3D-printed vascularized biofunctional scaffold for bone regeneration
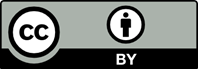
3D-printed biofunctional scaffolds have promising applications in bone tissue regeneration. However, the development of bioinks with rapid internal vascularization capabilities and relatively sustained osteoinductive bioactivity is the primary technical challenge. In this work, we added rat platelet-rich plasma (PRP) to a methacrylated gelatin (GelMA)/methacrylated alginate (AlgMA) system, which was further modified by a nanoclay, laponite (Lap). We found that Lap was effective in retarding the release of multiple growth factors from the PRP-GelMA/AlgMA (PRP-GA) hydrogel and sustained the release for up to 2 weeks. Our in vitro studies showed that the PRP-GA@Lap hydrogel significantly promoted the proliferation, migration, and osteogenic differentiation of rat bone marrow mesenchymal stem cells, accelerated the formation of endothelial cell vascular patterns, and promoted macrophage M2 polarization. Furthermore, we printed hydrogel bioink with polycaprolactone (PCL) layer-by-layer to form active bone repair scaffolds and implanted them in subcutaneous and femoral condyle defects in rats. In vivo experiments showed that the PRP-GA@Lap/PCL scaffolds significantly promoted vascular inward growth and enhanced bone regeneration at the defect site. This work suggests that PRP-based 3D-bioprinted vascularized scaffolds will have great potential for clinical translation in the treatment of bone defects.
1. Armiento AR, Hatt LP, Rosenberg GS, et al., 2020, Functional biomaterials for bone regeneration: A lesson in complex biology. Adv Funct Mater, 30(44):1909874. https://doi.org/10.1002/adfm.201909874
2. Zhang YB, Liu XC, Zeng LD, et al., 2019, Polymer fiber scaffolds for bone and cartilage tissue engineering. Adv Funct Mater, 29(36):1903279. https://doi.org/10.1002/adfm.201903279
3. Zheng CC, Attarilar S, Li K, et al., 2021, 3D-printed HA15- loaded beta-tricalcium phosphate/poly (lactic-co-glycolic acid) bone tissue scaffold promotes bone regeneration in rabbit radial defects. Int J Bioprint, 7(1):100–111. https://doi.org/10.18063/ijb.v7i1.317
4. Li Y, Xie KG, Wang C, et al., 2021, 3D printing of tricalcium phosphate/poly lactic-co-glycolic acid. Scaffolds loaded with carfilzomib for treating critical-sized rabbit radial bone defects. Int J Bioprint, 7(4):99–111. https://doi.org/10.18063/ijb.v7i4.405
5. Lin ZF, Wu MM, He HM, et al., 2019, 3D printing of mechanically stable calcium-free alginate-based scaffolds with tunable surface charge to enable cell adhesion and facile biofunctionalization. Adv Funct Mater, 29(9):1808439. https://doi.org/10.1002/adfm.201808439
6. Groll J, Burdick JA, Cho DW, et al., 2019, A definition of bioinks and their distinction from biomaterial inks. Biofabrication, 11(1):013001. https://doi.org/10.1088/1758-5090/aaec52
7. Zhang S, Chen J, Yu Y, et al., 2019, Accelerated bone regenerative efficiency by regulating sequential release of BMP-2 and VEGF and synergism with sulfated chitosan. ACS Biomater Sci Eng, 5(4):1944–1955. https://doi.org/10.1021/acsbiomaterials.8b01490
8. Kempen DH, Lu L, Heijink A, et al., 2009, Effect of local sequential VEGF and BMP-2 delivery on ectopic and orthotopic bone regeneration. Biomaterials, 30(14): 2816–2825. https://doi.org/10.1016/j.biomaterials.2009.01.031
9. Geuze RE, Theyse LF, Kempen DH, et al., 2012, A differential effect of bone morphogenetic protein-2 and vascular endothelial growth factor release timing on osteogenesis at ectopic and orthotopic sites in a large-animal model. Tissue Eng Part A, 18(19-20):2052–2062. https://doi.org/10.1089/ten.TEA.2011.0560
