Multifunctional 3D platforms for rapid hemostasis and wound healing: Structural and functional prospects at biointerfaces
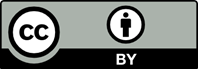
Fabrication of multifunctional hemostats is indispensable against chronic blood loss and accelerated wound healing. Various hemostatic materials that aid wound repair or rapid tissue regeneration has been developed in the last 5 years. This review provides an overview of the three-dimensional (3D) hemostatic platforms designed through the latest technologies like electrospinning, 3D printing, and lithography, solely or in combination, for application in rapid wound healing. We critically discuss the pivotal role of micro/nano-3D topography and biomaterial properties in mediating rapid blood clots and healing at the hemostat-biointerface. We also highlight the advantages and limitations of the designed 3D hemostats. We anticipate that this review will guide the fabrication of smart hemostats of the future for tissue engineering applications.
1. Phillips R, Friberg M, Lantz Cronqvist M, et al., 2020, Visual blood loss estimation accuracy: Directions for future research based on a systematic literature review. Proceedings of the human factors and ergonomics society annual meeting: Sage Publications, Los Angeles, CA, 1411–1415.
2. Granville-Chapman J, Jacobs N, Midwinter M, 2011, Prehospital haemostatic dressings: A systematic review. Injury, 42: 447–459.
3. Du X, Wu L, Yan H, et al., Micro channelled alkylated chitosan sponge to treat noncompressible hemorrhages and facilitate wound healing. Nat Commun, 12: 4733.
4. Zheng DW, Hong S, Xu L, et al., 2018, Hierarchical micro-/nanostructures from human hair for biomedical applications. Adv Mater, 30: e1800836.
5. He J, Liang Y, Shi M, et al., 2020, Anti-oxidant electroactive and antibacterial nanofibrous wound dressings based on poly(ε-caprolactone)/quaternized chitosan-graft-polyaniline for fullthickness skin wound healing. J Chem Eng, 385: 123464.
6. Nouri-Goushki M, Angeloni L, Modaresifar K, et al., 2021, 3D-printed submicron patterns reveal the interrelation between cell adhesion, cell mechanics, and osteogenesis. ACS Appl Mater Interfaces, 13: 33767–33781.
7. Xie X, Li D, Chen Y, et al., 2021, Conjugate electro spun 3D gelatin nanofiber sponge for rapid hemostasis. Adv Healthc Mater, 10, e2100918.
8. Wang K, Dong R, Tang J, et al., 2021, Exosomes laden self-healing injectable hydrogel enhances diabetic wound healing via regulating macrophage polarization to accelerate angiogenesis. J Chem Eng, 430: 132664.
9. Wang Z, Agrawal P, Zhang YS, 2021, Nanotechnologies and nanomaterials in 3D (Bio)printing toward bone regeneration. Adv NanoBio Res, 1: 2100035.
10. Sun X, Bai Y, Zhai H, et al., 2019, Devising micro/nanoarchitectures in multi-channel nerve conduits towards a pro-regenerative matrix for the repair of spinal cord injury. Acta Biomater, 86: 194–206.
11. Li M, Liang Y, Liang Y, et al., 2022, Injectable stretchable self-healing dual dynamic network hydrogel as adhesive anti-oxidant wound dressing for photothermal clearance of bacteria and promoting wound healing of MRSA infected motion wounds. J Chem Eng, 427: 132039.
12. Xie C, Ye J, Liang R, et al., 2021, Advanced strategies of biomimetic tissue-engineered grafts for bone regeneration. Adv Healthc Mater, 10: e2100408.
13. Nam S, Mooney D, 2021, Polymeric tissue adhesives. Chem Rev, 121:11336–11384.
14. Wan X, Liu Z, Li L, 2021, Manipulation of stem cells fates: The master and multifaceted roles of biophysical cues of biomaterials. Adv Funct Mater, 31: 2010626.
15. Mao AS, Özkalea B, Shah NJ, et al., 2019, Programmable microencapsulation for enhanced mesenchymal stem cell persistence and immunomodulation. PNAS, 116: 15392–15397.
16. Jia X, Minami K, Uto K, et al., 2020, Adaptive liquid interfacially assembled protein nanosheets for guiding mesenchymal stem cell fate. Adv Mater, 32: e1905942.
17. Ma Y, Jiang L, Hu J, et al., 2020, Multifunctional 3D micro nanostructures fabricated through temporally shaped femtosecond laser processing for preventing thrombosis and bacterial infection. ACS Appl Mater Interfaces, 12: 17155–17166.
18. Mariano A, Lubrano C, Bruno U, et al., 2021, Advances in cell-conductive polymer biointerfaces and role of the plasma membrane. Chem Rev, 122: 4552–4580.
