Research progress and challenges of bioprinting in wound dressing and healing: Bibliometrics-based analysis and perspectives
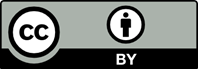
As the body’s largest organ, the skin has important roles in barrier function, immune response, prevention of water loss and excretion of waste. Patients with extensive and severe skin lesions would die due to insufficient graftable skin. Commonly used treatments include autologous skin grafts, allogeneic/allogeneic skin grafts, cytoactive factors, cell therapy, and dermal substitutes. However, traditional treatment methods are still inadequate regarding skin repair time, treatment costs, and treatment results. In recent years, the rapid development of bioprinting technology has provided new ideas to solve the above-mentioned challenges. This review describes the principles of bioprinting technology and research advances in wound dressing and healing. This review features a data mining and statistical analysis of this topic through bibliometrics. The annual publications on this topic, participating countries, and institutions were used to understand the development history. Keyword analysis was used to understand the focus of investigation and challenges in this topic. According to bibliometric analysis, bioprinting in wound dressing and healing is in an explosive phase, and future research should focus on discovering new cell sources, innovative bioink development, and developing largescale printing technology processes.
1. Singh M, Nuutila K, Kruse C, et al., 2015, Challenging the conventional therapy: Emerging skin graft techniques for wound healing. Plast Reconstr Surg, 136(4): 524e–530e.
2. Huyan Y, Lian Q, Zhao T, et al., 2020, Pilot study of the biological properties and vascularization of 3D printed bilayer skin grafts. Int J Bioprinting, 6(1): 246–246. https://doi.org/10.18063/ijb.v6i1.246
3. Ng WL, Yeong WY, 2019, The future of skin toxicology testing—Three-dimensional bioprinting meets microfluidics. Int J Bioprinting, 5(2.1): 237–237. https://doi.org/10.18063/ijb.v5i2.1.237
4. Huang J, Lei X, Huang Z, et al., 2022, Bioprinted gelatin recombinant type III collagen hydrogel promotes wound healing. Int J Bioprinting, 8(2): 517–517. https://doi.org/10.18063/ijb.v8i2.517
5. Heng MC, 2013, Signaling pathways targeted by curcumin in acute and chronic injury: Burns and photo‐damaged skin. Int J Dermatol, 52(5):531–543.
6. Tottoli EM, Dorati R, Genta I, et al., 2020, Skin wound healing process and new emerging technologies for skin wound care and regeneration. Pharmaceutics, 12(8): 735.
7. De Luca I, Pedram P, Moeini A, et al., 2021, Nanotechnology development for formulating essential oils in wound dressing materials to promote the wound-healing process: A review. Appl Sci, 11(4): 1713.
8. Capel AJ, Rimington RP, Lewis MP, et al., 2018, 3D printing for chemical, pharmaceutical and biological applications. Nat Rev Chem, 2(12): 422–436.
9. Mironov V, 2003, Printing technology to produce living tissue. Expert Opin Biol Ther, 3(5): 701–704.
10. Mironov V, Boland T, Trusk T, et al., 2003, Organ printing: Computer-aided jet-based 3D tissue engineering. TRENDS Biotechnol, 21(4): 157–161.
11. Wilson Jr WC, Boland T, 2003, Cell and organ printing 1: Protein and cell printers. Anat Rec A Discov Mol Cell Evol Biol, 272(2): 491–496.
12. Jiang T, Munguia-Lopez JG, Flores-Torres S, et al., 2019, Extrusion bioprinting of soft materials: An emerging technique for biological model fabrication. Appl Phys Rev, 6(1): 011310.
13. Zhuang P, Ng WL, An J, et al., 2019, Layer-by-layer ultraviolet assisted extrusion-based (UAE) bioprinting of hydrogel constructs with high aspect ratio for soft tissue engineering applications. PLoS One, 14(6): e0216776.
14. Li X, Liu B, Pei B, et al., 2020, Inkjet bioprinting of biomaterials. Chem Rev, 120(19): 10793–10833.
15. Ng WL, Huang X, Shkolnikov V, et al., 2022, Controlling droplet impact velocity and droplet volume: Key factors to achieving high cell viability in sub-nanoliter droplet-based bioprinting. Int J Bioprint, 8(1): 424.
16. Ng WL, Lee JM, Zhou M, et al., 2020, Vat polymerization based bioprinting—Process, materials, applications and regulatory challenges. Biofabrication, 12(2): 022001.
17. Li W, Mille LS, Robledo JA, et al., 2020, Recent advances in formulating and processing biomaterial inks for vat polymerization‐based 3D printing. Adv Healthc Mater, 9(15): 2000156.
18. Mobaraki M, Ghaffari M, Yazdanpanah A, et al., 2020, Bioinks and bioprinting: A focused review. Bioprinting, 18: e00080.
