3D-bioprinted human lipoaspirate-derived cellladen skin constructs for healing of full-thickness skin defects
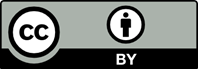
Three-dimensional (3D)-printed bioactive scaffolds that can be produced rapidly could offer an individualized approach for treating full-thickness skin defects. Decellularized extracellular matrix (dECM) and mesenchymal stem cells have been proven to support wound healing. Adipose tissues obtained by liposuction are rich in adipose-derived dECM (adECM) and adipose-derived stem cells (ADSCs) and thus represent a natural source of bioactive materials for 3D bioprinting. Herein, ADSC-laden 3D-printed bioactive scaffolds consisting of gelatin methacryloyl (GelMA), hyaluronic acid methacryloyl (HAMA), and adECM were fabricated with dual properties of photocrosslinking in vitro and thermosensitive crosslinking in vivo. adECM was prepared by decellularization of human lipoaspirate and mixed as a bioactive material with GelMA and HAMA to form a bioink. Compared with the GelMA–HAMA bioink, the adECM–GelMA–HAMA bioink had better wettability, degradability, and cytocompatibility. Full-thickness skin defect healing in a nude mouse model showed that ADSC-laden adECM–GelMA–HAMA scaffolds accelerated wound healing by promoting faster neovascularization, collagen secretion, and remodeling. ADSCs and adECM collectively conferred bioactivity on the prepared bioink. This study represents a novel approach to enhancing the biological activity of 3D-bioprinted skin substitutes by adding adECM and ADSCs derived from human lipoaspirate and may provide a promising therapeutic option for full-thickness skin defects.
1. Fu X, 2020, Wound healing center establishment and new technology application in improving the wound healing quality in China. Burns Trauma, 8:tkaa038. https://doi.org/10.1093/burnst/tkaa038
2. Nussbaum SR, Carter MJ, Fife CE, et al., 2018, An economic evaluation of the impact, cost, and Medicare policy implications of chronic nonhealing wounds. Value Health, 21(1):27–32. https://doi.org/10.1016/j.jval.2017.07.007
3. Wurzer P, Keil H, Branski LK, et al., 2016, The use of skin substitutes and burn care—A survey. J Surg Res, 201(2): 293–298. https://doi.org/10.1016/j.jss.2015.10.048
4. Riha SM, Maarof M, Fauzi MB, 2021, Synergistic effect of biomaterial and stem cell for skin tissue engineering in cutaneous wound healing: A concise review. Polymers (Basel), 13(10):1546. https://doi.org/10.3390/polym13101546
5. Stone RC, Stojadinovic O, Rosa AM, et al., 2017, A bioengineered living cell construct activates an acute wound healing response in venous leg ulcers. Sci Transl Med, 9(371):eaaf8611. https://doi.org/10.1126/scitranslmed.aaf8611
6. Eudy M, Eudy CL, Roy S, 2021, Apligraf as an alternative to skin grafting in the pediatric population. Cureus, 13(7):e16226. https://doi.org/10.7759/cureus.16226
7. Dai C, Shih S, Khachemoune A, 2020, Skin substitutes for acute and chronic wound healing: An updated review. J Dermatol Treat, 31(6):639–648. https://doi.org/10.1080/09546634.2018.1530443
8. Niu C, Wang L, Ji D, et al., 2022, Fabrication of SA/Gel/C scaffold with 3D bioprinting to generate micro-nano porosity structure for skin wound healing: A detailed animal in vivo study. Cell Regen, 11(1):10. https://doi.org/10.1186/s13619-022-00113-y
