Methodology for characterizing the printability of hydrogels
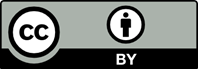
Currently, the characterization techniques for hydrogels used in bioprinting are extensive, and they could provide data on the physical, chemical, and mechanical properties of hydrogels. While characterizing the hydrogels, the analysis of their printing properties is of great importance in the determination of their potential for bioprinting. The study of printing properties provides data on their capacity to reproduce biomimetic structures and maintain their integrity after the process, as it also relates them to the possible cell viability after the generation of the structures. Current hydrogel characterization techniques require expensive measuring instrument that is not readily available in many research groups. Therefore, it would be interesting to propose a methodology to characterize and compare the printability of different hydrogels in a fast, simple, reliable, and inexpensive way. The aim of this work is to propose a methodology for extrusion-based bioprinters that allows determining the printability of hydrogels that are going to be loaded with cells, by analyzing cell viability with the sessile drop method, molecular cohesion with the filament collapse test, adequate gelation with the quantitative evaluation of the gelation state, and printing precision with the printing grid test. The data obtained after performing this work allow the comparison of different hydrogels or different concentrations of the same hydrogel to determine which one has the most favorable properties to carry out bioprinting studies.
1. Derby B, 2012, Printing and prototyping of tissues and scaffolds. Science, 338(6109):921–926. https://doi.org/10.1126/SCIENCE.1226340
2. Reddi AH, 2004, Morphogenesis and tissue engineering of bone and cartilage: Inductive signals, stem cells, and biomimetic biomaterials. Tissue Eng, 6(4):351–359. https://doi.org/10.1089/107632700418074
3. Ghorbani F , Li D , Zhong Z, et al., 2021, Bioprinting a cell-laden matrix for bone regeneration: A focused review. J Appl Polym Sci, 138(8):49888. https://doi.org/10.1002/APP.49888
4. Li X, Cai J, Lois K, et al., 2022, Current trends in biomedical hydrogels: From traditional crosslinking to plasma-assisted synthesis. Polymers, 14(13):2560. https://doi.org/10.3390/POLYM14132560
5. Naghieh S, Chen X, 2021, Printability—A key issue in extrusion-based bioprinting. J Pharm Anal, 11(5):564–579. https://doi.org/10.1016/J.JPHA.2021.02.001
6. Cha J, Kim P, 2017, Biomimetic strategies for the glioblastoma microenvironment. Front Mater, 4:45. https://doi.org/10.3389/FMATS.2017.00045/XML/NLM
7. Shokrani H, Shokrani A, Saeb MR, 2022, Methods for biomaterials printing: A short review and perspective. Methods, 206:1–7. https://doi.org/10.1016/J.YMETH.2022.07.016
8. Jia L , Hua Y, Zeng J, et al., 2022, Bioprinting and regeneration of auricular cartilage using a bioactive bioink based on microporous photocrosslinkable acellular cartilage matrix. Bioact Mater, 16:66–81. https://doi.org/10.1016/J.BIOACTMAT.2022.02.032
9. Fatimi A, Okoro OV, Podstawczyk D, et al., 2022, Natural hydrogel-based bio-inks for 3D bioprinting in tissue engineering: A review. Gels, 8(3):179. https://doi.org/10.3390/GELS8030179
10. Bai X, Gao M, Syed S, et al., 2018, Bioactive hydrogels for bone regeneration. Bioact Mater, 3(4):401–417. https://doi.org/10.1016/J.BIOACTMAT.2018.05.006
11. Cheng Y, Shi X, Jiang X, et al., 2020, Printability of a cellulose derivative for extrusion-based 3D printing: The application on a biodegradable support material. Front Mater, 7:86. https://doi.org/10.3389/FMATS.2020.00086/BIBTEX
12. Guan X , Avci-Adali M, Alarçin E , et al., 2017, Development of hydrogels for regenerative engineering. Biotechnol J, 12(5). https://doi.org/10.1002/BIOT.201600394
13. Klak M , Kowalska P, Dobrzański T, et al., 2021, Bionic organs: Shear forces reduce pancreatic islet and mammalian cell viability during the process of 3D bioprinting. Micromachines, 12(3):304. https://doi.org/10.3390/MI12030304
14. Mancha E , Gómez JC, López E, et al., 2020, Hydrogels for bioprinting: A systematic review of hydrogels synthesis, bioprinting parameters, and bioprinted structures behavior. Front Bioeng Biotechnol, 8:776. https://doi.org/10.3389/FBIOE.2020.00776/BIBTEX
15. Russo E, Qu X, Chwalik-Pilszyk G, et al., 2022, Influence of selected ophthalmic fluids on the wettability and hydration of hydrogel and silicone hydrogel contact lenses—In vitro study. Materials, 15(3):930. https://doi.org/10.3390/MA15030930
