Zn-doped chitosan/alginate multilayer coatings on porous hydroxyapatite scaffold with osteogenic and antibacterial properties
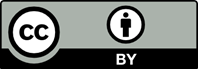
Porous hydroxyapatite (HA) scaffolds prepared by three-dimensional (3D) printing have wide application prospects owing to personalized structural design and excellent biocompatibility. However, the lack of antimicrobial properties limits its widespread use. In this study, a porous ceramic scaffold was fabricated by digital light processing (DLP) method. The multilayer chitosan/alginate composite coatings prepared by layer-by-layer method were applied to scaffolds and Zn2+ was doped into coatings in the form of ion crosslinking. The chemical composition and morphology of coatings were characterized by scanning electron microscope (SEM) and X-ray photoelectron spectroscopy (XPS). Energy dispersive spectroscopy (EDS) analysis demonstrated that Zn2+ was uniformly distributed in the coating. Besides, the compressive strength of coated scaffolds (11.52 ± 0.3 MPa) was slightly improved compared with that of bare scaffolds (10.42 ± 0.56 MPa). The result of soaking experiment indicated that coated scaffolds exhibited delayed degradation. In vitro experiments demonstrated that within the limits of concentration, a higher Zn content in the coating has a stronger capacity to promote cell adhesion, proliferation and differentiation. Although excessive release of Zn2+ led to cytotoxicity, it presented a stronger antibacterial effect against Escherichia coli (99.4%) and Staphylococcus aureus (93%).
1. Cheng H, Chawla A, Yang Y, et al., 2017, Development of nanomaterials for bone-targeted drug delivery. Drug Discov Today, 22: 1336–1350. https://doi.org/10.1016/j.drudis.2017.04.021
2. Ferreira AM, Gentile P, Chiono V, et al., 2012, Collagen for bone tissue regeneration. Acta Biomater, 8: 3191–3200. https://doi.org/10.1016/j.actbio.2012.06.014
3. Arrigoni C, Gilardi M, Bersini S, et al., 2017, Bioprinting and organ-on-chip applications towards personalized medicine for bone diseases. Stem Cell Rev Rep, 13: 407–417. https://doi.org/10.1007/s12015-017-9741-5
4. Koons GL, Diba M, Mikos AG, 2020, Materials design for bone-tissue engineering. Nat Rev Mater, 5: 584–603. https://doi.org/10.1038/s41578-020-0204-2
5. Garcia P, Histing T, Holstein JH, et al., 2013, Rodent animal models of delayed bone healing and non-union formation: A comprehensive review. Eur Cells Mater, 26: 1–12. https://doi.org/10.22203/ecm.v026a01
6. Turnbull G, Clarke J, Picard F, et al., 2018, 3D bioactive composite scaffolds for bone tissue engineering. Bioact Mater, 3: 278–314. https://doi.org/10.1016/j.bioactmat.2017.10.001
7. Aulakh TS, Jayasekera N, Kuiper JH, et al., 2009, Long-term clinical outcomes following the use of synthetic hydroxyapatite and bone graft in impaction in revision hip arthroplasty. Biomaterials, 30: 1732–1738. https://doi.org/10.1016/j.biomaterials.2008.12.035
8. Jeong J, Kim JH, Shim JH, et al., 2019, Bioactive calcium phosphate materials and applications in bone regeneration. Biomater Res, 23: 4. https://doi.org/10.1186/s40824-018-0149-3
9. Zeng JH, Liu SW, Xiong L, et al., 2018, Scaffolds for the repair of bone defects in clinical studies: A systematic review. J Orthop Surg Res, 13: 33. https://doi.org/10.1186/s13018-018-0724-2
10. Mahanty A, Shikha D, 2022, Changes in the morphology, mechanical strength and biocompatibility of polymer and metal/polymer fabricated hydroxyapatite for orthopaedic implants: A review. J Polym Eng, 42: 298–322. https://doi.org/10.1515/polyeng-2021-0171
11. Vilardell AM, Cinca N, Concustell A, et al., 2015, Cold spray as an emerging technology for biocompatible and antibacterial coatings: State of art. J Mater Sci, 50: 4441–4462. https://doi.org/10.1007/s10853-015-9013-1
12. Przekora A, 2019, Current trends in fabrication of biomaterials for bone and cartilage regeneration: Materials modifications and biophysical stimulations. Int J Mol Sci, 20: 435. https://doi.org/10.3390/ijms20020435
13. Li F, Li S, Liu Y, et al., 2022, Current advances in the roles of doped bioactive metal in biodegradable polymer composite scaffolds for bone repair: A mini review. Adv Eng Mater, 24: 2101510. https://doi.org/10.1002/adem.202101510
14. Sierra MA, Casarrubios L, de la Torre MC, 2019, Bio-organometallic derivatives of antibacterial drugs. Chemistry, 25: 7232–7242. https://doi.org/10.1002/chem.201805985