19. Derakhshanfar S, Mbeleck R, Xu K, et al., 2018, 3D bioprinting for biomedical devices and tissue engineering: A review of recent trends and advances. Bioact Mater, 3(2): 144–156.
20. Gungor-Ozkerim PS, Inci I, Zhang YS, et al., 2018, Bioinks for 3D bioprinting: An overview. Biomater Sci, 6(5): 915–946.
21. Gudapati H, Dey M, Ozbolat I, 2016, A comprehensive review on droplet-based bioprinting: Past, present and future. Biomaterials, 102: 20–42.
22. Sun W, Starly B, Daly AC, et al., 2020, The bioprinting roadmap. Biofabrication, 12(2): 022002.
23. Murphy SV, Atala A, 2014, 3D bioprinting of tissues and organs. Nat Biotechnol, 32(8): 773–785.
24. He P, Zhao J, Zhang J, et al., 2018, Bioprinting of skin constructs for wound healing. Burns Trauma, 6: 5. https://doi.org/10.1186/s41038-017-0104-x
25. Vijayavenkataraman S, Lu W, Fuh J, 2016, 3D bioprinting of skin: A state-of-the-art review on modelling, materials, and processes. Biofabrication, 8(3): 032001.
26. Varkey M, Visscher DO, van Zuijlen PPM, et al., 2019, Skin bioprinting: The future of burn wound reconstruction? Burns Trauma, 7: s41038-019-0142–7. https://doi.org/10.1186/s41038-019-0142-7
27. Wang Y, Yuan X, Yao B, et al., 2022, Tailoring bioinks of extrusion-based bioprinting for cutaneous wound healing. Bioact Mater, 17: 178–194. https://doi.org/10.1016/j.bioactmat.2022.01.024
28. Xiang S, Mao S, Chen F, et al., 2022, A bibliometric analysis of graphene in acetaminophen detection: Current status, development, and future directions. Chemosphere, 306: 135517. https://doi.org/10.1016/j.chemosphere.2022.135517
29. Pan Y, Yin C, Fernandez C, et al., 2022, A systematic review and bibliometric analysis of flame-retardant rigid polyurethane foam from 1963 to 2021. Polymers, 14(15): 3011.
30. Zheng Y, Karimi-Maleh H, Fu L, 2022, Advances in electrochemical techniques for the detection and analysis of genetically modified organisms: An analysis based on bibliometrics. Chemosensors, 10(5): 194. https://doi.org/10.3390/chemosensors10050194
31. Zheng Y, Mao S, Zhu J, et al., 2022, Current status of electrochemical detection of sunset yellow based on bibliometrics. Food Chem Toxicol, 164: 113019. https://doi.org/10.1016/j.fct.2022.113019
32. Shen Y, Mao S, Chen F, et al., 2022, Electrochemical detection of Sudan red series azo dyes: Bibliometrics based analysis. Food Chem Toxicol, 163: 112960. https://doi.org/10.1016/j.fct.2022.112960
33. Li X, Zheng Y, Wu W, et al., 2022, Graphdiyne applications in sensors: A bibliometric analysis and literature review. Chemosphere, 307: 135720. https://doi.org/10.1016/j.chemosphere.2022.135720
34. Zheng Y, Karimi-Maleh H, Fu L, 2022, Evaluation of antioxidants using electrochemical sensors: A bibliometric analysis. Sensors, 22(9): 3238. https://doi.org/10.3390/s22093238
35. Fu L, Mao S, Chen F, et al., 2022, Graphene-based electrochemical sensors for antibiotic detection in water, food and soil: A scientometric analysis in CiteSpace (2011–2021). Chemosphere, 297: 134127. https://doi.org/10.1016/j.chemosphere.2022.134127
36. Jin M, Liu J, Wu W, et al., 2022, Relationship between graphene and pedosphere: A scientometric analysis. Chemosphere, 300: 134599. https://doi.org/10.1016/j.chemosphere.2022.134599
37. Rodríguez-Salvador M, Rio-Belver RM, Garechana-Anacabe G, 2017, Scientometric and patentometric analyses to determine the knowledge landscape in innovative technologies: The case of 3D bioprinting. PLoS One, 12(6): e0180375.
38. García-García LA, Rodríguez-Salvador M, 2020, Disclosing main authors and organisations collaborations in bioprinting through network maps analysis. J Biomed Semant, 11(1): 3. https://doi.org/10.1186/s13326-020-0219-z
39. Naveau A, Smirani R, Catros S, et al., 2017, A bibliometric study to assess bioprinting evolution. Appl Sci, 7(12): 1331.