16. González Yeguas L, Estudio de la bioimpresión de hidrogeles aplicados a la generación de piel artificial mediante impresión laser BA-LIFT [Study of the bioprinting of hydrogels applied to the generation of artificial skin by BA-LIFT laser printing]. Universidad Politécnica de Madrid, Madrid, 2020. [Article in Spanish]
17. Castillo HE, Carballo SM, Alfaro ME, et al., 2021, Hidrogeles híbridos de quitosano y polietilenglicol (quit:peg) para potenciales aplicaciones biomédicas [Hydrogels chitosan and polyethylene glycol (quit:peg) hybrids for potential applications biomedical sciences]. Rev Iberoam Polim, 22(2):97–112. [Article in Spanish]
18. Nakagawa M, Teraoka F, Fujimoto S, et al., 2006, Improvement of cell adhesion on poly(L-lactide) by atmospheric plasma treatment. J Biomed Mater Res A, 77(1):112–118. https://doi.org/10.1002/JBM.A.30521
19. Rodríguez Rego JM, 2021, Estudio de la bioimpresión 3d con aplicación en odontología a partir de una nueva metodología [Study of 3D Bioprinting With Application in Dentistry Based on a New Methodology]. Universidad de Extremadura, Badajoz. [Article in Spanish]
20. Omrani MM , Kumar H, Mohamed MGA, et al., 2020, Polyether ether ketone surface modification with plasma and gelatin for enhancing cell attachment. J Biomed Mater Res B Appl Biomater, 109(5):622–629. https://doi.org/10.1002/JBM.B.34726
21. Morello MC, 2019, Estudio del composite hidroxiapatita– acrílico como material de bioimpresión 3D [Study of the Hydroxyapatite-Acrylic Composite as a 3D Bioprinting Material]. Universidad Nacional de Córdoba, Córdoba. [Article in Spanish]
22. Gillispie GJ, Han A, Uzun-Per M, et al., 2020, The influence of printing parameters and cell density on bioink printing outcomes. Tissue Eng Part A, 26(23–24):1349–1358. https://doi.org/10.1089/TEN.TEA.2020.0210
23. He Y, Yang F, Zhao H, et al., 2016, Research on the printability of hydrogels in 3D bioprinting. Sci Rep, 6(1):1–13. https://doi.org/10.1038/srep29977
24. Ouyang L, Yao R, Zhao Y, et al., 2016, Effect of bioink properties on printability and cell viability for 3D bioplotting of embryonic stem cells. Biofabrication, 8(3). https://doi.org/10.1088/1758-5090/8/3/035020
25. Kyle S, Jessop ZM, Al-Sabah A, et al., 2017, Printability of candidate biomaterials for extrusion based 3D printing: State-of-the-art. Adv Healthc Mater, 6(16). https://doi.org/10.1002/ADHM.201700264
26. Habib MA, Khoda B, 2018, Development of clay based novel bio-ink for 3D bio-printing process. Procedia Manuf, 26:846–856. https://doi.org/10.1016/J.PROMFG.2018.07.105
27. García Villegas C, Vidarte Pastrana MM, 2011, Informe 1. Estado del arte de la bioimpresión 3D [Report 1. State of the Art of 3D Bioprinting]. Bogotá. [Article in Spanish]
28. Yang I, Salas F, Pomares G, et al., 2018, Bioimpresión de órganos y tejidos en tercera dimensión: técnicas, aplicaciones y limitaciones [3D organ and tissue bioprinting: Techniques, applications and limitations]. Rev Tecnol Marcha, 31(3):41–51. https://doi.org/10.18845/TM.V31I3.3900. [Article in Spanish]
29. César ÁA, Olivos A, Landa C, et al., 2018, Use and application of 3D printing and bioimpression technology in medicine. Rev Facult Med, 61:9.
30. Therriault D, White SR, Lewis JA, 2007, Rheological behavior of fugitive organic inks for direct-write assembly. Appl Rheol, 17:10112-1–10112-8.
31. Billiet T, Gevaert E, de Schryver T, et al., 2014, The 3D printing of gelatin methacrylamide cell-laden tissue-engineered constructs with high cell viability. Biomaterials, 35(1):49–62. https://doi.org/10.1016/J.BIOMATERIALS.2013.09.078
32. Gómez JC, Galván V, Patrocinio D, et al., 2021, Improving cell viability and velocity in μ-extrusion bioprinting with a novel pre-incubator bioprinter and a standard FDM 3D printing nozzle. Materials, 14(11):3100. https://doi.org/10.3390/MA14113100
33. Matamoros M, et al., 2020, Temperature and humidity PID controller for a bioprinter atmospheric enclosure system. Micromachines, 11(11):999. https://doi.org/10.3390/MI11110999
34. Agujetas R, Marcos AC, Fernández-Vigo JI, et al., 2019, Influence of an iris-fixed phakic intraocular lens on the transport of nutrients by the aqueous humor. Biomech Model Mechanobiol, 18(2):491–502. https://doi.org/10.1007/S10237-018-1099-3/TABLES/5
35. Matamoros M, Gómez JC, Sánchez A, et al., 2021, Development of a device for the control of variables in the bioprinting of hydrogels. 25th International Congress on Project Management and Engineering, 13.