Commercial articulated collaborative in situ 3D bioprinter for skin wound healing
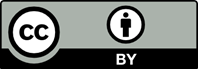
In situ bioprinting is one of the most clinically relevant techniques in the emerging bioprinting technology because it could be performed directly on the human body in the operating room and it does not require bioreactors for post-printing tissue maturation. However, commercial in situ bioprinters are still not available on the market. In this study, we demonstrated the benefit of the originally developed first commercial articulated collaborative in situ bioprinter for the treatment of full-thickness wounds in rat and porcine models. We used an articulated and collaborative robotic arm from company KUKA and developed original printhead and correspondence software enabling in situ bioprinting on curve and moving surfaces. The results of in vitro and in vivo experiments show that in situ bioprinting of bioink induces a strong hydrogel adhesion and enables printing on curved surfaces of wet tissues with a high level of fidelity. The in situ bioprinter was convenient to use in the operating room. Additional in vitro experiments (in vitro collagen contraction assay and in vitro 3D angiogenesis assay) and histological analyses demonstrated that in situ bioprinting improves the quality of wound healing in rat and porcine skin wounds. The absence of interference with the normal process of wound healing and even certain improvement in the dynamics of this process strongly suggests that in situ bioprinting could be used as a novel therapeutic modality in wound healing.
1. Murphy SV, Atala A, 2014, 3D bioprinting of tissues and organs. Nat Biotechnol, 32: 773–785. https://doi.org/10.1038/nbt.2958
2. Sun W, Starly B, Daly AC, et al., 2020, The bioprinting roadmap. Biofabrication, 12: 022002. https://doi.org/10.1088/1758-5090/ab5158
3. Murphy SV, De Coppi P, Atala A, 2020, Opportunities and challenges of translational 3D bioprinting. Nat Biomed Eng, 4: 370–380. https://doi.org/10.1038/s41551-019-0471-7
4. Ozbolat IT, 2015, Bioprinting scale-up tissue and organ constructs for transplantation. Trends Biotechnol, 33: 395–400. https://doi.org/10.1016/j.tibtech.2015.04.005
5. Mironov V, Kasyanov V, Drake C, et al., 2008, Organ printing: Promises and challenges. Regen Med, 3: 93–103. https://doi.org/10.2217/17460751.3.1.93
6. He J, Mao M, Li X, et al., 2021, Bioprinting of 3D functional tissue constructs. Int J Bioprint, 7: 395. https://doi.org/10.18063/ijb.v7i3.395
7. Mironov V, Boland T, Trusk T, et al., 2003, Organ printing: Computer-aided jet-based 3D tissue engineering. Trends Biotechnol, 1: 157–161. https://doi.org/10.1016/S0167-7799(03)00033-7
8. Zhang J, Wehrle E, Rubert M, et al., 2021, 3D bioprinting of human tissues: Biofabrication, bioinks, and bioreactors. Int J Mol Sci, 22: 3971. https://doi.org/10.3390/ijms22083971
9. Yeong WY, Chua CK, Leong KF, et al., 2004, Rapid prototyping in tissue engineering: Challenges and potential. Trends Biotechnol, 22: 643–652. https://doi.org/10.1016/j.tibtech.2004.10.004
10. Ng WL, Chua CK, Shen YF, 2019, Print me an organ! Why we are not there yet. Prog Polym Sci, 97: 101145. https://doi.org/10.1016/j.progpolymsci.2019.101145
11. Weng T, Zhang W, Xia Y, et al., 2021, 3D bioprinting for skin tissue engineering: Current status and perspectives. J Tissue Eng, 12: 20417314211028574. https://doi.org/10.1177/20417314211028574
12. Gao C, Lu C, Jian Z, et al., 2021, 3D bioprinting for fabricating artificial skin tissue. Colloids Surf B Biointerfaces, 208: 112041. https://doi.org/10.1016/j.colsurfb.2021.112041
13. Martin I, Wendt D, Heberer M, 2004, The role of bioreactors in tissue engineering. Trends Biotechnol, 22: 80–6. https://doi.org/10.1016/j.tibtech.2003.12.001
14. Hansmann J, Groeber F, Kahlig A, et al., 2013, Bioreactors in tissue engineering-principles, applications and commercial constraints. Biotechnol J, 8: 298–307. https://doi.org/10.1002/biot.201200162
15. Martin I, Smith T, Wendt D, 2009, Bioreactor-based roadmap for the translation of tissue engineering strategies into clinical products. Trends Biotechnol, 27: 495–502. https://doi.org/10.1016/j.tibtech.2009.06.002