40. Rodriguez-Salvador M, Fox-Miranda I, Perez-Benitez BE, et al., 2022, Research dynamics of tissue spheroids as building blocks: A scientometric analysis. Int J Bioprint, 8(3): 585.
41. Pedroza-González SC, Rodriguez-Salvador M, Pérez Benítez BE, et al., 2021, Bioinks for 3D bioprinting: A scientometric analysis of two decades of progress. Int J Bioprint, 7(2): 333
42. Börner K, Chen C, Boyack KW, 2003, Visualizing knowledge domains. Annu Rev Inf Sci Technol, 37(1): 179–255.
43. Chen C, 2006, CiteSpace II: Detecting and visualizing emerging trends and transient patterns in scientific literature. J Am Soc Inf Sci Technol, 57(3): 359–377.
44. Chen C, 2004, Searching for intellectual turning points: Progressive knowledge domain visualization. Proc Natl Acad Sci, 101(suppl 1): 5303–5310.
45. Chen C, Ibekwe‐SanJuan F, Hou J, 2010, The structure and dynamics of cocitation clusters: A multiple‐perspective cocitation analysis. J Am Soc Inf Sci Technol, 61(7): 1386–1409.
46. Xueshu D, Wenxian, 2022, COOC is a software for bibliometrics and knowledge mapping [CP/OL]. https://github.com/2088904822. Accessed February 15, 2022.
47. Miller ED, Li K, Kanade T, et al., 2011, Spatially directed guidance of stem cell population migration by immobilized patterns of growth factors. Biomaterials, 32(11): 2775–2785. https://doi.org/10.1016/j.biomaterials.2010.12.005
48. Zhang G, Wang Z, Han F, et al., 2021, Mechano-regulation of vascular network formation without branches in 3D bioprinted cell-laden hydrogel constructs. Biotechnol Bioeng, 118(10): 3787–3798. https://doi.org/10.1002/bit.27854
49. Glover K, Mathew E, Pitzanti G, et al., 2022, 3D bioprinted scaffolds for diabetic wound-healing applications. Drug Deliv Transl Res, in-press. https://doi.org/10.1007/s13346-022-01115-8
50. Alblawi A, Ranjani AS, Yasmin H, et al., 2020, Scaffold-free: A developing technique in field of tissue engineering. Comput Methods Programs Biomed, 185: 105148. https://doi.org/10.1016/j.cmpb.2019.105148
51. Cubo N, Garcia M, Del Canizo JF, et al., 2016, 3D bioprinting of functional human skin: Production and in vivo analysis. Biofabrication, 9(1): 015006.
52. Skardal A, Mack D, Kapetanovic E, et al., 2012, Bioprinted amniotic fluid-derived stem cells accelerate healing of large skin wounds. Stem Cells Transl Med, 1(11): 792–802. https://doi.org/10.5966/sctm.2012-0088
53. Ishack S, Lipner SR, 2020, A review of 3-dimensional skin bioprinting techniques: Applications, approaches, and trends. Dermatol Surg, 46(12): 1500–1505.
54. Hamidi M, Azadi A, Rafiei P, 2008, Hydrogel nanoparticles in drug delivery. Adv Drug Deliv Rev, 60(15):1638–1649.
55. Bhattarai N, Gunn J, Zhang M, 2010, Chitosan-based hydrogels for controlled, localized drug delivery. Adv Drug Deliv Rev, 62(1): 83–99.
56. Maver T, Smrke DM, Kurečič M, et al., 2018, Combining 3D printing and electrospinning for preparation of pain-relieving wound-dressing materials. J Sol-Gel Sci Technol, 88(1): 33–48. https://doi.org/10.1007/s10971-018-4630-1
57. Abasalizadeh F, Moghaddam SV, Alizadeh E, et al., 2020, Alginate-based hydrogels as drug delivery vehicles in cancer treatment and their applications in wound dressing and 3D bioprinting. J Biol Eng, 14(1): 1–22
58. Milojević M, Harih G, Vihar B, et al., 2021, Hybrid 3D printing of advanced hydrogel-based wound dressings with tailorable properties. Pharmaceutics, 13(4): 564.
59. Hartwell R, Chan B, Elliott K, et al., 2016, Polyvinyl alcohol-graft-polyethylene glycol hydrogels improve utility and biofunctionality of injectable collagen biomaterials. Biomed Mater, 11(3): 035013. https://doi.org/10.1088/1748-6041/11/3/035013
60. Yegappan R, Selvaprithiviraj V, Amirthalingam S, et al., 2018, Carrageenan based hydrogels for drug delivery, tissue engineering and wound healing. Carbohydr Polym, 198: 385–400. https://doi.org/10.1016/j.carbpol.2018.06.086
61. Qamar SA, Junaid M, Riasat A, et al., 2022, Carrageenan-based hybrids with biopolymers and nano-structured materials for biomimetic applications. Starch - Stärke, n/a(n/a): 2200018. https://doi.org/10.1002/star.202200018
62. Xu J, Liu Y, Hsu S, 2019, Hydrogels based on Schiff base linkages for biomedical applications. Molecules, 24(16):3005.