9. Pontiggia L, Van Hengel IA, Klar A, et al., 2022, Bioprinting and plastic compression of large pigmented and vascularized human dermo-epidermal skin substitutes by means of a new robotic platform. J Tissue Eng, 13:20417314221088513. https://doi.org/10.1177/20417314221088513
10. Michael S, Sorg H, Peck CT, et al., 2013, Tissue engineered skin substitutes created by laser-assisted bioprinting form skin-like structures in the dorsal skin fold chamber in mice. PLoS One, 8(3):e57741. https://doi.org/10.1371/journal.pone.0057741
11. Ng WL, Qi JTZ, Yeong WY, et al., 2018, Proof-of-concept: 3D bioprinting of pigmented human skin constructs. Biofabrication, 10(2):025005. https://doi.org/10.1088/1758-5090/aa9e1e
12. Zhang Y, Enhejirigala, Yao B, et al., 2021, Using bioprinting and spheroid culture to create a skin model with sweat glands and hair follicles. Burns Trauma, 9:tkab013. https://doi.org/10.1093/burnst/tkab013
13. Albanna M, Binder KW, Murphy SV, et al., 2019, In situ bioprinting of autologous skin cells accelerates wound healing of extensive excisional full-thickness wounds. Sci Rep, 9(1):1856. https://doi.org/10.1038/s41598-018-38366-w
14. Jorgensen AM, Varkey M, Gorkun A, et al., 2020, Bioprinted skin recapitulates normal collagen remodeling in full-thickness wounds. Tissue Eng Part A, 26(9-10):512–526. https://doi.org/10.1089/ten.TEA.2019.0319
15. He P, Zhao J, Zhang J, et al., 2018, Bioprinting of skin constructs for wound healing. Burns Trauma, 6:5. https://doi.org/10.1186/s41038-017-0104-x
16. Raziyeva K, Kim Y, Zharkinbekov Z, et al., 2021, Immunology of acute and chronic wound healing . Biomolecules, 11(5):700. https://doi.org/10.3390/biom11050700
17. Rodrigues M, Kosaric N, Bonham CA, et al., 2019, Wound healing: A cellular perspective. Physiol Rev, 99(1):665–706. https://doi.org/10.1152/physrev.00067.2017
18. Shaik S, Wu X, Gimble JM, et al., 2020, Non-toxic freezing media to retain the stem cell reserves in adipose tissues. Cryobiology, 96:137–144. https://doi.org/10.1016/j.cryobiol.2020.07.005
19. Chaudhuri O, Cooper-White J, Janmey PA, et al., 2020, Effects of extracellular matrix viscoelasticity on cellular behaviour. Nature, 584(7822):535–546. https://doi.org/10.1038/s41586-020-2612-2
20. Frantz C, Stewart KM, Weaver VM, 2010, The extracellular matrix at a glance. J Cell Sci, 123(Pt 24):4195–4200. https://doi.org/10.1242/jcs.023820
21. Ventura RD, Padalhin AR, Park CM, et al., 2019, Enhanced decellularization technique of porcine dermal ECM for tissue engineering applications. Mater Sci Eng C Mater Biol Appl, 104:109841. https://doi.org/10.1016/j.msec.2019.109841
22. Saldin LT, Cramer MC, Velankar SS, et al., 2017, Extracellular matrix hydrogels from decellularized tissues: Structure and function. Acta Biomater. 49:1–15. https://doi.org/10.1016/j.actbio.2016.11.068
23. Panwar A, Tan LP, 2016, Current status of bioinks for micro-extrusion-based 3D bioprinting. Molecules, 21(6):685. https://doi.org/10.3390/molecules21060685
24. Yue K, Trujillo-de Santiago G, Alvarez MM, et al., 2015, Synthesis, properties, and biomedical applications of gelatin methacryloyl (GelMA) hydrogels. Biomaterials, 73:254–271. https://doi.org/10.1016/j.biomaterials.2015.08.045
25. Zhai P, Peng X, Li B, et al., 2020, The application of hyaluronic acid in bone regeneration. Int J Biol Macromol, 151:1224–1239. https://doi.org/10.1016/j.ijbiomac.2019.10.169
26. Dung TN, Han VD, Tien GN, et al., 2021, Autologous adipose-derived stem cell (ADSC) transplantation in the management of chronic wounds. Ann Burns Fire Disasters, 34(4):343–350.
