Fabrication of 3D gel-printed β-tricalcium phosphate/titanium dioxide porous scaffolds for cancellous bone tissue engineering
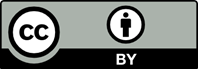
Human bone is composed of cortical bone and cancellous bone. The interior portion of natural bone is cancellous with a porosity of 50%–90%, but the outer layer is made of dense cortical bone, of which porosity was not higher than 10%. Porous ceramics were expected to be research hotspot in bone tissue engineering by virtue of their similarity to the mineral constituent and physiological structure of human bone. However, it is challenging to utilize conventional manufacturing methods to fabricate porous structures with precise shapes and pore sizes. Three-dimensional (3D) printing of ceramics is currently the latest research trend because it has many advantages in the fabrication of porous scaffolds, which can meet the requirements of cancellous bone strength, arbitrarily complex shapes, and individualized design. In this study, β-tricalcium phosphate (β-TCP)/titanium dioxide (TiO2 ) porous ceramics scaffolds were fabricated by 3D gel-printing sintering for the first time. The chemical constituent, microstructure, and mechanical properties of the 3D-printed scaffolds were characterized. After sintering, a uniform porous structure with appropriate porosity and pore sizes was observed. Besides, biological mineralization activity and biocompatibility were evaluated by in vitro cell assay. The results demonstrated that the incorporation of TiO2 (5 wt%) significantly improved the compressive strength of the scaffolds, with an increase of 283%. Additionally, the in vitro results showed that the β-TCP/TiO2 scaffold had no toxicity. Meanwhile, the adhesion and proliferation of MC3T3-E1 cells on scaffolds were desirable, revealing that the β-TCP/TiO2 scaffolds can be used as a promising candidate for repair scaffolding in orthopedics and traumatology.
1. Ribas RG, Schatkoski VM, Do Amaral Montanheiro TL, et al., 2019, Current advances in bone tissue engineering concerning ceramic and bioglass scaffolds: A review. Ceram Int, 45(17):21051–21061.
2. Brunello G, Sivolella S, Meneghello R, et al., 2016, Powder-based 3D printing for bone tissue engineering. Biotechnol Adv, 34(5):740–753.
3. Salhotra A, Shah HN, Levi B, et al., 2020, Mechanisms of bone development and repair. Nat Rev Mol Cell Biol, 21(11):696–711.
4. Palmer S, Gibbons C, Athanasou N, 1999, The pathology of bone allograft. J Bone Joint Surg Br, 81(2):333–335.
5. Meißner R, Bertol L, Rehman MAU, et al., 2019, Bioprinted 3D calcium phosphate scaffolds with gentamicin releasing capability. Ceram Int, 45(6):7090–7094.
6. Mohan N, Palangadan R, Fernandez FB, et al., 2018, Preparation of hydroxyapatite porous scaffold from a ‘coral-like’ synthetic inorganic precursor for use as a bone substitute and a drug delivery vehicle. Mater Sci Eng C, 92:329–337.
7. Ravanbakhsh H, Luo Z, Zhang X, et al., 2022, Freeform cell-laden cryobioprinting for shelf-ready tissue fabrication and storage. Matter, 5(2):573–593.
8. Amini AR, Laurencin CT, Nukavarapu SP, 2012, Bone tissue engineering: Recent advances and challenges. Crit Rev Biomed Eng, 40(5):363-408.
9. Salgado AJ, Coutinho OP, Reis RL, 2004, Bone tissue engineering: State of the art and future trends. Macromol Biosci, 4(8):743–765.
10. Moore WR, Graves SE, Bain GI, 2001, Synthetic bone graft substitutes. ANZ J Surg, 71(6):354–361.
11. Hu X, Zhao W, Zhang Z, et al., 2023, Novel 3D printed shape-memory PLLA-TMC/GA-TMC scaffolds for bone tissue engineering with the improved mechanical properties and degradability. Chin Chem Lett, 34(1):107451.
12. Lu J, Hu X, Yuan T, et al., 2022, 3D-printed poly (P-dioxanone) stent for endovascular application: In vitro evaluations. Polymers, 14(9):1751.
13. Hu X, Lin Z, He J, et al., 2022, Recent progress in 3D printing degradable polylactic acid‐based bone repair scaffold for the application of cancellous bone defect. MedComm Biomater Appl, 1(1):e14.
14. Ghahsareh ZS, Banijamali S, Aghaei A, 2022, Cerium oxide containing canasite based glass-ceramics for dental applications: Crystallization behavior, mechanical and chemical properties. Ceram Int, 48(6):8489–8501.
15. Chen Q, Thouas GA, 2015, Metallic implant biomaterials. Mater Sci Eng R, 87:1–57.
16. Li Y, Xie K, Wang C, et al., 2021, 3D printing of tricalcium phosphate/poly lactic-co-glycolic acid scaffolds loaded with carfilzomib for treating critical-sized rabbit radial bone defects. Int J Bioprint, 7(4):405.
17. Zheng C, Attarilar S, Li K, et al., 2021, 3D-printed HA15- loaded β-tricalcium phosphate/poly (lactic-co-glycolic acid) bone tissue scaffold promotes bone regeneration in rabbit radial defects. Int J Bioprint, 7(1):317.