63. Graham S, Marina PF, Blencowe A, 2019, Thermoresponsive polysaccharides and their thermoreversible physical hydrogel networks. Carbohydr Polym, 207: 143–159. https://doi.org/10.1016/j.carbpol.2018.11.053
64. Rastin H, Ramezanpour M, Hassan K, et al., 2021, 3D bioprinting of a cell-laden antibacterial polysaccharide hydrogel composite. Carbohydr Polym, 264: 117989. https://doi.org/10.1016/j.carbpol.2021.117989
65. Nowak JA, Polak L, Pasolli HA, et al., 2008, Hair follicle stem cells are specified and function in early skin morphogenesis. Cell Stem Cell, 3(1): 33–43.
66. Min D, Lee W, Bae I, et al., 2018, Bioprinting of biomimetic skin containing melanocytes. Exp Dermatol, 27(5): 453–459.
67. Shi L, Xiong L, Hu Y, et al., 2018, Three-dimensional printing alginate/gelatin scaffolds as dermal substitutes for skin tissue engineering. Polym Eng Sci, 58(10): 1782–1790. https://doi.org/10.1002/pen.24779
68. Won J-Y, Lee M-H, Kim M-J, et al., 2019, A potential dermal substitute using decellularized dermis extracellular matrix derived bio-ink. Artif Cells Nanomed Biotechnol, 47(1): 644–649.
69. Ng WL, Qi JTZ, Yeong WY, et al., 2018, Proof-of-concept: 3D bioprinting of pigmented human skin constructs. Biofabrication, 10(2): 025005.
70. Huang S, Yao B, Xie J, et al., 2016, 3D bioprinted extracellular matrix mimics facilitate directed differentiation of epithelial progenitors for sweat gland regeneration. Acta Biomater, 32: 170–177. https://doi.org/10.1016/j.actbio.2015.12.039
71. Schmitt T, Katz N, Kishore V, 2021, A feasibility study on 3D bioprinting of microfat constructs towards wound healing applications. Front Bioeng Biotechnol, 9: 707098
72. Lee V, Singh G, Trasatti JP, et al., 2014, Design and fabrication of human skin by three-dimensional bioprinting. Tissue Eng Part C Methods, 20(6): 473–484. https://doi.org/10.1089/ten.tec.2013.0335
73. Grijalvo S, Nieto-Díaz M, Maza RM, et al., 2019, Alginate hydrogels as scaffolds and delivery systems to repair the damaged spinal cord. Biotechnol J, 14(12): 1900275. https://doi.org/10.1002/biot.201900275
74. Azadmanesh F, Pourmadadi M, Zavar Reza J, et al., 2021, Synthesis of a novel nanocomposite containing chitosan as a three-dimensional printed wound dressing technique: Emphasis on gene expression. Biotechnol Prog, 37(4): e3132. https://doi.org/10.1002/btpr.3132
75. Masri S, Zawani M, Zulkiflee I, et al., 2022, Cellular interaction of human skin cells towards natural bioink via 3D-bioprinting technologies for chronic wound: A comprehensive review. Int J Mol Sci, 23(1): 476.
