Application of 3D-printed tissue-engineered skin substitute using innovative biomaterial loaded with human adipose-derived stem cells in wound healing
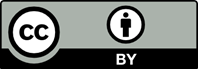
Large-scale skin injuries are usually accompanied by impaired wound healing, resulting in scar formation, or significant morbidity and mortality. The aim of this study is to explore the in vivo application of 3D-printed tissue-engineered skin substitute using innovative biomaterial loaded with human adipose-derived stem cells (hADSCs) in wound healing. Adipose tissue was decellularized, and extracellular matrix components were lyophilized and solubilized to obtain adipose tissue decellularized extracellular matrix (dECM) pre-gel. The newly designed biomaterial is composed of adipose tissue dECM pre-gel, methacrylated gelatin (GelMA), and methacrylated hyaluronic acid (HAMA). Rheological measurement was performed to evaluate the phase-transition temperature and the storage and loss modulus at this temperature. Tissue-engineered skin substitute loaded with hADSCs was fabricated by 3D printing. We used nude mice to establish full-thickness skin wound healing model and divided them into four groups randomly: (A) Full-thickness skin graft treatment group, (B) 3D-bioprinted skin substitute treatment group as the experimental group, (C) microskin graft treatment group, and (D) control group. The amount of DNA in each milligram of dECM was 24.5 ± 7.1 ng, fulfilling the currently accepted decellularization criteria. The solubilized adipose tissue dECM was thermo-sensitive biomaterial and underwent a sol-gel phase transition when temperature rises. The dECM-GelMA-HAMA precursor undergoes a gel-sol phase transition at 17.5°C, where the storage and loss modulus of the precursor is about 8 Pa. The scanning electron microscope showed that the interior of crosslinked dECM-GelMA-HAMA hydrogel is 3D porous network structure with suitable porosity and pore size. The shape of the skin substitute is stable with regular grid-like scaffold structure. Wound healing in the experimented animals was accelerated after being treated with 3D-printed skin substitute, which attenuate inflammatory response, increase blood perfusion around the wound, as well as promote re-epithelialization, collagen deposition and alignment, and angiogenesis. In summary, 3D-printed dECM-GelMA-HAMA tissue-engineered skin substitute loaded with hADSCs, which can be fabricated by 3D printing, can accelerate wound healing and improve healing quality by promoting angiogenesis. The hADSCs and the stable 3D-printed stereoscopic grid-like scaffold structure play a critical role in promoting wound healing.
1. Eke G, Mangir N, Hasirci N, et al., 2017, Development of a UV cross-linked biodegradable hydrogel containing adipose derived stem cells to promote vascularization for skin wounds and tissue engineering. Biomaterials, 129: 188–198.
https://doi.org/10.1016/j.biomaterials.2017.03.021
2. Weng T, Zhang W, Xia Y, et al., 2021, 3D bioprinting for skin tissue engineering: Current status and perspectives. J Tissue Eng, 12: 20417314211028574. https://doi.org/10.1177/20417314211028574
3. Goodarzi P, Falahzadeh K, Nematizadeh M, et al., 2018, Tissue engineered skin substitutes. Adv Exp Med Biol, 1107: 143–188. https://doi.org/10.1007/5584_2018_226
4. Mu L, Zeng J, Huang Y, et al., 2021, Experimental study on tissue engineered cartilage constructed by three-dimensional bioprinted human adipose-derived stem cells combined with gelatin methacryloyl. Zhongguo Xiu Fu Chong Jian Wai Ke Za Zhi, 35: 896–903. https://doi.org/10.7507/1002-1892.202101049
5. Getova VE, van Dongen JA, Brouwer LA, et al., 2019, Adipose tissue-derived ECM hydrogels and their use as 3D culture scaffold. Artif Cells Nanomed Biotechnol, 47: 1693–1701. https://doi.org/10.1080/21691401.2019.1608215
6. Chen Z, Zhang B, Shu J, et al., 2021, Human decellularized adipose matrix derived hydrogel assists mesenchymal stem cells delivery and accelerates chronic wound healing. J Biomed Mater Res A, 109: 1418–1428. https://doi.org/10.1002/jbm.a.37133