10. Wei S, Xu P, Yao Z, et al., 2021, A composite hydrogel with co-delivery of antimicrobial peptides and platelet-rich plasma to enhance healing of infected wounds in diabetes. Acta Biomater, 124:205–218. https://doi.org/10.1016/j.actbio.2021.01.046
11. Singh M, Nanda HS, Lee JYH, et al., 2020, Photocurable platelet rich plasma bioadhesives. Acta Biomater, 117: 133–141. https://doi.org/10.1016/j.actbio.2020.09.030
12. Iyer SR, Scheiber AL, Yarowsky P, et al., 2020, Exosomes isolated from platelet-rich plasma and mesenchymal stem cells promote recovery of function after muscle injury. Am J Sports Med, 48(9):2277–2286. https://doi.org/10.1177/0363546520926462
13. Uludag H, D’Augusta D, Palmer R, et al., 1999, Characterization of rhBMP-2 pharmacokinetics implanted with biomaterial carriers in the rat ectopic model. J Biomed Mater Res, 46(2):193–202. https://doi.org/10.1002/(sici)1097-4636(199908)46: 2<193::aid-jbm8>3.0.co;2-1
14. Bouletreau PJ, Warren SM, Spector JA, et al., 2002, Hypoxia and VEGF up-regulate BMP-2 mRNA and protein expression in microvascular endothelial cells: Implications for fracture healing. Plast Reconstr Surg, 109(7):2384–2397. https://doi.org/10.1097/00006534-200206000-00033
15. Pufe T, Wildemann B, Petersen W, et al., 2002, Quantitative measurement of the splice variants 120 and 164 of the angiogenic peptide vascular endothelial growth factor in the time flow of fracture healing: A study in the rat. Cell Tissue Res, 309(3):387–392. https://doi.org/10.1007/s00441-002-0605-0
16. Uchida S, Sakai A, Kudo H, et al., 2003, Vascular endothelial growth factor is expressed along with its receptors during the healing process of bone and bone marrow after drill-hole injury in rats. Bone, 32(5):491–501. https://doi.org/10.1016/s8756-3282(03)00053-x
17. Schär MO, Diaz-Romero J, Kohl S, et al., 2015, Platelet-rich concentrates differentially release growth factors and induce cell migration in vitro. Clin Orthop Relat Res, 473(5): 1635–1643. https://doi.org/10.1007/s11999-015-4192-2
18. Dohan Ehrenfest DM, Rasmusson L, Albrektsson T, 2009, Classification of platelet concentrates: From pure platelet-rich plasma (P-PRP) to leucocyte- and platelet-rich fibrin (L-PRF). Trends Biotechnol, 27(3):158–167. https://doi.org/10.1016/j.tibtech.2008.11.009
19. Chuah YJ, Peck Y, Lau JE, et al., 2017, Hydrogel based cartilaginous tissue regeneration: Recent insights and technologies. Biomater Sci, 5(4):613–631. https://doi.org/10.1039/c6bm00863a
20. Yue K, Trujillo-de Santiago G, Alvarez MM, et al., 2015, Synthesis, properties, and biomedical applications of gelatin methacryloyl (GelMA) hydrogels. Biomaterials, 73:254–271. https://doi.org/10.1016/j.biomaterials.2015.08.045
21. Chu C, Deng J, Sun X, et al., 2017, Collagen membrane and immune response in guided bone regeneration: Recent progress and perspectives. Tissue Eng Part B Rev, 23(5):421–435. https://doi.org/10.1089/ten.TEB.2016.0463
22. Niu X, Ferracci G, Lin M, et al., 2021, Highly substituted decoupled gelatin methacrylamide free of hydrolabile methacrylate impurities: An optimum choice for long-term stability and cytocompatibility. Int J Biol Macromol, 167:479–490. https://doi.org/10.1016/j.ijbiomac.2020.11.187
23. Krishna KV, Ménard-Moyon C, Verma S, et al., 2013, Graphene-based nanomaterials for nanobiotechnology and biomedical applications. Nanomedicine (Lond), 8(10):1669– 1688.