27. Tanios E, Ahmed TM, Shafik EA, et al., 2021, Efficacy of adipose-derived stromal vascular fraction cells in the management of chronic ulcers: A randomized clinical trial. Regen Med, 16(11):975–988. https://doi.org/10.2217/rme-2020-0207
28. Zhang J, Liu Y, Chen Y, et al., 2020, Adipose-derived stem cells: Current applications and future directions in the regeneration of multiple tissues. Stem Cells Int, 2020:8810813. https://doi.org/10.1155/2020/8810813
29. Sivan U, Jayakumar K, Krishnan LK, 2014, Constitution of fibrin-based niche for in vitro differentiation of adipose-derived mesenchymal stem cells to keratinocytes. Biores Open Access, 3(6):339–347. https://doi.org/10.1089/biores.2014.0036
30. Hutchings G, Janowicz K, Moncrieff L, et al., 2020, The proliferation and differentiation of adipose-derived stem cells in neovascularization and angiogenesis. Int J Mol Sci, 21(11):3790. https://doi.org/10.3390/ijms21113790
31. Zhou ZQ, Chen Y, Chai M, et al., 2019, Adipose extracellular matrix promotes skin wound healing by inducing the differentiation of adipose derived stem cells into fibroblasts. Int J Mol Med, 43(2):890–900. https://doi.org/10.3892/ijmm.2018.4006
32. Lee DE, Ayoub N, Agrawal DK, 2016, Mesenchymal stem cells and cutaneous wound healing: Novel methods to increase cell delivery and therapeutic efficacy. Stem Cell Res Ther, 7:37. https://doi.org/10.1186/s13287-016-0303-6
33. Roshangar L, Rad JS, Kheirjou R, et al., 2021, Using 3D-bioprinting scaffold loaded with adipose-derived stem cells to burns wound healing. J Tissue Eng Regen Med, 15(6):546–555. https://doi.org/10.1002/term.3194
34. Wu Y, Liang T, Hu Y, et al., 2021, 3D bioprinting of integral ADSCs-NO hydrogel scaffolds to promote severe burn wound healing. Regen Biomater, 8(3):rbab014. https://doi.org/10.1093/rb/rbab014
35. Flynn LE, 2010, The use of decellularized adipose tissue to provide an inductive microenvironment for the adipogenic differentiation of human adipose-derived stem cells. Biomaterials, 31(17):4715–4724. https://doi.org/10.1016/j.biomaterials.2010.02.046
36. Crapo PM, Gilbert TW, Badylak SF, 2011, An overview of tissue and whole organ decellularization processes. Biomaterials, 32(12):3233–3243. https://doi.org/10.1016/j.biomaterials.2011.01.057
37. Aamodt JM, Grainger DW, 2016, Extracellular matrix-based biomaterial scaffolds and the host response. Biomaterials, 86:68–82. https://doi.org/10.1016/j.biomaterials.2016.02.003
38. Pati F, Jang J, Ha DH, et al., 2014, Printing three-dimensional tissue analogues with decellularized extracellular matrix bioink. Nat Commun, 5:3935. https://doi.org/10.1038/ncomms4935
39. Paxton N, Smolan W, Bock T, et al., 2017, Proposal to assess printability of bioinks for extrusion-based bioprinting and evaluation of rheological properties governing bioprintability. Biofabrication, 9(4):044107. https://doi.org/10.1088/1758-5090/aa8dd8
40. Holzl K, Lin S, Tytgat L, et al., 2016, Bioink properties before, during and after 3D bioprinting. Biofabrication, 8(3):032002. https://doi.org/10.1088/1758-5090/8/3/032002
41. Somasekharan LT, Kasoju N, Raju R, et al., 2020, Formulation and characterization of alginate dialdehyde, gelatin, and platelet-rich plasma-based bioink for bioprinting applications. Bioengineering (Basel), 7(3):108. https://doi.org/10.3390/bioengineering7030108