15. Li B, Webster TJ, 2018, Bacteria antibiotic resistance: New challenges and opportunities for implant-associated orthopedic infections. J Orthop Res, 36: 22–32. https://doi.org/10.1002/jor.23656
16. Godoy-Gallardo M, Eckhard U, Delgado LM, et al., 2021, Antibacterial approaches in tissue engineering using metal ions and nanoparticles: From mechanisms to applications. Bioact Mater, 6: 4470–4490. https://doi.org/10.1016/j.bioactmat.2021.04.033
17. Saidin S, Jumat MA, Amin NA, et al., 2021, Organic and inorganic antibacterial approaches in combating bacterial infection for biomedical application. Mater Sci Eng C Mater, 118: 111382. https://doi.org/10.1016/j.msec.2020.111382
18. Bhattacharjee A, Gupta A, Verma M, et al., 2019, Site-specific antibacterial efficacy and cyto/hemo-compatibility of zinc substituted hydroxyapatite. Ceram Int, 45: 12225–12233. https://doi.org/10.1016/j.ceramint.2019.03.132
19. Yang Y, Zan J, Shuai Y, et al., 2022, In Situ growth of a metal-organic framework on graphene oxide for the chemo-photothermal therapy of bacterial infection in bone repair. ACS Appl Mater Inter, 14: 21996–22005. https://doi.org/10.1021/acsami.2c0484121996
20. Wätjen W, Haase H, Biagioli M, et al., 2002, Induction of apoptosis in mammalian cells by cadmium and zinc. Environ Health Persp, 110: 865–867. https://doi.org/10.1289/ehp.110-1241262
21. Lu T, Yuan X, Zhang L, et al., 2021, High throughput synthesis and screening of zinc-doped biphasic calcium phosphate for bone regeneration. Appl Mater Today, 25: 101225. https://doi.org/10.1016/j.apmt.2021.101225
22. Shen J, Chen B, Zhai X, et al., 2021, Stepwise 3D-spatio-temporal magnesium cationic niche: Nanocomposite scaffold mediated microenvironment for modulating intramembranous ossification, Bioact Mater, 6: 503–519. https://doi.org/10.1016/j.bioactmat.2020.08.025
23. Ullah I, Siddiqui MA, Kolawole SK, et al., 2020, Synthesis, characterization and in vitro evaluation of zinc and strontium binary doped hydroxyapatite for biomedical application. Ceram Int, 46: 14448–14459. https://doi.org/10.1016/j.ceramint.2020.02.242
24. Wang Q, Tang P, Ge X, et al., 2018, Experimental and simulation studies of strontium/zinc-codoped hydroxyapatite porous scaffolds with excellent osteoinductivity and antibacterial activity. Appl Surf Sci, 462: 118–126. https://doi.org/10.1016/j.apsusc.2018.08.068
25. Campoccia D, Montanaro L, Arciola CR, 2006, The significance of infection related to orthopedic devices and issues of antibiotic resistance. Biomaterials, 27: 2331–2339. https://doi.org/10.1016/j.biomaterials.2005.11.044
26. Gasik M, 2017, Understanding biomaterial-tissue interface quality: Combined in vitro evaluation. Sci Technol Adv Mat, 18: 550–562. https://doi.org/10.1080/14686996.2017.1348872
27. Islam MM, Shahruzzaman M, Biswas S, et al., 2020, Chitosan based bioactive materials in tissue engineering applications-A review. Bioact Mater, 5: 164–183. https://doi.org/10.1016/j.bioactmat.2020.01.012
28. Aguero L, Alpdagtas S, Ilhan E, et al., 2021, Functional role of crosslinking in alginate scaffold for drug delivery and tissue engineering: A review. Eur Polym J, 160: 110807. https://doi.org/10.1016/j.eurpolymj.2021.110807
29. Huang C, Fang G, Zhao Y, et al., 2019, Bio-inspired nanocomposite by layer-by-layer coating of chitosan/ hyaluronic acid multilayers on a hard nanocellulose-hydroxyapatite matrix. Carbohyd Polym, 222: 115036. https://doi.org/10.1016/j.carbpol.2019.115036
30. Wang R, Sun L, Zhu X, et al., 2022, Carbon nanotube‐based strain sensors: Structures, fabrication, and applications. Adv Mater Technol, 2022: 2200855. https://doi.org/10.1002/admt.202200855
31. Hernandez-Gonzalez AC, Tellez-Jurado L, Rodriguez- Lorenzo LM, 2020, Alginate hydrogels for bone tissue engineering, from injectables to bioprinting: A review. Carbohyd Polym, 229: 115514. https://doi.org/10.1016/j.carbpol.2019.115514
32. Jiao C, Xie D, He Z, et al., 2022, Additive manufacturing of Bio-inspired ceramic bone Scaffolds: Structural Design, mechanical properties and biocompatibility. Mater Design, 217: 110610. https://doi.org/10.1016/j.matdes.2022.110610
33. Liang H, Yang Y, Xie D, et al., 2019, Trabecular-like Ti-6Al-4V scaffolds for orthopedic: Fabrication by selective laser melting and in vitro biocompatibility. J Mater Sci Technol, 35: 1284–1297. https://doi.org/10.1016/j.jmst.2019.01.012
