.
1. Aswathy SH, Narendrakumar U, Manjubala I, 2020, Commercial hydrogels for biomedical applications. Heliyon, 6: e03719. https://doi.org/10.1016/j.heliyon.2020.e03719
2. Billiet T, Vandenhaute M, Schelfhout J, et al., 2012, A review of trends and limitations in hydrogel-rapid prototyping for tissue engineering. Biomaterials, 33: 6020–6041. https://doi.org/10.1016/j.biomaterials.2012.04.050
3. Khademhosseini A, Langer R, 2007, Microengineered hydrogels for tissue engineering. Biomaterials, 28: 5087–5092. https://doi.org/10.1016/j.biomaterials.2007.07.021
4. Zhu J, Marchant RE, 2011, Design properties of hydrogel tissue-engineering scaffolds. Expert Rev Med Devices, 8: 607–626. https://doi.org/10.1586/erd.11.27
5. Ji S, Guvendiren M, 2017, Recent advances in bioink design for 3D bioprinting of tissues and organs. Front Bioeng Biotechnol, 5: 23. https://doi.org/10.3389/fbioe.2017.00023
6. Jia J, Richards DJ, Pollard S, et al., 2014, Engineering alginate as bioink for bioprinting. Acta Biomater, 10: 4323–4331. https://doi.org/10.1016/j.actbio.2014.06.034
7. Kesti M, Muller M, Becher J, et al., 2015, A versatile bioink for three-dimensional printing of cellular scaffolds based on thermally and photo-triggered tandem gelation. Acta Biomater, 11: 162–172. https://doi.org/10.1016/j.actbio.2014.09.033
8. Kumar H, Sakthivel K, Mohamed MG, et al., 2021, Designing gelatin methacryloyl (GelMA)-based bioinks for visible light stereolithographic 3D biofabrication. Macromol Biosci, 21: e2000317. https://doi.org/10.1002/mabi.202000317
9. Li N, Guo R, Zhang ZJ, 2021, Bioink formulations for bone tissue regeneration. Front Bioeng Biotechnol, 9: 630488. https://doi.org/10.3389/fbioe.2021.630488
10. Naranda J, Bracic M, Vogrin M, et al., 2021, Recent advancements in 3D printing of polysaccharide hydrogels in cartilage tissue engineering. Materials (Basel), 14: 3977. https://doi.org/10.3390/ma14143977
11. Possl A, Hartzke D, Schmidts TM, et al., 2021, A targeted rheological bioink development guideline and its systematic correlation with printing behavior. Biofabrication, 13: 035021. https://doi.org/10.1088/1758-5090/abde1e
12. Unagolla JM, Jayasuriya AC, 2020, Hydrogel-based 3D bioprinting: A comprehensive review on cell-laden hydrogels, bioink formulations, and future perspectives. Appl Mater Today, 18: 100479. https://doi.org/10.1016/j.apmt.2019.100479
13. Yin J, Yan M, Wang Y, et al., 2018, 3D bioprinting of low-concentration cell-laden gelatin methacrylate (GelMA) bioinks with a two-step cross-linking strategy. ACS Appl Mater Interfaces, 10: 6849–6857. https://doi.org/10.1021/acsami.7b16059
14. Zheng Z, Wu J, Liu M, et al., 2018, 3D bioprinting of self-standing silk-based bioink. Adv Healthc Mater, 7: e1701026. https://doi.org/10.1002/adhm.201701026
15. Puertas-Bartolome M, Wlodarczyk-Biegun MK, Del Campo A, et al., 2020, 3D printing of a reactive hydrogel bio-ink using a static mixing tool. Polymers (Basel), 12: 1986. https://doi.org/10.3390/polym12091986
16. Tamayol A, Najafabadi AH, Aliakbarian B, et al., 2015, Hydrogel templates for rapid manufacturing of bioactive fibers and 3D constructs. Adv Healthc Mater, 4: 2146–2153. https://doi.org/10.1002/adhm.201500492
17. Li Y, Yang HY, Lee DS, 2021, Advances in biodegradable and injectable hydrogels for biomedical applications. J Control Release, 330: 151–160. https://doi.org/10.1016/j.jconrel.2020.12.008
18. Ma T, Lv L, Ouyang C, et al., 2021, Rheological behavior and particle alignment of cellulose nanocrystal and its composite hydrogels during 3D printing. Carbohydr Polyme, 253: 117217. https://doi.org/10.1016/j.carbpol.2020.117217
19. Zhang W, Ma X, Li Y, et al., 2020, Preparation of smooth and macroporous hydrogel via hand-held blender for wound healing applications: In-vitro and in-vivo evaluations. Biomed Mater, 15: 055032. https://doi.org/10.1088/1748-605X/ab9d6f
20. Mulakkal MC, Trask RS, Ting VP, et al., 2018, Responsive cellulose-hydrogel composite ink for 4D printing. Mater Des, 160: 108–118. https://doi.org/10.1016/j.matdes.2018.09.009
21. Fu YC, Chen CH, Wang CZ, et al., 2013, Preparation of porous bioceramics using reverse thermo-responsive hydrogels in combination with rhBMP-2 carriers: In vitro and in vivo evaluation. J Mech Behav Biomed Mater, 27: 64–76. https://doi.org/10.1016/j.jmbbm.2013.06.009
22. Müller M, Öztürk E, Arlov Ø, et al., 2017, Alginate sulfate-nanocellulose bioinks for cartilage bioprinting applications. Ann Biomed Eng, 45: 210–223. https://doi.org/10.1007/s10439-016-1704-5
23. Apelgren P, Amoroso M, Säljö K, et al., 2018, Skin grafting on 3D bioprinted cartilage constructs in vivo. Plast Reconstr Surg Glob Open, 6: e1930. https://doi.org/10.1097/GOX.0000000000001930
