Biomimetic three-dimensional glioma model printed in vitro for the studies of glioma cells and neurons interactions
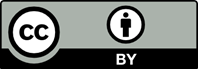
The interactions between glioma cells and neurons are important for glioma progression but are rarely mimicked and recapitulated in in vitro three-dimensional (3D) models, which may affect the success rate of relevant drug research and development. In this study, an in vitro bioprinted 3D glioma model consisting of an outer hemispherical shell with neurons and an inner hemisphere with glioma cells is proposed to simulate the natural glioma. This model was produced by extrusion-based 3D bioprinting technology. The cells survival rate, morphology, and intercellular Ca2+ concentration studies were carried out up to 5 days of culturing. It was found that neurons could promote the proliferation of glioma cells around them, associate the morphological changes of glioma cells to be neuron-like, and increase the expression of intracellular Ca2+ of glioma cells. Conversely, the presence of glioma cells could maintain the neuronal survival rate and promote the neurite outgrowth. The results indicated that glioma cells and neurons facilitated each other implying a symbiotic pattern established between two types of cells during the early stage of glioma development, which were seldom found in the present artificial glioma models. The proposed bioprinted glioma model can mimic the natural microenvironment of glioma tissue, provide an in-depth understanding of cell–cell interactions, and enable pathological and pharmacological studies of glioma.
1. Li Z, Li M, Xia P, et al., 2022, Targeting long non-coding RNA PVT1/TGF-β/Smad by p53 prevents glioma progression. Cancer Biol Ther, 23(1):225–233.
2. Weller M, Cloughesy T, Perry JR, et al., 2013, Standards of care for treatment of recurrent glioblastoma—Are we there yet? Neuro-Oncology, 15(1):4–27.
3. Bobustuc GC, Baker CH, Limaye A, et al., 2010, Levetiracetam enhances p53-mediated MGMT inhibition and sensitizes glioblastoma cells to temozolomide. Neuro- Oncology, 12(9):917–927.
4. Chen Y, Henson ES, Xiao W, et al., 2016, Bcl-2 family member Mcl-1 expression is reduced under hypoxia by the E3 ligase FBW7 contributing to BNIP3 induced cell death in glioma cells. Cancer Biol Ther, 17(6):604–613.
5. Wang C, Tong X, Yang F, 2014, Bioengineered 3D brain tumor model to elucidate the effects of matrix stiffness on glioblastoma cell behavior using PEG-based hydrogels. Mol Pharm, 11(7):2115–2125.
6. Xu X, Li L, Luo L, et al., 2021, Opportunities and challenges of glioma organoids. Cell Commun Signal, 19(1):1–13.
7. Zhang C, Jin M, Zhao J, et al., 2020, Organoid models of glioblastoma: Advances, applications and challenges. Am J Cancer Res, 10(8):2242–2257.
8. De Witt Hamer PC, Van Tilborg AAG, Eijk PP, et al., 2008, The genomic profile of human malignant glioma is altered early in primary cell culture and preserved in spheroids. Oncogene, 27(14):2091–2096.
9. Wang X, Prager BC, Wu Q, et al., 2018, Reciprocal signaling between glioblastoma stem cells and differentiated tumor cells promotes malignant progression. Cell Stem Cell, 22(4):514–528.
10. Goers L, Freemont P, Polizzi KM, 2014, Co-culture systems and technologies: Taking synthetic biology to the next level. J R Soc Interface, 11(96):20140065.
11. Singh SK, Hawkins C, Clarke ID, et al., 2004, Identification of human brain tumour initiating cells. Nature, 432(7015): 396–401.
12. Yuan X, Curtin J, Xiong Y, et al., 2004, Isolation of cancer stem cells from adult glioblastoma multiforme. Oncogene, 23(58):9392–9400.
13. Mirab F, Kang YJ, Majd S, 2019, Preparation and characterization of size-controlled glioma spheroids using agarose hydrogel microwells. PLoS One, 14(1): e0211078.
14. Barresi V, Belluardo N, Sipione S, et al., 2003, Transplantation of prodrug-converting neural progenitor cells for brain tumor therapy. Cancer Gene Ther, 10(5):396–402.
15. Liu Y, Carlsson R, Ambjørn M, et al., 2013, PD-L1 expression by neurons nearby tumors indicates better prognosis in glioblastoma patients. J Neurosci, 33(35):14231–14245.
16. Gillespie S, Monje M, 2018, An active role for neurons in glioma progression: Making sense of Scherer’s structures. Neuro-Oncology, 20(10):1292–1299.
17. Scherer HJ, 1938, Structural development in gliomas. Am J Cancer, 34(3):333–351.
18. Venkatesh HS, Morishita W, Geraghty AC, et al., 2019, Electrical and synaptic integration of glioma into neural circuits. Nature, 573(7775):539–545.