76. Ho J, Yue D, Cheema U, et al., 2022, Innovations in stem cell therapy for diabetic wound healing. Adv Wound Care, in-press. https://doi.org/10.1089/wound.2021.0104
77. Maharajan N, Cho GW, Jang CH, 2020, Application of mesenchymal stem cell for tympanic membrane regeneration by tissue engineering approach. Int J Pediatr Otorhinolaryngol, 133: 109969. https://doi.org/10.1016/j.ijporl.2020.109969
78. Michael S, Sorg H, Peck C-T, et al., 2013, Tissue engineered skin substitutes created by laser-assisted bioprinting form skin-like structures in the dorsal skin fold chamber in mice. PLoS One, 8(3): e57741. https://doi.org/10.1371/journal.pone.0057741
79. Albanna M, Binder KW, Murphy SV, et al., 2019, In situ bioprinting of autologous skin cells accelerates wound healing of extensive excisional full-thickness wounds. Sci Rep, 9(1): 1856. https://doi.org/10.1038/s41598-018-38366-w
80. Zhao W, Xu T, 2020, Preliminary engineering for in situ in vivo bioprinting: A novel micro bioprinting platform for in situ in vivo bioprinting at a gastric wound site. Biofabrication, 12(4): 045020. https://doi.org/10.1088/1758-5090/aba4ff
81. O’Connell CD, Di Bella C, Thompson F, et al., 2016, Development of the biopen: A handheld device for surgical printing of adipose stem cells at a chondral wound site. Biofabrication, 8(1): 015019. https://doi.org/10.1088/1758-5090/8/1/015019
82. Cheng RY, Eylert G, Gariepy J-M, et al., 2020, Handheld instrument for wound-conformal delivery of skin precursor sheets improves healing in full-thickness burns. Biofabrication, 12(2): 025002. https://doi.org/10.1088/1758-5090/ab6413
83. Ma J, Qin C, Wu J, et al., 2021, 3D printing of strontium silicate microcylinder-containing multicellular biomaterial inks for vascularized skin regeneration. Adv Healthc Mater, 10(16): 2100523. https://doi.org/10.1002/adhm.202100523
84. Ulusu Y, Dura G, Waller H, et al., 2017, Thermal stability and rheological properties of the ‘non-stick’ Caf1 biomaterial. Biomed Mater, 12(5): 051001. https://doi.org/10.1088/1748-605x/aa7a89
85. Pitton M, Fiorati A, Buscemi S, et al., 2021, 3D bioprinting of pectin-cellulose nanofibers multicomponent bioinks. Front Bioeng Biotechnol, 9: 732689.
86. Boffito M, Gioffredi E, Chiono V, et al., 2016, Novel polyurethane-based thermosensitive hydrogels as drug release and tissue engineering platforms: Design and in vitro characterization. Polym Int, 65(7): 756–769. https://doi.org/10.1002/pi.5080
87. Xia S, Weng T, Jin R, et al., 2022, Curcumin-incorporated 3D bioprinting gelatin methacryloyl hydrogel reduces reactive oxygen species-induced adipose-derived stem cell apoptosis and improves implanting survival in diabetic wounds. Burns Trauma, 10: tkac001. https://doi.org/10.1093/burnst/tkac001
88. de Rutte JM, Koh J, Di Carlo D, 2019, Scalable high-throughput production of modular microgels for in situ assembly of microporous tissue scaffolds. Adv Funct Mater, 29(25): 1900071. https://doi.org/10.1002/adfm.201900071
89. Masri S, Fauzi MB, 2021, Current insight of printability quality improvement strategies in natural-based bioinks for skin regeneration and wound healing. Polymers, 13(7): 1011.
90. Nie M, Takeuchi S, 2018, Bottom-up biofabrication using microfluidic techniques. Biofabrication, 10(4): 044103.
91. Serban MA, Skardal A, 2019, Hyaluronan chemistries for three-dimensional matrix applications. Matrix Biol, 78: 337–345.
92. Wan W, Cai F, Huang J, et al., 2019, A skin-inspired 3D bilayer scaffold enhances granulation tissue formation and anti-infection for diabetic wound healing. J Mater Chem B, 7(18):2954–2961.
93. Andriotis EG, Eleftheriadis GK, Karavasili C, et al., 2020, Development of bio-active patches based on pectin for the treatment of ulcers and wounds using 3D-bioprinting technology. Pharmaceutics, 12(1): 56.
94. Rees A, Powell LC, Chinga-Carrasco G, et al., 2015, 3D bioprinting of carboxymethylated-periodate oxidized nanocellulose constructs for wound dressing applications. BioMed Res Int, 2015: 925757. https://doi.org/10.1155/2015/925757
95. Barrett-Catton E, Ross ML, Asuri P, 2021, Multifunctional hydrogel nanocomposites for biomedical applications. Polymers, 13(6): 856.
96. Jones LO, Williams L, Boam T, et al., 2021, Cryogels: Recent applications in 3D-bioprinting, injectable cryogels, drug delivery, and wound healing. Beilstein J Org Chem, 17: 2553–2569. https://doi.org/10.3762/bjoc.17.171
97. Murphy SV, Skardal A, Atala A, 2013, Evaluation of hydrogels for bio-printing applications. J Biomed Mater Res A, 101A(1): 272–284. https://doi.org/10.1002/jbm.a.34326
98. Devi VKA, Shyam R, Palaniappan A, et al., 2021, Self-healing hydrogels: Preparation, mechanism and advancement in biomedical applications. Polymers, 13(21): 3782.
