Preparation and characterization of 3D-printed antibacterial hydrogel with benzyl isothiocyanate
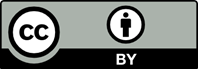
Benzyl isothiocyanate (BITC) is an isothiocyanate of plant origin, especially the mustard family, which has good antibacterial properties. However, its applications are challenging due to its poor water solubility and chemical instability. We used food hydrocolloids, including xanthan gum, locust bean gum, konjac glucomannan, and carrageenan as three-dimensional (3D)-printing food ink base and successfully prepared 3D-printed BITC antibacterial hydrogel (BITC-XLKC-Gel). The characterization and fabrication procedure of BITC-XLKC-Gel was studied. The results show that BITC-XLKC-Gel hydrogel has better mechanical properties by lowfield nuclear magnetic resonance (LF-NMR), mechanical properties, and rheometer analysis. The strain rate of BITC-XLKC-Gel hydrogel is 76.5%, which is better than that of human skin. Scanning electron microscope (SEM) analysis showed that BITC-XLKC-Gel has uniform pore size and provides a good carrier environment for BITC carriers. In addition, BITC-XLKC-Gel has good 3D-printing performance, and 3D printing can be used for customizing patterns. Finally, inhibition zone analysis showed that the BITC-XLKC-Gel added with 0.6% BITC had strong antibacterial activity against Staphylococcus aureus and the BITC-XLKC-Gel added with 0.4% BITC had strong antibacterial activity against Escherichia coli. Antibacterial wound dressing has always been considered essential in burn wound healing. In experiments that simulated burn infection, BITC-XLKC-Gel showed good antimicrobial activity against methicillin-resistant S. aureus. BITC-XLKC-Gel is a good 3D-printing food ink attributed to strong plasticity, high safety profile, and good antibacterial performance and has great application prospects.
1. Wu X, Zhou QH, Xu K, 2009, Are isothiocyanates potential anti-cancer drugs? Acta Pharmacol Sin, 30: 501–512. https://doi.org/10.1038/aps.2009.50
2. Nakamura Y, Yoshimoto M, Murata Y, et al., 2007, Papaya seed represents a rich source of biologically active isothiocyanate. J Agric Food Chem, 55: 4407–4413. https://doi.org/10.1021/jf070159w
3. Li P, Zhao YM, Wang C, et al., 2021, Antibacterial activity and main action pathway of benzyl isothiocyanate extracted from papaya seeds. J Food Sci, 86: 169–176. https://doi.org/10.1111/1750-3841.15539
4. Li H, Ming X, Xu D, et al., 2021, Transcriptome analysis and weighted gene co-expression network reveal multitarget-directed antibacterial mechanisms of benzylisothiocyanate against Staphylococcus aureus. J Agric Food Chem, 69: 11733–11741. https://doi.org/10.1021/acs.jafc.1c03979
5. Romeo L, Iori R, Rollin P, et al., 2018, Isothiocyanates: An overview of their antimicrobial activity against human infections. Molecules, 23: 624. https://doi.org/10.3390/molecules23030624
6. Uppal S, Kaur K, Kumar R, et al., 2018, Chitosan nanoparticles as a biocompatible and efficient nanowagon for benzyl isothiocyanate. Int J Biol Macromol, 115: 18–28. https://doi.org/10.1016/j.ijbiomac.2018.04.036
7. Uppal S, Sharma P, Kumar R, et al., 2020, Effect of benzyl isothiocyanate encapsulated biocompatible nanoemulsion prepared via ultrasonication on microbial strains and breast cancer cell line MDA MB 231. Colloids Surf A Physicochem Eng Asp, 596: 124732. https://doi.org/10.1016/j.colsurfa.2020.124732
8. Li W, Liu X, Yang Q, et al., 2015, Preparation and characterization of inclusion complex of benzyl isothiocyanate extracted from papaya seed with β-cyclodextrin. Food Chem, 184: 99–104. https://doi.org/10.1016/j.foodchem.2015.03.091
9. Ramirez-Blanco CE, Ramirez-Rivero CE, Diaz-Martinez LA, et al., 2017, Infection in burn patients in a referral center in Colombia. Burns, 43: 642–653. https://doi.org/10.1016/j.burns.2016.07.008
10. Keswani RK, Miglani OP, Sabherwai U, et al., 1982, Infection in burn patients. Burns, 8: 256–262. https://doi.org/10.1016/0305-4179(82)90006-7
11. Bagdonas R, Tamelis A, Rimdeika R, 2003, Staphylococcus aureus infection in the surgery of burns. Medicina (Kaunas), 39: 1078–1081.