42. Aboomeirah AA, Sarhan WA, Khalil EA, et al., 2022, Wet electrospun nanofibers-fortified gelatin/alginate-based nanocomposite as a single-dose biomimicking skin substitute. ACS Appl Bio Mater, 5(8):3678–3694. https://doi.org/10.1021/acsabm.2c00147
43. Lutzweiler G, Barthes J, Koenig G, et al., 2019, Modulation of cellular colonization of porous polyurethane scaffolds via the control of pore interconnection size and nanoscale surface modifications. ACS Appl Mater Interfaces, 11(22):19819– 19829. https://doi.org/10.1021/acsami.9b04625
44. Ng WL, Lee JM, Zhou M, et al., 2020, Vat polymerization-based bioprinting-process, materials, applications and regulatory challenges. Biofabrication, 12(2):022001. https://doi.org/10.1088/1758-5090/ab6034
45. Ng WL, Huang X, Shkolnikov V, et al., 2022, Controlling droplet impact velocity and droplet volume: Key factors to achieving high cell viability in sub-nanoliter droplet-based bioprinting. Int J Bioprint, 8(1):424. https://doi.org/10.18063/ijb.v8i1.424
46. Jiang T, Munguia-Lopez JG, Flores-Torres S, et al., 2019, Extrusion bioprinting of soft materials: An emerging technique for biological model fabrication. Appl Phys Rev, 6:011310. https://doi.org/10.1063/1.5059393
47. Barros NR, Kim HJ, Gouidie MJ, et al., 2021, Biofabrication of endothelial cell, dermal fibroblast, and multilayered keratinocyte layers for skin tissue engineering. Biofabrication, 13(3). https://doi.org/10.1088/1758-5090/aba503
48. Almalki SG, 2022, Adipose-derived mesenchymal stem cells and wound healing: Potential clinical applications in wound repair. Saudi Med J, 43(10):1075–1086. https://doi.org/10.15537/smj.2022.43.10.20220522
49. Zhou L, Wang H, Yao S, et al., 2022, Efficacy of human adipose derived mesenchymal stem cells in promoting skin wound healing. J Healthc Eng, 2022:6590025. https://doi.org/10.1155/2022/6590025
50. Huayllani MT, Sarabia-Estrada R, Restrepo DJ, et al., 2020, Adipose-derived stem cells in wound healing of full-thickness skin defects: A review of the literature. J Plast Surg Hand Surg, 54(5):263–279. https://doi.org/10.1080/2000656X.2020.1767116
51. Wang N, Liu H, Li X, et al., 2017, Activities of MSCs derived from transgenic mice seeded on ADM scaffolds in wound healing and assessment by advanced optical techniques. Cell Physiol Biochem, 42(2):623–639. https://doi.org/10.1159/000477872
52. Rousselle P, Montmasson M, Garnier C, 2019, Extracellular matrix contribution to skin wound re-epithelialization. Matrix Biol, 75–76:12–26. https://doi.org/10.1016/j.matbio.2018.01.002
53. Li ZJ, Wang LQ, Li YZ, et al., 2021, Application of adipose-derived stem cells in treating fibrosis. World J Stem Cells, 13(11):1747–1761. https://doi.org/10.4252/wjsc.v13.i11.1747
54. Ibañez RIR, do Amaral RJFC, Reis RL, et al., 2021, 3D-printed gelatin methacrylate scaffolds with controlled architecture and stiffness modulate the fibroblast phenotype towards dermal regeneration. Polymers (Basel), 13(15):2510. https://doi.org/10.3390/polym13152510
55. Pati F, Ha D-H, Jang J, et al., 2015, Biomimetic 3D tissue printing for soft tissue regeneration. Biomaterials, 62: 164–175. https://doi.org/10.1016/j.biomaterials.2015.05.043