99. Miguel SP, Cabral CSD, Moreira AF, et al., 2019, Production and characterization of a novel asymmetric 3D printed construct aimed for skin tissue regeneration. Colloids Surf B Biointerfaces, 181: 994–1003. https://doi.org/10.1016/j.colsurfb.2019.06.063
100. Cai L, Liu S, Guo J, et al., 2020, Polypeptide-based self-healing hydrogels: Design and biomedical applications. Acta Biomater, 113: 84–100. https://doi.org/10.1016/j.actbio.2020.07.001
101. Zhao H, Xu J, Zhang E, et al., 2021, 3D bioprinting of polythiophene materials for promoting stem cell proliferation in a nutritionally deficient environment. ACS Appl Mater Interfaces, 13(22): 25759–25770. https://doi.org/10.1021/acsami.1c04967
102. Chinga-Carrasco G, 2018, Potential and limitations of nanocelluloses as components in biocomposite inks for three-dimensional bioprinting and for biomedical devices. Biomacromolecules, 19(3): 701–711. https://doi.org/10.1021/acs.biomac.8b00053
103. Biranje SS, Sun J, Cheng L, et al., 2022, Development of cellulose nanofibril/casein-based 3D composite hemostasis scaffold for potential wound-healing application. ACS Appl Mater Interfaces, 14(3): 3792–3808. https://doi.org/10.1021/acsami.1c21039
104. Irmak G, Gümüşderelioğlu M, 2020, Photo-activated platelet-rich plasma (PRP)-based patient-specific bio-ink for cartilage tissue engineering. Biomed Mater, 15(6): 065010. https://doi.org/10.1088/1748-605x/ab9e46
105. Reddy KLV, Murugan D, Mullick M, et al., 2020, Recent approaches for angiogenesis in search of successful tissue engineering and regeneration. Curr Stem Cell Res Ther, 15(2): 111–134. https://doi.org/10.2174/1574888X14666191104151928
106. Deng Y, Shavandi A, Okoro OV, et al., 2021, Alginate modification via click chemistry for biomedical applications. Carbohydr Polym, 270: 118360. https://doi.org/10.1016/j.carbpol.2021.118360
107. Wang M, Li H, Yang Y, et al., 2021, A 3D-bioprinted scaffold with doxycycline-controlled BMP2-expressing cells for inducing bone regeneration and inhibiting bacterial infection. Bioact Mater, 6(5): 1318–1329. https://doi.org/10.1016/j.bioactmat.2020.10.022
108. Bom S, Martins AM, Ribeiro HM, et al., 2021, Diving into 3D (bio)printing: A revolutionary tool to customize the production of drug and cell-based systems for skin delivery. Int J Pharm, 605: 120794. https://doi.org/10.1016/j.ijpharm.2021.120794
109. Datta S, Sarkar R, Vyas V, et al., 2018, Alginate-honey bioinks with improved cell responses for applications as bioprinted tissue engineered constructs. J Mater Res, 33(14): 2029–2039. https://doi.org/10.1557/jmr.2018.202
110. McKay TB, Hutcheon AEK, Guo X, et al., 2020, Modeling the cornea in 3-dimensions: Current and future perspectives. Exp Eye Res, 197: 108127. https://doi.org/10.1016/j.exer.2020.108127
111. Si H, Xing T, Ding Y, et al., 2019, 3D bioprinting of the sustained drug release wound dressing with double-crosslinked hyaluronic-acid-based hydrogels. Polymers, 11(10): 1584.
112. Kostenko A, Swioklo S, Connon CJ, 2022, Alginate in corneal tissue engineering. Biomed Mater, 17: 022004. https://doi.org/10.1088/1748-605x/ac4d7b
113. Miranda-Nieves D, Chaikof EL, 2017, Collagen and elastin biomaterials for the fabrication of engineered living tissues. ACS Biomater Sci Eng, 3(5): 694–711. https://doi.org/10.1021/acsbiomaterials.6b00250
114. Chouhan D, Dey N, Bhardwaj N, et al., 2019, Emerging and innovative approaches for wound healing and skin regeneration: Current status and advances. Biomaterials, 216: 119267. https://doi.org/10.1016/j.biomaterials.2019.119267
115. Weng T, Zhang W, Xia Y, et al., 2021, 3D bioprinting for skin tissue engineering: Current status and perspectives. J Tissue Eng, 12: 20417314211028576. https://doi.org/10.1177/20417314211028574
116. Li J, Chi J, Liu J, et al., 2017, 3D printed gelatin-alginate bioactive scaffolds combined with mice bone marrow mesenchymal stem cells: A biocompatibility study. Int J Clin Exp Pathol, 10(6): 6299–6307.
117. López-Iglesias C, Quílez C, Barros J, et al., 2020, Lidocaine-loaded solid lipid microparticles (SLMPs) produced from gas-saturated solutions for wound applications. Pharmaceutics, 12(9): 870.
118. Das AK, Gavel PK, 2020, Low molecular weight self-assembling peptide-based materials for cell culture, antimicrobial, anti-inflammatory, wound healing, anticancer, drug delivery, bioimaging and 3D bioprinting applications. Soft Matter, 16(44): 10065–10095. https://doi.org/10.1039/D0SM01136C
119. Tan CT, Liang K, Ngo ZH, et al., 2020, Application of 3D bioprinting technologies to the management and treatment of diabetic foot ulcers. Biomedicines, 8(10): 441.