16. Singh S, Choudhury D, Yu F, et al., 2019, In situ bioprinting-bioprinting from benchside to bedside? Acta Biomater, 101: 14–25. https://doi.org/10.1016/j.actbio.2019.08.045
17. Samandari M, Mostafavi A, Quint J, et al., 2022, In situ bioprinting: Intraoperative implementation of regenerative medicine. Trends Biotechnol, 40: 1229–1247. https://doi.org/10.1016/j.tibtech.2022.03.009
18. Dias JR, Ribeiro N, Baptista-Silva S, et al., 2020, In situ enabling approaches for tissue regeneration: Current challenges and new developments. Front Bioeng Biotechnol, 8: 85. https://doi.org/10.3389/fbioe.2020.00085
19. Ashammakhi N, Ahadian S, Pountos I, et al., 2019, In situ three-dimensional printing for reparative and regenerative therapy. Biomed Microdevices, 21: 42. https://doi.org/10.1007/s10544-019-0372-2
20. Wu Y, Ravnic DJ, Ozbolat IT, 2020, Intraoperative bioprinting: repairing tissues and organs in a surgical setting. Trends Biotechnol, 38: 594–605. https://doi.org/10.1016/j.tibtech.2020.01.004
21. Neng X, Guohong S, Yuling S, et al., 2020, Research progress of robot technology in in situ 3D bioprinting. Int J Bioprint, 8: 614. https://doi.org/10.18063/ijb.v8i4.614
22. Choudhury D, Anand S, Naing MW, 2018, The arrival of commercial bioprinters-towards 3D bioprinting revolution! Int J Bioprint, 4: 139. https://doi.org/10.18063/IJB.v4i2.139
23. Wang M, He J, Liu Y, et al., 2015, The trend towards in vivo bioprinting. Int J Bioprint, 1: 15–26. https://doi.org/10.18063/IJB.2015.01.001
24. Ding H, Chang RC, 2018, Simulating image-guided in situ bioprinting of a skin graft onto a phantom burn wound bed. Addit Manuf, 22: 708–719. https://doi.org/10.1016/j.addma.2018.06.022
25. Fortunato GM, Rossi G, Bonatti AF, et al., 2021, Robotic platform and path planning algorithm for in situ bioprinting. Bioprinting, 22: e00139. https://doi.org/10.1016/j.bprint.2021.e00139
26. Di Bella C, Duchi S, O’Connell CD, et al., 2018, In situ handheld three-dimensional bioprinting for cartilage regeneration. J Tissue Eng Regen Med, 12: 611–621. https://doi.org/10.1002/term.2476
27. Hakimi N, Cheng R, Leng L, et al., 2018, Handheld skin printer: In situ formation of planar biomaterials and tissues. Lab Chip, 18: 1440–1451. https://doi.org/10.1039/c7lc01236e
28. Albanna M, Binder KW, Murphy SV, et al., 2019, In situ bioprinting of autologous skin cells accelerates wound healing of extensive excisional full-thickness wounds. Sci Rep, 9: 1856. https://doi.org/10.1038/s41598-018-38366-w
29. Pazhouhnia Z, Beheshtizadeh N, Namini MS, et al., 2022, Portable hand-held bioprinters promote in situ tissue regeneration. Bioeng Transl Med, 7: e10307. https://doi.org/10.1002/btm2.10307
30. Campos DF, Zhang S, Kreimendahl F, et al., 2020, Hand-held bioprinting for de novo vascular formation applicable to dental pulp regeneration. Connect Tissue Res, 61: 205–215. https://doi.org/10.1080/03008207.2019.1640217
31. Osidak EO, Karalkin PA, Osidak MS, et al., 2019, Viscoll collagen solution as a novel bioink for direct 3D bioprinting. J Mater Sci Mater Med, 30: 31. https://doi.org/10.1007/s10856-019-6233-y
32. Shansky YD, Sergeeva NS, Sviridova IK, et al., 2019, Human platelet lysate sustains the osteogenic/adipogenic differentiation potential of adipose-derived mesenchymal stromal cells and maintains their dna integrity in vitro. Cells Tissues Organs, 207: 149–164. https://doi.org/10.1159/000502813
33. Lang S, Loibl M, Herrmann M, 2018, Platelet-rich plasma in tissue engineering: Hype and hope. Eur Surg Res, 59: 265–275. https://doi.org/10.1159/000492415
34. Nuutila K, Samandari M, Endo Y, et al., 2022, In vivo printing of growth factor-eluting adhesive scaffolds improves wound healing. Bioact Mater, 8: 296–308. https://doi.org/10.1016/j.bioactmat.2021.06.030
35. Montesano R, Orci L, 1988, Transforming growth factor beta stimulates collagen-matrix contraction by fibroblasts: Implications for wound healing. Proc Natl Acad Sci U S A, 85(13):4894-7. https://doi.org/10.1073/pnas.85.13.4894
36. Popp CM, Miller WC, Eide CR, et al., 2022, Future applications of 3D bioprinting: A promising technology for treating recessive dystrophic epidermolysis bullosa. Exp Dermatol, 31: 384–392. https://doi.org/10.1111/exd.14484