56. Baron JM, Glatz M, Proksch E, 2020, Optimal support of wound healing: New insights. Dermatology, 236(6):593–600. https://doi.org/10.1159/000505291
57. Leavitt T, Hu MS, Marshall CD, et al., 2016, Scarless wound healing: Finding the right cells and signals. Cell Tissue Res, 365(3):483–493. https://doi.org/10.1007/s00441-016-2424-8
58. Sorg H, Tilkorn DJ, Mirastschijski U, et al., 2018, Panta rhei: Neovascularization, angiogenesis and nutritive perfusion in wound healing. Eur Surg Res, 59(3–4):232–241. https://doi.org/10.1159/000492410
59. DiPietro LA, 2016, Angiogenesis and wound repair: When enough is enough. J Leukoc Biol, 100(5):979–984. https://doi.org/10.1189/jlb.4MR0316-102R
60. Diller RB, Tabor AJ, 2022, The role of the extracellular matrix (ECM) in wound healing: A review. Biomimetics (Basel), 7(3):87. https://doi.org/10.3390/biomimetics7030087
61. Moffat D, Ye K, Jin S, 2022, Decellularization for the retention of tissue niches. J Tissue Eng, 13:20417314221101151. https://doi.org/10.1177/20417314221101151
62. Guilak F, Cohen DM, Estes BT, et al., 2009, Control of stem cell fate by physical interactions with the extracellular matrix. Cell Stem Cell, 5(1):17–26. https://doi.org/10.1016/j.stem.2009.06.016
63. Xu Y, Deng M, Cai Y, et al., 2020, Cell-free fat extract increases dermal thickness by enhancing angiogenesis and extracellular matrix production in nude mice. Aesthet Surg J, 40(8):904–913. https://doi.org/10.1093/asj/sjz306
64. Mazini L, Rochette L, Admou B, et al., 2020, Hopes and limits of adipose-derived stem cells (ADSCs) and mesenchymal stem cells (MSCs) in wound healing. Int J Mol Sci, 21(4):1306. https://doi.org/10.3390/ijms21041306
65. Zhang P, Zhang C, Li J, et al., 2019, The physical microenvironment of hematopoietic stem cells and its emerging roles in engineering applications. Stem Cell Res Ther, 10(1):327. https://doi.org/10.1186/s13287-019-1422-7
66. Zhou X, Wang J, Huang X, et al., 2018, Injectable decellularized nucleus pulposus-based cell delivery system for differentiation of adipose-derived stem cells and nucleus pulposus regeneration. Acta Biomater, 81:115–128. https://doi.org/10.1016/j.actbio.2018.09.044
67. Xiao Y, Peng J, Liu Q, et al., 2020, Ultrasmall CuS@ BSA nanoparticles with mild photothermal conversion synergistically induce MSCs-differentiated fibroblast and improve skin regeneration. Theranostics, 10(4):1500–1513. https://doi.org/10.7150/thno.39471
68. An R, Zhang Y, Qiao Y, et al., 2020, Adipose stem cells isolated from diabetic mice improve cutaneous wound healing in streptozotocin-induced diabetic mice. Stem Cell Res Ther, 11(1):120. https://doi.org/10.1186/s13287-020-01621-x
69. Liubaviciute A, Kaseta V, Vaitkuviene A, et al., 2018, Regenerative potential of partially differentiated mesenchymal stromal cells in a mouse model of a full-thickness skin wound. Excl J, 17:871–888. https://doi.org/10.17179/excli2018-1504
70. Dos Santos JF, Borcari NR, Silva Araujo, et al., 2019, Mesenchymal stem cells differentiate into keratinocytes and express epidermal kallikreins: Towards an in vitro model of human epidermis. J Cell Biochem, 120(8):13141–13155. https://doi.org/10.1002/jcb.28589