120. Jorgensen AM, Varkey M, Gorkun A, et al., 2020, Bioprinted skin recapitulates normal collagen remodeling in full-thickness wounds. Tissue Eng Part A, 26(9–10): 512–526. https://doi.org/10.1089/ten.tea.2019.0319
121. Ishack S, Khachemoune A, 2022, Future prospects in 3-dimensional (3D) technology and Mohs micrographic surgery. J Dermatol Treat, 33(6): 2810–2812. https://doi.org/10.1080/09546634.2022.2080171
122. Wang Q, Xia Q, Wu Y, et al., 2015, 3D-printed atsttrin-incorporated alginate/hydroxyapatite scaffold promotes bone defect regeneration with TNF/TNFR signaling involvement. Adv Healthc Mater, 4(11): 1701–1708. https://doi.org/10.1002/adhm.201500211
123. Kuo C-Y, Eranki A, Placone JK, et al., 2016, Development of a 3D printed, bioengineered placenta model to evaluate the role of trophoblast migration in preeclampsia. ACS Biomater Sci Eng, 2(10): 1817–1826. https://doi.org/10.1021/acsbiomaterials.6b00031
124. Moakes RJA, Senior JJ, Robinson TE, et al., 2021, A suspended layer additive manufacturing approach to the bioprinting of tri-layered skin equivalents. APL Bioeng, 5(4): 046103. https://doi.org/10.1063/5.0061361
125. Antezana PE, Municoy S, Álvarez-Echazú MI, et al., 2022, The 3D bioprinted scaffolds for wound healing. Pharmaceutics, 14(2): 464.
126. Kotlarz M, Ferreira AM, Gentile P, et al., 2022, Droplet-based bioprinting enables the fabrication of cell–hydrogel– microfibre composite tissue precursors. Bio-Des Manuf, 5: 512–528. https://doi.org/10.1007/s42242-022-00192-5
127. Ríos-Galacho M, Martínez-Moreno D, López-Ruiz E, et al., 2021, An overview on the manufacturing of functional and mature cellular skin substitutes. Tissue Eng Part B Rev, 28(5): 1035–1052. https://doi.org/10.1089/ten.teb.2021.0131
128. Bhardwaj N, Chouhan D, Mandal B, 2017, Tissue engineered skin and wound healing: Current strategies and future directions. Curr Pharm Des, 23(24): 3455–3482. https://doi.org/10.2174/1381612823666170526094606
129. Malekmohammadi S, Sedghi Aminabad N, Sabzi A, et al., 2021, Smart and biomimetic 3D and 4D printed composite hydrogels: Opportunities for different biomedical applications. Biomedicines, 9(11): 1537.
130. Zidarič T, Milojević M, Gradišnik L, et al., 2020, Polysaccharide-based bioink formulation for 3D bioprinting of an in vitro model of the human dermis. Nanomaterials, 10(4): 733.
131. Puertas-Bartolomé M, Włodarczyk-Biegun MK, del Campo A, et al., 2021, Development of bioactive catechol functionalized nanoparticles applicable for 3D bioprinting. Mater Sci Eng C, 131: 112515. https://doi.org/10.1016/j.msec.2021.112515
132. Sohn S, Buskirk MV, Buckenmeyer MJ, et al., 2020, Whole organ engineering: Approaches, challenges, and future directions. Appl Sci, 10(12): 4277.
133. Jin R, Cui Y, Chen H, et al., 2021, Three-dimensional bioprinting of a full-thickness functional skin model using acellular dermal matrix and gelatin methacrylamide bioink. Acta Biomater, 131: 248–261. https://doi.org/10.1016/j.actbio.2021.07.012
134. Pleguezuelos-Beltrán P, Gálvez-Martín P, Nieto-García D, et al., 2022, Advances in spray products for skin regeneration. Bioact Mater, 16: 187–203. https://doi.org/10.1016/j.bioactmat.2022.02.023
135. Phang SJ, Arumugam B, Kuppusamy UR, et al., 2021, A review of diabetic wound models—Novel insights into diabetic foot ulcer. J Tissue Eng Regen Med, 15(12): 1051–1068. https://doi.org/10.1002/term.3246
136. Manita PG, Garcia-Orue I, Santos-Vizcaino E, et al., 2021, 3D bioprinting of functional skin substitutes: From current achievements to future goals. Pharmaceuticals, 14(4): 362.