https://doi.org/10.2217/nnm.13.140
24. Waters R, Pacelli S, Maloney R, et al., 2016, Stem cell secretome-rich nanoclay hydrogel: A dual action therapy for cardiovascular regeneration. Nanoscale, 8(14):7371–7376. https://doi.org/10.1039/c5nr07806g
25. Ding X, Gao J, Wang Z, et al., 2016, A shear-thinning hydrogel that extends in vivo bioactivity of FGF2. Biomaterials, 111:80–89. https://doi.org/10.1016/j.biomaterials.2016.09.026
26. Zhang Y, Xu J, Ruan YC, et al., 2016, Implant-derived magnesium induces local neuronal production of CGRP to improve bone-fracture healing in rats. Nat Med, 22(10):1160–1169. https://doi.org/10.1038/nm.4162
27. Lee CS, Hwang HS, Kim S, et al., 2020, Inspired by nature: Facile design of nanoclay-organic hydrogel bone sealant with multifunctional properties for robust bone regeneration. Adv Funct Mater, 30(43):2003717. https://doi.org/10.1002/adfm.202003717
28. Cao BJ, Wang XW, Zhu L, et al., 2019, MicroRNA-146a sponge therapy suppresses neointimal formation in rat vein grafts. IUBMB Life, 71(1):125–133. https://doi.org/10.1002/iub.1946
29. Cao BJ, Wang XW, Zhu L, et al., 2019, Dedicator of cytokinesis 2 silencing therapy inhibits neointima formation and improves blood flow in rat vein grafts. J Mol Cell Cardiol, 128:134–144. https://doi.org/10.1016/j.yjmcc.2019.01.030
30. Liu X, Yang Y, Niu X, et al., 2017, An in situ photocrosslinkable platelet rich plasma—Complexed hydrogel glue with growth factor controlled release ability to promote cartilage defect repair. Acta Biomater, 62:179–187. https://doi.org/10.1016/j.actbio.2017.05.023
31. Daskalakis E, Liu FY, Huang BY, et al., 2021, Investigating the influence of architecture and material composition of 3D printed anatomical design scaffolds for large bone defects. Int J Bioprint, 7(2):43–52. https://doi.org/10.18063/ijb.v7i2.268
32. Shao H, Liu A, Ke X, et al., 2017, 3D robocasting magnesium-doped wollastonite/TCP bioceramic scaffolds with improved bone regeneration capacity in critical sized calvarial defects. J Mater Chem B, 5(16):2941–2951. https://doi.org/10.1039/c7tb00217c
33. Matai I, Kaur G, Seyedsalehi A, et al., 2020, Progress in 3D bioprinting technology for tissue/organ regenerative engineering. Biomaterials, 226:119536. https://doi.org/10.1016/j.biomaterials.2019.119536
34. Epstein NE, 2013, Complications due to the use of BMP/ INFUSE in spine surgery: The evidence continues to mount. Surg Neurol Int, 4(Suppl 5):S343–S352. https://doi.org/10.4103/2152-7806.114813
35. Shields LB, Raque GH, Glassman SD, et al., 2006, Adverse effects associated with high-dose recombinant human bone morphogenetic protein-2 use in anterior cervical spine fusion. Spine (Phila Pa 1976), 31(5):542–547. https://doi.org/10.1097/01.brs.0000201424.27509.72
36. Lee K, Silva EA, Mooney DJ, 2011, Growth factor delivery-based tissue engineering: General approaches and a review of recent developments. J R Soc Interface, 8(55):153–170. https://doi.org/10.1098/rsif.2010.0223
37. Liu KT, Zhao M, Li Y, et al., 2022, VEGF loaded porcine decellularized adipose tissue derived hydrogel could enhance angiogenesis in vitro and in vivo. J Biomater Sci Poly Ed, 33(5):569–589. https://doi.org/10.1080/09205063.2021.2002235
38. Jiang GY, Li SH, Yu K, et al., 2021, A 3D-printed PRP-GelMA hydrogel promotes osteochondral regeneration through M2 macrophage polarization in a rabbit model. Acta Biomater, 128:150–162. https://doi.org/10.1016/j.actbio.2021.04.010
39. Zhao M, Wang J, Zhang JX, et al., 2022, Functionalizing multi-component bioink with platelet-rich plasma for customized in-situ bilayer bioprinting for wound healing. Mater Today Bio, 16:100334. https://doi.org/10.1016/j.mtbio.2022.100334
40. Gaharwar AK, Mihaila SM, Swami A, et al., 2013, Bioactive silicate nanoplatelets for osteogenic differentiation of human mesenchymal stem cells. Adv Mater, 25(24):3329–3336. https://doi.org/10.1002/adma.201300584
41. Stegen S, van Gastel N, Carmeliet G, 2015, Bringing new life to damaged bone: The importance of angiogenesis in bone repair and regeneration. Bone, 70:19–27. https://doi.org/10.1016/j.bone.2014.09.017
42. Klopfleisch R, Jung F, 2017, The pathology of the foreign body reaction against biomaterials. J Biomed Mater Res A, 105(3):927–940. https://doi.org/10.1002/jbm.a.35958
43. Brown BN, Badylak SF, 2013, Expanded applications, shifting paradigms and an improved understanding of host-biomaterial interactions. Acta Biomater, 9(2):4948–4955. https://doi.org/10.1016/j.actbio.2012.10.025
44. Papait A, Cancedda R, Mastrogiacomo M, et al., 2018, Allogeneic platelet-rich plasma affects monocyte differentiation to dendritic cells causing an anti-inflammatory microenvironment, putatively fostering wound healing. J Tissue Eng Regen Med, 12(1):30–43. https://doi.org/10.1002/term.2361