12. Lowy FD, 1998, Staphylococcus aureus infections. N Engl J Med, 339: 520–532. https://doi.org/10.1056/NEJM199808203390806
13. Ladhani HA, Yowler CJ, Claridge JA, 2021, Burn wound colonization, infection, and sepsis. Surg Infect (Larchmt), 22: 44–48. https://doi.org/10.1089/sur.2020.346
14. Lima T, Passos MF, 2021, Skin wounds, the healing process, and hydrogel-based wound dressings: A short review. J Biomater Sci Polym Sci, 32: 1910–1925. https://doi.org/10.1080/09205063.2021.1946461
15. Kopecki Z, 2021, Development of next-generation antimicrobial hydrogel dressing to combat burn wound infection. Biosci Rep, 41: BSR20203404. https://doi.org/10.1042/BSR20203404
16. Kamoun EA, Kenawy ES, Chen X, 2017, A review on polymeric hydrogel membranes for wound dressing applications: PVA-based hydrogel dressings. J Adv Res, 8: 217–233. https://doi.org/10.1016/j.jare.2017.01.005
17. Kim H, 2018, Wound dressing materials: The essentials. J Wound Manag Res, 14: 141–142. https://doi.org/10.22467/jwmr.2018.00458
18. Zhang M, Zhao X, 2020, Alginate hydrogel dressings for advanced wound management. Int J Biol Macromol, 162: 1414–1428. https://doi.org/10.1016/j.ijbiomac.2020.07.311
19. Barak S, Mudgil D, 2014, Locust bean gum: Processing, properties and food applications--a review. Int J Biol Macromol, 66: 74–80. https://doi.org/10.1016/j.ijbiomac.2014.02.017
20. Chen Y, Zhang M, Bhandari B, 2021, 3D printing of steak-like foods based on textured soybean protein. Foods, 10: 2011. https://doi.org/10.3390/foods10092011
21. McKim JM, 2014, Food additive carrageenan: Part I: A critical review of carrageenan in vitro studies, potential pitfalls, and implications for human health and safety. Crit Rev Toxicol, 44: 211–243. https://doi.org/10.3109/10408444.2013.861797
22. Li JQ, Geng S, Zhen SY, et al., 2022, Fabrication and characterization of oil-in-water emulsions stabilized by whey protein isolate/phloridzin/sodium alginate ternary complex. Food Hydrocolloids, 129: 107625. https://doi.org/10.1016/j.foodhyd.2022.107625
23. Ma W, Dong W, Zhao S, et al., 2022, An injectable adhesive antibacterial hydrogel wound dressing for infected skin wounds. Biomater Adv, 134: 112584. https://doi.org/10.1016/j.msec.2021.112584
24. Yoon WB, Gunasekaran S, Park JW, 2006, Characterization of thermorheological behavior of Alaska Pollock and Pacific whiting surimi. J Food Sci, 69: 338–343. https://doi.org/10.1111/j.1365-2621.2004.tb13639.x
25. Zou Q, Tian X, Luo S, et al., 2021, Agarose composite hydrogel and PVA sacrificial materials for bioprinting large-scale, personalized face-like with nutrient networks. Carbohydr Polym, 269: 118222. https://doi.org/10.1016/j.carbpol.2021.118222
26. Xiu H, Zhao H, Dai L, et al., 2022, Robust and adhesive lignin hybrid hydrogel as an ultrasensitive sensor. Int J Biol Macromol, 213: 226–233. https://doi.org/10.1016/j.ijbiomac.2022.05.168
27. Qi L, Wang Y, Chen B, et al., 2021, In vitro bacteriostatic effects of polymyxin B combined with propofol medium and long chain fat emulsion injection against Escherichia coli. Ann Palliat Med, 10: 4687-4687. https://doi.org/10.21037/apm-21-751
28. Chen H, Zhou Y, Zhou X, et al., 2020, Dimethylaminododecyl methacrylate inhibits Candida albicans and oropharyngeal candidiasis in a pH-dependent manner. Appl Microbiol Biotechnol, 104:3585–3595. https://doi.org/10.1007/s00253-020-10496-0
29. Liu J, Jiang J, Zong J, et al., 2021, Antibacterial and anti-biofilm effects of fatty acids extract of dried Lucilia sericata larvae against Staphylococcus aureus and Streptococcus pneumoniae in vitro. Nat Prod Res, 35: 1702–1705. https://doi.org/10.1080/14786419.2019.1627353
30. Lin S, Pei L, Zhang W, et al., 2021, Chitosan-poloxamer-based thermosensitive hydrogels containing zinc gluconate/ recombinant human epidermal growth factor benefit for antibacterial and wound healing. Mater Sci Eng C Mater Biol Appl, 130: 112450. https://doi.org/10.1016/j.msec.2021.112450