18. Ma PX, 2008, Biomimetic materials for tissue engineering. Adv Drug Delivery Rev, 60(2):184–198.
19. Srinivasan B, Kolanthai E, Nivethaa E, et al., 2022, Enhanced in vitro inhibition of MCF-7 and magnetic properties of cobalt incorporated calcium phosphate (HAp and β-TCP) nanoparticles. Ceram Int, 49(1):855–861.
20. Mofakhami S, Salahinejad E, 2021, Biphasic calcium phosphate microspheres in biomedical applications. J Control Release, 338:527–536.
21. Lobo SE, Arinzeh TL, 2010, Biphasic calcium phosphate ceramics for bone regeneration and tissue engineering applications. Materials, 3(2):815–826.
22. Deisinger U. Generating porous ceramic scaffolds: processing and properties; proceedings of the Key Engineering Materials, F, 2010 [C]. Trans Tech Publ. Key Eng Mater, 441:155–179
23. Wongwitwichot P, Kaewsrichan J, Chua K, et al., 2010, Comparison of TCP and TCP/HA hybrid scaffolds for osteoconductive activity. Open Biomed Eng J, 4:279.
24. Shahrouzifar M, Salahinejad E, Sharifi E, 2019, Co-incorporation of strontium and fluorine into diopside scaffolds: Bioactivity, biodegradation and cytocompatibility evaluations. Mater Sci Eng C, 103:109752.
25. Macchetta A, Turner IG, Bowen CR, 2009, Fabrication of HA/TCP scaffolds with a graded and porous structure using a camphene-based freeze-casting method. Acta Biomater, 5(4):1319–1327.
26. Gbureck U, Hölzel T, Klammert U, et al., 2007, Resorbable dicalcium phosphate bone substitutes prepared by 3D powder printing. Adv Funct Mater, 17(18):3940–3945.
27. Xu T, Zhao W, Zhu J-M, et al., 2013, Complex heterogeneous tissue constructs containing multiple cell types prepared by inkjet printing technology. Biomaterials, 34(1):130–139.
28. Söhling N, Al Zoghool S, Schätzlein E, et al., 2022, In vitro evaluation of a 20% bioglass-containing 3D printable PLA composite for bone tissue engineering. Int J Bioprint, 8(4):602.
29. Horn TJ, Harrysson OL, 2012, Overview of current additive manufacturing technologies and selected applications. Sci Prog, 95(3):255–282.
30. Yi S, Liu Q, Luo Z, et al., 2022, Micropore‐forming gelatin methacryloyl (GelMA) bioink toolbox 2.0: Designable tunability and adaptability for 3D bioprinting applications. Small, 18(25):2106357.
31. Baudín C, Benet T, Pena P, 2019, Effect of graphene on setting and mechanical behaviour of tricalcium phosphate bioactive cements. J Mech Behav Biomed Mater, 89:33–47.
32. Bakhtiyari SSE, Karbasi S, Monshi A, et al., 2016, Evaluation of the effects of nano-TiO2 on bioactivity and mechanical properties of nano bioglass-P3HB composite scaffold for bone tissue engineering. J Mater Sci Mater Med, 27(1):1–17.
33. Ghasemi S, Ghomi H, 2021, Investigation of applying chitosan coating on antibacterial and biocompatibility properties of bredigite/titanium dioxide composite scaffolds. J Biomater Appl, 36(3):406–418.
34. Melchels FP, Domingos MA, Klein TJ, et al., 2012, Additive manufacturing of tissues and organs. Prog Polym Sci, 37(8):1079–1104.
35. Naicker PK, Cummings PT, Zhang H, et al., 2005, Characterization of titanium dioxide nanoparticles using molecular dynamics simulations. J Phys Chem B, 109(32):15243–15249.
36. Arsad MS, Lee PM, Hung LK, 2011, Synthesis and characterization of hydroxyapatite nanoparticles and β-TCP particles. Proceedings of the 2nd International Conference on Biotechnology and Food Science, IPCBEE, F.
37. Peters RJ, Van Bemmel G, Herrera-Rivera Z, et al., 2014, Characterization of titanium dioxide nanoparticles in food products: Analytical methods to define nanoparticles. J Agric Food Chem, 62(27):6285–6293.
38. Wu T, Yu S, Chen D, et al., 2017, Bionic design, materials and performance of bone tissue scaffolds. Materials, 10(10):1187.
39. Chen Z, Li J, Liu C, et al., 2019, Preparation of high solid loading and low viscosity ceramic slurries for photopolymerization-based 3D printing. Ceram Int, 45(9):11549–11557.
40. Shepherd J, Best S, 2011, Calcium phosphate scaffolds for bone repair. JOM, 63(4):83–92.
41. Miller C, Kokubo T, Reaney I, et al., 2002, Formation of apatite layers on modified canasite glass–ceramics in simulated body fluid. J Biomed Mater Res, 59(3):473–480.
42. Li P, Ohtsuki C, Kokubo T, et al., 1993, Process of formation of bone-like apatite layer on silica gel. J Mater Sci Mater Med, 4(2):127–131.
43. Gandolfi MG, Taddei P, Tinti A, et al., 2011, Alpha-TCP improves the apatite-formation ability of calcium-silicate hydraulic cement soaked in phosphate solutions. Mater Sci Eng C, 31(7):1412–1422.