137. Kant RJ, Coulombe KLK, 2018, Integrated approaches to spatiotemporally directing angiogenesis in host and engineered tissues. Acta Biomater, 69: 42–62. https://doi.org/10.1016/j.actbio.2018.01.017
138. Douillet C, Nicodeme M, Hermant L, et al., 2022, From local to global matrix organization by fibroblasts: A 4D laser-assisted bioprinting approach. Biofabrication, 14(2): 025006.
139. Glover K, Stratakos ACh, Varadi A, et al., 2021, 3D scaffolds in the treatment of diabetic foot ulcers: New trends vs conventional approaches. Int J Pharm, 599: 120423. https://doi.org/10.1016/j.ijpharm.2021.120423
140. Hendriks J, Willem Visser C, Henke S, et al., 2015, Optimizing cell viability in droplet-based cell deposition. Sci Rep, 5(1): 11304. https://doi.org/10.1038/srep11304
141. Das S, Das D, 2021, Rational design of peptide-based smart hydrogels for therapeutic applications. Front Chem, 9: 770102.
142. Jahanshahi M, Hamdi D, Godau B, et al., 2020, An engineered infected epidermis model for in vitro study of the skin’s pro-inflammatory response. Micromachines, 11(2): 227.
143. Zhao W, Chen H, Zhang Y, et al., 2022, Adaptive multi-degree-of-freedom in situ bioprinting robot for hair-follicle-inclusive skin repair: A preliminary study conducted in mice. Bioeng Transl Med, n/a(n/a): e10303. https://doi.org/10.1002/btm2.10303
144. Tasoglu S, Demirci U, 2013, Bioprinting for stem cell research. Trends Biotechnol, 31(1): 10–19. https://doi.org/10.1016/j.tibtech.2012.10.005
145. Echave MC, Hernáez-Moya R, Iturriaga L, et al., 2019, Recent advances in gelatin-based therapeutics. Expert Opin Biol Ther, 19(8): 773–779. https://doi.org/10.1080/14712598.2019.1610383
146. Zhou F, Hong Y, Liang R, et al., 2020, Rapid printing of bio-inspired 3D tissue constructs for skin regeneration. Biomaterials, 258: 120287. https://doi.org/10.1016/j.biomaterials.2020.120287
147. Kong B, Liu R, Guo J, et al., 2023, Tailoring micro/nano-fibers for biomedical applications. Bioact Mater, 19: 328–347. https://doi.org/10.1016/j.bioactmat.2022.04.016
148. Xu J, Zheng S, Hu X, et al., 2020, Advances in the research of bioinks based on natural collagen, polysaccharide and their derivatives for skin 3D bioprinting. Polymers, 12(6): 1237
.
149. Kogelenberg SV, Yue Z, Dinoro JN, et al., 2018, Three-dimensional printing and cell therapy for wound repair. Adv Wound Care, 7(5): 145–156. https://doi.org/10.1089/wound.2017.0752
150. Khoda AB, Koc B, 2012, Designing controllable porosity for multifunctional deformable tissue scaffolds. J Med Devices, 6(3): 031003. https://doi.org/10.1115/1.4007009
151. Kim SH, Hong H, Ajiteru O, et al., 2021, 3D bioprinted silk fibroin hydrogels for tissue engineering. Nat Protoc, 16(12): 5484–5532. https://doi.org/10.1038/s41596-021-00622-1
152. Turner PR, Murray E, McAdam CJ, et al., 2020, Peptide chitosan/dextran core/shell vascularized 3D constructs for wound healing. ACS Appl Mater Interfaces, 12(29): 32328–32339. https://doi.org/10.1021/acsami.0c07212
153. Johnson AP, Sabu C, Nivitha KP, et al., 2022, Bioinspired and biomimetic micro- and nanostructures in biomedicine. J Controlled Release, 343: 724–754. https://doi.org/10.1016/j.jconrel.2022.02.013
154. Ding H, Chang RC, 2018, Simulating image-guided in situ bioprinting of a skin graft onto a phantom burn wound bed. Addit Manuf, 22: 708–719. https://doi.org/10.1016/j.addma.2018.06.022
155. Piola B, Sabbatini M, Gino S, et al., 2022, 3D bioprinting of gelatin–xanthan gum composite hydrogels for growth of human skin cells. Int J Mol Sci, 23(1): 539.
156. Kathawala MH, Ng WL, Liu D, et al., 2019, Healing of chronic wounds: An update of recent developments and future possibilities. Tissue Eng Part B Rev, 25(5): 429–444. https://doi.org/10.1089/ten.teb.2019.0019
157. Chocarro-Wrona C, López-Ruiz E, Perán M, et al., 2019, Therapeutic strategies for skin regeneration based on biomedical substitutes. J Eur Acad Dermatol Venereol, 33(3):484–496. https://doi.org/10.1111/jdv.15391