7. Kang MS, Kwon M, Lee SH, et al., 2022, 3D Printing of skin equivalents with hair follicle structures and epidermal-papillary-dermal layers using gelatin/hyaluronic acid hydrogels. Chem Asian J, 17: e202200620. https://doi.org/10.1002/asia.202200620
8. Zhou ZQ, Chen Y, Chai M, et al., 2019, Adipose extracellular matrix promotes skin wound healing by inducing the differentiation of adiposederived stem cells into fibroblasts. Int J Mol Med, 43: 890–900. https://doi.org/10.3892/ijmm.2018.4006
9. Crapo PM, Gilbert TW, Badylak SF, 2011, An overview of tissue and whole organ decellularization processes. Biomaterials, 32: 3233–3243. https://doi.org/10.1016/j.biomaterials.2011.01.057
10. Pu W, Ren J, Chen Y, et al., 2020, Injectable human decellularized adipose tissue hydrogel containing stem cells enhances wound healing in mouse. Colloids Surf A Physicochem Eng Asp, 604: 125268. https://doi.org/10.1016/j.colsurfa.2020.125268
11. Chen M, Przyborowski M, Berthiaume F, 2009, Stem cells for skin tissue engineering and wound healing. Crit Rev Biomed Eng, 37: 399–421. https://doi.org/10.1615/critrevbiomedeng.v37.i4-5.50
12. Choi YC, Choi JS, Woo CH, et al., 2014, Stem cell delivery systems inspired by tissue-specific niches. J Control Release, 193: 42-50. https://doi.org/10.1016/j.jconrel.2014.06.032
13. Cho KH, Uthaman S, Park IK, et al., 2018, Injectable biomaterials in plastic and reconstructive surgery: A review of the current status. Tissue Eng Regen Med, 15: 559–574. https://doi.org/10.1007/s13770-018-0158-2
14. Zhao Y, Fan J, Bai S, 2019, Biocompatibility of injectable hydrogel from decellularized human adipose tissue in vitro and in vivo. J Biomed Mater Res B Appl Biomater, 107: 1684–1694. https://doi.org/10.1002/jbm.b.34261
15. Zhu J, Marchant RE, 2011, Design properties of hydrogel tissue-engineering scaffolds. Expert Rev Med Devices, 8: 607–626. https://doi.org/10.1586/erd.11.27
16. Murphy SV, Skardal A, Atala A, 2013, Evaluation of hydrogels for bio-printing applications. J Biomed Mater Res A, 101: 272–284. https://doi.org/10.1002/jbm.a.34326
17. Xu P, Guan J, Chen Y, et al., 2021, Stiffness of photocrosslinkable gelatin hydrogel influences nucleus pulposus cell properties in vitro. J Cell Mol Med, 25: 880–891. https://doi.org/10.1111/jcmm.16141
18. Schuurman W, Levett PA, Pot MW, et al., 2013, Gelatin-methacrylamide hydrogels as potential biomaterials for fabrication of tissue-engineered cartilage constructs. Macromol Biosci, 13: 551–561. https://doi.org/10.1002/mabi.201200471
19. Guan G, Qizhuang L, Liu S, et al., 2022, 3D-bioprinted peptide coupling patches for wound healing. Mater Today Bio, 13: 100188. https://doi.org/10.1016/j.mtbio.2021.100188
20. Camci-Unal G, Cuttica D, Annabi N, et al., 2013, Synthesis and characterization of hybrid hyaluronic acid-gelatin hydrogels. Biomacromolecules, 14: 1085–1092. https://doi.org/10.1021/bm3019856
21. Mazini L, Rochette L, Admou B, et al., 2020, Hopes and limits of adipose-derived stem cells (ADSCs) and mesenchymal stem cells (MSCs) in wound healing. Int J Mol Sci, 21: 1306. https://doi.org/10.3390/ijms21041306.
22. Li CY, Wu XY, Tong JB, et al., 2015, Comparative analysis of human mesenchymal stem cells from bone marrow and adipose tissue under xeno-free conditions for cell therapy. Stem Cell Res Ther, 6: 55. https://doi.org/10.1186/s13287-015-0066-5
23. Hassanshahi A, Hassanshahi M, Khabbazi S, et al., 2019, Adipose-derived stem cells for wound healing. J Cell Physiol, 234: 7903–7914. https://doi.org/10.1002/jcp.27922
24. Boggio P, Tiberio R, Gattoni M, et al., 2008, Is there an easier way to autograft skin in chronic leg ulcers? ‘Minced micrografts’, a new technique. J Eur Acad Dermatol Venereol, 22: 1168–1172. https://doi.org/10.1111/j.1468-3083.2008.02737.x
25. Zhang ML, 1987, The use of microskin-grafting in extensive burns. Zhonghua Zheng Xing Shao Shang Wai Ke Za Zhi, 3: 100–102, 156.
26. Mota C, Puppi D, Chiellini F, et al., 2015, Additive manufacturing techniques for the production of tissue engineering constructs. J Tissue Eng Regen Med, 9: 174–190. https://doi.org/10.1002/term.1635
27. Siddiqui N, Asawa S, Birru B, et al., 2018, PCL-based composite scaffold matrices for tissue engineering applications. Mol Biotechnol, 60: 506–532. https://doi.org/10.1007/s12033-018-0084-5