34. He H, Lian J, Chen C, et al., 2022, Enabling multi-chemisorption sites on carbon nanofibers cathodes by an in-situ exfoliation strategy for high-performance Zn-Ion hybrid capacitors. Nanomicro Lett, 14: 106. https://doi.org/10.1007/s40820-022-00839-z
35. Khan MU, Razak SI, Ansari MN, et al., 2021, Development of biodegradable bio-based composite for bone tissue engineering: Synthesis, characterization and in vitro biocompatible evaluation. Polymers (Basel), 13: 3611. https://doi.org/10.3390/polym13213611
36. Li D, Liu P, Hao F, et al., 2022, Preparation and application of silver/chitosan-sepiolite materials with antimicrobial activities and low cytotoxicity. Int J Biol Macromol, 210: 337–349. https://doi.org/10.1016/j.ijbiomac.2022.05.015
37. Ding H, Peng X, Yu X, et al., 2022, The construction of a self-assembled coating with chitosan-grafted reduced graphene oxide on porous calcium polyphosphate scaffolds for bone tissue engineering. Biomed Mater, 17: 045016. https://doi.org/10.1088/1748-605X/ac6eab
38. Zhang R, Liu X, Xiong Z, et al., 2018, Novel micro/ nanostructured TiO2/ZnO coating with antibacterial capacity and cytocompatibility. Ceram Int, 44: 9711–9719. https://doi.org/10.1016/j.ceramint.2018.02.202
39. Aljohani W, Ullah MW, Zhang X, et al., 2018, Bioprinting and its applications in tissue engineering and regenerative medicine. Int J Biol Macromol, 107: 261–275. https://doi.org/10.1016/j.ijbiomac.2017.08.171
40. Bian T, Wang L, Xing H, 2021, Preparation and biological assessment of a ZrO2-based bone scaffold coated with hydroxyapatite and bioactive glass composite. Mater Chem Phys, 267: 124616. https://doi.org/10.1016/j.matchemphys.2021.124616
41. Khosrowshahi A, Khoshfetrat AB, Khosrowshahi YB, et al., 2021, Cobalt content modulates characteristics and osteogenic properties of cobalt-containing hydroxyapatite in in-vitro milieu. Mater Today Commun, 27: 102392. https://doi.org/10.1016/j.mtcomm.2021.102392
42. Wang S, Liu L, Zhou X, et al., 2019, Effect of strontium-containing on the properties of Mg-doped wollastonite bioceramic scaffolds. Biomed Eng Online, 18: 119. https://doi.org/10.1186/s12938-019-0739-x
43. Rajkumar P, Sarma BK, 2021, Role of Zn and Mg substitutions on the mechanical behaviour of biomimetic hydroxyapatite and insight of the emergence of hydroxyapatite-ZnO nanocomposite. Mater Charact, 176: 111107. https://doi.org/10.1016/j.matchar.2021.111107
44. Chen X, Zhang L, Liu X, et al., 2012, Preparation and properties of biodegradable composites derived from poly(lactide-co-glycolide), poly(L-lactide), and nanohydroxyapatite. J Macromol Sci B, 52: 462–475. https://doi.org/10.1080/00222348.2012.712007
45. Mohajernia S, Hejazi S, Eslami A, et al., 2015, Modified nanostructured hydroxyapatite coating to control the degradation of magnesium alloy AZ31 in simulated body fluid. Surf Coat Tech, 263: 54–60. https://doi.org/10.1016/j.surfcoat.2014.12.059
46. Shen Y, Liu W, Wen C, et al., 2012, Bone regeneration: Importance of local pH-strontium-doped borosilicate scaffold. J Mater Chem, 22: 8662–8670. https://doi.org/10.1039/c2jm16141a
47. Rokusek D, Davitt C, Bandyopadhyay A, et al., 2005, Interaction of human osteoblasts with bioinert and bioactive ceramic substrates. J Biomed Mater Res A, 75: 588–594. https://doi.org/10.1002/jbm.a.30459
48. Lian JB, Stein GS, 1992, Concepts of osteoblast growth and differentiation: basis for modulation of bone cell development and tissue formation. Crit Rev Oral Biol Med, 3: 269–305. https://doi.org/10.1177/10454411920030030501
49. Silva AS, Santos LF, Mendes MC, et al., 2020, Multi-layer pre-vascularized magnetic cell sheets for bone regeneration. Biomaterials, 231: 119664. https://doi.org/10.1016/j.biomaterials.2019.119664
50. Gregory CA, Gunn WG, Peister A, et al., 2004, An Alizarin red-based assay of mineralization by adherent cells in culture: Comparison with cetylpyridinium chloride extraction. Anal Biochem, 329: 77–84. https://doi.org/10.1016/j.ab.2004.02.002
51. Wang X, Liu S, Li M, et al., 2016, The synergistic antibacterial activity and mechanism of multicomponent metal ions-containing aqueous solutions against Staphylococcus aureus. J Inorg Biochem, 163: 214–220. https://doi.org/10.1016/j.jinorgbio.2016.07.019