19. Fu YS, Lin YY, Chou SC, et al., 2008, Tetramethylpyrazine inhibits activities of glioma cells and glutamate neuro-excitotoxicity: Potential therapeutic application for treatment of gliomas. Neuro-Oncology, 10(2):139–152.
20. Chaunsali L, Tewari BP, Gallucci A, et al., 2020, Glioma-induced peritumoral hyperexcitability in a pediatric glioma model. Physiol Rep, 8(19):e14567.
21. Wei Z, Kale S, El Fatimy R, et al., 2019, Co-cultures of glioma stem cells and primary neurons, astrocytes, microglia, and endothelial cells for investigation of intercellular communication in the brain. Front Neurosci-Switz, 13:361.
22. Fuchs Q, Batut A, Gleyzes M, et al., 2021, Co-culture of glutamatergic neurons and pediatric high-grade glioma cells into microfluidic devices to assess electrical interactions. JoVE, 16(177): e62748.
23. Saunders RE, Derby B, 2014, Inkjet printing biomaterials for tissue engineering: Bioprinting. Int Mater Rev, 59(8): 430–448.
24. Schiele NR, Corr DT, Huang Y, et al., 2010, Laser-based direct-write techniques for cell printing. Biofabrication, 2(3):032001.
25. Gu Z, Fu J, Lin H, et al., 2020, Development of 3D bioprinting: From printing methods to biomedical applications. Asian J Pharm Sci, 15(5):529–557.
26. Ji S, Guvendiren M, 2021, Complex 3D bioprinting methods. APL Bioeng, 5(1):011508.
27. Choudhury D, Tun HW, Wang T, et al., 2018, Organ-derived decellularized extracellular matrix: A game changer for bioink manufacturing? Trends Biotechnol, 36(8):787–805.
28. Sorushanova A, Delgado LM, Wu Z, et al., 2019, The collagen suprafamily: From biosynthesis to advanced biomaterial development. Adv Mater, 31(1):1801651.
29. Di Nunno V, Franceschi E, Tosoni A, et al., 2022, Tumor-associated microenvironment of adult gliomas: A review. Front Oncol, 12:891543.
30. Gómez-Oliva R, Domínguez-García S, Carrascal L, et al., 2021, Evolution of experimental models in the study of glioblastoma: Toward finding efficient treatments. Front Oncol, 10:614295.
31. Wang X, Li X, Ding J, et al., 2021, 3D bioprinted glioma microenvironment for glioma vascularization. J Biomed Mater Res Part A, 109(6):915–925.
32. Cha J, Kim P, 2017, Biomimetic strategies for the glioblastoma microenvironment. Front Mater, 4:45.
33. Zhao J, He H, Zhou K, et al., 2012, Neuronal transcription factors induce conversion of human glioma cells to neurons and inhibit tumorigenesis. PLoS One, 7(7):41506–41517.
34. Su Z, Zang T, Liu ML, et al., 2014, Reprogramming the fate of human glioma cells to impede brain tumor development. Cell Death Discov, 5(10): e1463–e1463.
35. Fu JQ, Chen Z, Hu YJ, et al., 2019, A single factor induces neuronal differentiation to suppress glioma cell growth. CNS Neurosci Ther, 25(4):486–495.
36. Yan T, Skaftnesmo KO, Leiss L, et al., 2011, Neuronal markers are expressed in human gliomas and NSE knockdown sensitizes glioblastoma cells to radiotherapy and temozolomide. BMC Cancer, 11(1):1–11.
37. Li F, Zhang W, Wang M, et al., 2020, IL1RAP regulated by PRPRD promotes gliomas progression via inducing neuronal synapse development and neuron differentiation in vitro. Pathol Res Pract, 216(11):153141.
38. Nagaraja S, Vitanza NA, Woo PJ, et al., 2017, Transcriptional dependencies in diffuse intrinsic pontine glioma. Cancer Cell, 31(5):635–652.
39. Lawn S, Krishna N, Pisklakova A, et al., 2015, Neurotrophin signaling via TrkB and TrkC receptors promotes the growth of brain tumor-initiating cells. J Biol Chem, 290(6):3814– 3824.
40. Aryal M, Vykhodtseva N, Zhang YZ, et al., 2013, Multiple treatments with liposomal doxorubicin and ultrasound-induced disruption of blood–tumor and blood–brain barriers improve outcomes in a rat glioma model. J Controlled Release, 169(1–2):103–111.
41. Buckingham SC, Campbell SL, Haas BR, et al., 2011, Glutamate release by primary brain tumors induces epileptic activity. Nat Med, 17(10):1269–1274.
42. Campbell SL, Buckingham SC, Sontheimer H, 2012, Human glioma cells induce hyperexcitability in cortical networks. Epilepsia, 53(8):1360–1370.