24. Apelgren P, Amoroso M, Lindahl A, et al., 2017, Chondrocytes and stem cells in 3D-bioprinted structures create human cartilage in vivo. PLoS One, 12: e0189428. https://doi.org/10.1371/journal.pone.0189428
25. Cohen DL, Lo W, Tsavaris A, et al., 2011, Increased mixing improves hydrogel homogeneity and quality of three-dimensional printed constructs. Tissue Eng Part C Methods, 17: 239–248. https://doi.org/10.1089/ten.TEC.2010.0093
26. Lowe SB, Tan VT, Soeriyadi AH, et al., 2014, Synthesis and high-throughput processing of polymeric hydrogels for 3D cell culture. Bioconjug Chem, 25: 1581–1601. https://doi.org/10.1021/bc500310v
27. Rabanel JM, Hildgen P, 2004, Preparation of hydrogel hollow particles for cell encapsulation by a method of polyester core degradation. J Microencapsul, 21: 413–431. https://doi.org/10.1080/02652040410001729223
28. Billiet T, Gevaert E, De Schryver T, et al., 2014, The 3D printing of gelatin methacrylamide cell-laden tissue-engineered constructs with high cell viability. Biomaterials, 35: 49–62. https://doi.org/10.1016/j.biomaterials.2013.09.078
29. Wang LH, Ernst AU, An D, et al., 2021, A bioinspired scaffold for rapid oxygenation of cell encapsulation systems. Nat Commun, 12: 5846. https://doi.org/10.1038/s41467-021-26126-w
30. Touani FK, Borie M, Azzi F, et al., 2021, Pharmacological preconditioning improves the viability and proangiogenic paracrine function of hydrogel-encapsulated mesenchymal stromal cells. Stem Cells Int, 2021: 6663467. https://doi.org/10.1155/2021/6663467
31. Pan Z, Bui L, Yadav V, et al., 2021, Conformal single cell hydrogel coating with electrically induced tip streaming of an AC cone. Biomater Sci, 9: 3284–3292. https://doi.org/10.1039/d0bm02100h
32. Karimi S, Bagher Z, Najmoddin N, et al., 2021, Alginate-magnetic short nanofibers 3D composite hydrogel enhances the encapsulated human olfactory mucosa stem cells bioactivity for potential nerve regeneration application. Int J Biol Macromol, 167: 796–806. https://doi.org/10.1016/j.ijbiomac.2020.11.199
33. Hwang DG, Jo Y, Kim M, et al., 2021, A 3D bioprinted hybrid encapsulation system for delivery of human pluripotent stem cell-derived pancreatic islet-like aggregates. Biofabrication, 14: 014101. https://doi.org/10.1088/1758-5090/ac23ac
34. Ghasemi A, Akbari E, Imani R, 2021, An overview of engineered hydrogel-based biomaterials for improved β-cell survival and insulin secretion. Front Bioeng Biotechnol, 9: 662084. https://doi.org/10.3389/fbioe.2021.662084
35. Bonani W, Cagol N, Maniglio D, 2020, Alginate hydrogels: A tool for 3D cell encapsulation, tissue engineering, and biofabrication. Adv Exp Med Biol, 1250: 49–61. https://doi.org/10.1007/978-981-15-3262-7_4
36. Garcia-Ochoa F, Santos VE, Casas JA, et al., 2000, Xanthan gum: Production, recovery, and properties. Biotechnol Adv, 18: 549–579. https://doi.org/10.1016/s0734-9750(00)00050-1
37. Riaz T, Iqbal MW, Jiang B, et al., 2021, A review of the enzymatic, physical, and chemical modification techniques of xanthan gum. Int J Biol Macromol, 186: 472–489. https://doi.org/10.1016/j.ijbiomac.2021.06.196
38. Wang L, Li W, Qin S, 2021, Three polymers from the sea: unique structures, directional modifications, and medical applications. Polymers (Basel), 13: 2482. https://doi.org/10.3390/polym13152482
39. Van Hoorick J, Tytgat L, Dobos A, et al., 2019, (Photo-) crosslinkable gelatin derivatives for biofabrication applications. Acta Biomater, 97: 46–73. https://doi.org/10.1016/j.actbio.2019.07.035
40. Chen H, Fei F, Li X, et al., 2021, A structure-supporting, self-healing, and high permeating hydrogel bioink for establishment of diverse homogeneous tissue-like constructs. Bioact Mater, 6: 3580–3595. https://doi.org/10.1016/j.bioactmat.2021.03.019
41. Marques CF, Diogo GS, Pina S, et al., 2019, Collagen-based bioinks for hard tissue engineering applications: A comprehensive review. J Mater Sci Mater Med, 30: 32. https://doi.org/10.1007/s10856-019-6234-x
42. Rajabi N, Rezaei A, Kharaziha M, et al., 2021, Recent advances on bioprinted gelatin methacrylate-based hydrogels for tissue repair. Tissue Eng Part A, 27: 679–702. https://doi.org/10.1089/ten.TEA.2020.0350
43. Magalhaes LS, Santos FE, de Maria Vaz Elias C, et al., 2020, Printing 3D hydrogel structures employing low-cost stereolithography technology. J Funct Biomater, 11: 12. https://doi.org/10.3390/jfb11010012
44. Acosta-Velez GF, Zhu TZ, Linsley CS, et al., 2018, Photocurable poly(ethylene glycol) as a bioink for the inkjet 3D pharming of hydrophobic drugs. Int J Pharm, 546: 145–153. https://doi.org/10.1016/j.ijpharm.2018.04.056