31. Katoch A, Choudhury AR, 2020, Understanding the rheology of novel guar-gellan gum composite hydrogels. Mater Lett, 263: 127234. https://doi.org/10.1016/j.matlet.2019.127234
32. Huang M, Mao Y, Li H, et al., 2021, Kappa-carrageenan enhances the gelation and structural changes of egg yolk via electrostatic interactions with yolk protein. Food Chem, 360: 129972. https://doi.org/10.1016/j.foodchem.2021.129972
33. Janarthanan G, Shin HS, Kim IG, et al., 2020, Self-crosslinking hyaluronic acid-carboxymethylcellulose hydrogel enhances multilayered 3D-printed construct shape integrity and mechanical stability for soft tissue engineering. Biofabrication, 12: 045026. https://doi.org/10.1088/1758-5090/aba2f7
34. Ma W, Zhou M, Dong W, et al., 2021, A bi-layered scaffold of a poly (lactic-co-glycolic acid) nanofiber mat and an alginate-gelatin hydrogel for wound healing. J Mater Chem B, 9: 7492–7505. https://doi.org/10.1039/d1tb01039e
35. Annabi N, Rana D, Sani S, et al., 2017, Engineering a sprayable and elastic hydrogel adhesive with antimicrobial properties for wound healing. Biomaterials, 139, 229–243. https://doi.org/10.1016/j.biomaterials.2017.05.011
36. Bertram HC, Engelsen SB, Busk, et al., 2004, Water properties during cooking of pork studied by low-field NMR relaxation: Effects of curing and the RN(-)-gene. Meat Sci, 66: 437–446. https://doi.org/10.1016/S0309-1740(03)00132-3
37. Pearce KL, Rosenvold K, Andersen HJ, et al., 2011, Water distribution and mobility in meat during the conversion of muscle to meat and ageing and the impacts on fresh meat quality attributes--a review. Meat Sci, 89: 111–124. https://doi.org/10.1016/j.meatsci.2011.04.007
38. Yang KC, Wu CC, Cheng YH, et al., 2008, Chitosan/gelatin hydrogel prolonged the function of insulinoma/agarose microspheres in vivo during xenogenic transplantation. Transplant Proc, 40: 3623–3626. https://doi.org/10.1016/j.transproceed.2008.06.092
39. Chen F, Chen C, Zhao D, et al., 2020, On-line monitoring of the sol-gel transition temperature of thermosensitive chitosan/β-glycerophosphate hydrogels by low field NMR. Carbohydr Polym, 238: 116196. https://doi.org/10.1016/j.carbpol.2020.116196
40. Kooistra-Smid AM, van Zanten E, Ott A, et al., 2008, Prevention of Staphylococcus aureus burn wound colonization by nasal mupirocin. Burns, 34: 835–839. https://doi.org/10.1016/j.burns.2007.09.011
41. Azzopardi EA, Azzopardi E, Camilleri L, et al., 2014, Gram negative wound infection in hospitalised adult burn patients--systematic review and metanalysis-. PloS One, 9: e95042. https://doi.org/10.1371/journal.pone.0095042
42. Venkatesan N, Perumal G, Doble M, 2015, Bacterial resistance in biofilm-associated bacteria. Future Microbiol, 10: 1743–1750. https://doi.org/10.2217/fmb.15.69
43. Tu C, Wang Y, Yi L, et al., 2019, Roles of signaling molecules in biofilm formation. Sheng Wu Gong Cheng Xue Bao, 35: 558–566. https://doi.org/10.13345/j.cjb.180326
44. Del Pozo JL, 2018, Biofilm-related disease. Expert Rev Anti Infect Ther, 16: 51–65. https://doi.org/10.1080/14787210.2018.1417036
45. Church D, Elsayed S, Reid O, et al., 2006, Burn wound infections. Clin Microbiol Rev, 19: 403–434. https://doi.org/10.1128/CMR.19.2.403-434.2006
46. Lachiewicz AM, Hauck CG, Weber DJ, et al., 2017, Bacterial infections after burn injuries: Impact of multidrug resistance. Clin Infect Dis, 65: 2130–2136. https://doi.org/10.1093/cid/cix682
47. Chen K, Lin S, Li P, et al., 2018, Characterization of Staphylococcus aureus isolated from patients with burns in a regional burn center, Southeastern China. BMC Infect Dis, 18: 51. https://doi.org/10.1186/s12879-018-2955-6
48. Reardon CM, Brown TP, Stephenson AJ, et al., 1998, Methicillin-resistant Staphylococcus aureus in burns patients--why all the fuss? Burns, 24: 393–397. https://doi.org/10.1016/s0305-4179(98)00036-9
49. Chanda A, 2018, Biomechanical modeling of human skin tissue surrogates. Biomimetics (Basel), 3: 18. https://doi.org/10.3390/biomimetics3030018