43. Johung T, Monje M, 2017, Neuronal activity in the glioma microenvironment. Curr Opin Neurobiol, 47:156–161.
44. Pei Z, Lee KC, Khan A, et al., 2020, Pathway analysis of glutamate-mediated, calcium-related signaling in glioma progression. Biochem Pharmacol, 176:113814.
45. Leclerc C, Haeich J, Aulestia FJ, et al., 2016, Calcium signaling orchestrates glioblastoma development: Facts and conjunctures. Biochim Biophys Acta Mol Cell Res, 1863(6):1447–1459.
46. Zündorf G, Reiser G, 2011, Calcium dysregulation and homeostasis of neural calcium in the molecular mechanisms neuroprotection. Antioxid Redox Signal, 14(7):1275–1288.
47. Prevarskaya N, Skryma R, Shuba Y, 2011, Calcium in tumour metastasis: New roles for known actors. Nat Rev Cancer, 11(8):609–618.
48. Marchi S, Pinton P, 2016, Alterations of calcium homeostasis in cancer cells. Curr Opin Pharmacol, 29:1–6.
49. Déliot N, Constantin B, 2015, Plasma membrane calcium channels in cancer: Alterations and consequences for cell proliferation and migration. Biochim Biophys Acta Biomembr, 1848(10):2512–2522.
50. Lang F, Stournaras C, 2014, Ion channels in cancer: future perspectives and clinical potential. Philos Trans R Soc B, 369(1638):20130108.
51. Chen WL, Turlova E, Sun CLF, et al., 2015, Xyloketal B suppresses glioblastoma cell proliferation and migration in vitro through inhibiting TRPM7-regulated PI3K/Akt and MEK/ERK signaling pathways. Mar Drugs, 13(4): 2505–2525.
52. Morrone FB, Gehring MP, Nicoletti NF, 2016, Calcium channels and associated receptors in malignant brain tumor therapy. Mol Pharmacol, 90(3):403–409.
53. Wee S, Niklasson M, Marinescu VD, et al., 2014, Selective calcium sensitivity in immature glioma cancer stem cells. PLoS One, 9(12):e115698.
54. Berridge MJ, Bootman MD, Roderick HL, 2003, Calcium signalling: Dynamics, homeostasis and remodelling. Nat Rev Mol Cell Biol, 4(7):517–529.
55. Wiegert JS, Bading H, 2011, Activity-dependent calcium signaling and ERK-MAP kinases in neurons: A link to structural plasticity of the nucleus and gene transcription regulation. Cell Calcium, 49(5):296–305.
56. Danbolt NC, 2001, Glutamate uptake. Prog Neurobiol, 65(1):1–105.
57. Barria A, 2019, Dangerous liaisons as tumour cells form synapses with neurons. Nature, 573:499–501.
58. Parpura V, Grubišić V, Verkhratsky A, 2011, Ca2+ sources for the exocytotic release of glutamate from astrocytes. Biochim Biophys Acta Mol Cell Res, 1813(5):984–991.
59. Malarkey EB, Parpura V, 2008, Mechanisms of glutamate release from astrocytes. Neurochem Int, 52(1–2):142–154.
60. Metea MR, Newman EA, 2006, Calcium signaling in specialized glial cells. Glia, 54(7):650–655.
61. Ye ZC, Sontheimer H, 1999, Glioma cells release excitotoxic concentrations of glutamate. Cancer Res, 59(17):4383–4391.
62. Sontheimer H, 2008, A role for glutamate in growth and invasion of primary brain tumors. J Neurochem, 105(2): 287–295.
63. Maklad A, Sharma A, Azimi I, 2019, Calcium signaling in brain cancers: Roles and therapeutic targeting. Cancers, 11(2):145.
64. Wypych D, Pomorski P, 2013, Calcium signaling in glioma cells—The role of nucleotide receptors. Glioma Signal, 986:61–79.
65. Lin C, Chen J, Su Z, et al., 2021, A calcium-related immune signature in prognosis prediction of patients with glioma. Front Cell Dev Biol, 9:2740.
66. Vannini E, Olimpico F, Middei S, et al., 2016, Electrophysiology of glioma: A Rho GTPase-activating protein reduces tumor growth and spares neuron structure and function. Neuro Oncol, 18(12):1634–1643.
67. Sprugnoli G, Golby AJ, Santarnecchi E, 2021, Newly discovered neuron-to-glioma communication: New noninvasive therapeutic opportunities on the horizon? Neurooncol Adv, 3(1): vdab018.
68. Zhang Y, Sloan SA, Clarke LE, et al., 2016, Purification and characterization of progenitor and mature human astrocytes reveals transcriptional and functional differences with mouse. Neuron, 89(1):37–53.