19. Guo B, Dong R, Liang Y, et al., 2021, Haemostatic materials for wound healing applications. Nat Rev Chem, 5: 773–791.
20. Sakurai Y, Hardy ET, Ahn B, et al., 2018, A micro engineered vascularized bleeding model that integrates the principal components of hemostasis. Nat Commun, 9: 509.
21. Wilgus TA, Roy S, McDaniel JC, 2013, Neutrophils and wound repair: Positive actions and negative reactions. Adv Wound Care, 2: 379–388.
22. Davies LC, Jenkins SJ, Allen JE, et al., 2013, Tissue-resident macrophages. Nat Immun, 14: 986–995.
23. Ansell DM, Izeta A, 2015, Pericytes in wound healing: Friend or foe? Exp Dermatol, 24: 833–834.
24. Armulik A, Genové G, Betsholtz C, 2011, Pericytes: Developmental, physiological, and pathological perspectives, problems, and promises. Dev Cell, 21: 193–215.
25. Crisan M, Yap S, Casteilla L, et al., 2008, A perivascular origin for mesenchymal stem cells in multiple human organs. Cell Stem Cell, 3: 301–313.
26. Asahara T, Murohara T, Sullivan A, et al., 1997, Isolation of putative progenitor endothelial cells for angiogenesis. Science, 275: 964–966.
27. Ceradini DJ, Kulkarni AR, Callaghan MJ, et al., 2004, Progenitor cell trafficking is regulated by hypoxic gradients through HIF-1 induction of SDF-1. Nat Med, 10: 858–864.
28. Kosaraju R, Rennert RC, Maan ZN, et al., 2016, Adipose derived stem cell-seeded hydrogels increase endogenous progenitor cell recruitment and neovascularization in wounds. Tissue Eng Part A, 22: 295–305.
29. Tepper OM, Capla JM, Galiano RD, et al., 2005, Adult vasculogenesis occurs through in situ recruitment, proliferation, and tubulization of circulating bone marrow–derived cells. Blood, 105: 1068–1077.
30. Donati G, Rognoni E, Hiratsuka T, et al., 2017, Wounding induces dedifferentiation of epidermal Gata6+ cells and acquisition of stem cell properties. Nat Cell Biol, 19: 603–613.
31. Hsu C, Hutt E, Bloomfield DM, et al., 2021, Factor XI inhibition to uncouple thrombosis from hemostasis: JACC review topic of the week. J Am Coll Cardiol, 78: 625–631.
32. Liu J, Solanki A, White MJ, et al., 2022, Therapeutic use of α2-antiplasmin as an antifibrinolytic and hemostatic agent in surgery and regenerative medicine. NPJ Regen Med, 7: 1–11.
33. Neubauer K, Zieger B, 2021, Endothelial cells and coagulation. Cell Tissue Res, 7: 1–8.
34. Law LA, Graham DK, Di Paola J, 2018, GAS6/TAM pathway signaling in hemostasis and thrombosis. Front Med, 5: 137.
35. Weisel J, Litvinov R, 2019, Red blood cells: The forgotten player in hemostasis and thrombosis. J Thromb Haemost, 17: 271–282.
36. Rodrigues M, Kosaric N, Bonham CA, et al., 2019, Wound healing: A cellular perspective. Physiol Rev, 99: 665–706.
37. Abdi MM, Razalli RL, Tahir PM, et al., 2019, Optimized fabrication of newly cholesterol biosensor based on nanocellulose. Int J Biol Macromol, 126: 1213–1222.
38. Freedman BR, Uzun O, Luna NMM, et al., 2021, Degradable and removable tough adhesive hydrogels. Adv Mater, 33: e2008553.
39. Feng Y, Luo X, Wu F, et al., 2022, Systematic studies on blood coagulation mechanisms of halloysite nanotubes-coated PET dressing as superior topical hemostatic agent. J Chem Eng, 428: 132049.
40. Long M, Zhang Y, Huang P, et al., 2018, Emerging nanoclay composite for effective hemostasis. Adv Funct Mater, 28: 1704452.
41. Cui Y, Huang Z, Lei L, et al., 2021, Robust hemostatic bandages based on nanoclay electrospun membranes. Nat Commun, 12: 5922.
42. Zhang Y, Li C, Zhang W, et al., 2021, 3D-printed NIR responsive shape memory polyurethane/magnesium scaffolds with tight-contact for robust bone regeneration. Bioact Mater, 16–218–231.
43. Liang Y, He J, Guo B, 2021, Functional hydrogels as wound dressing to enhance wound healing. ACS Nano, 15: 12687–12722.
44. Bhusal A, Dogan E, Nguyen HA, et al., 2021, Multi-material digital light processing bioprinting of hydrogel-based microfluidic chips. Biofabrication, 14: 1–14.
45. Lei C, Xie Z, Wu K, et al., 2021, Controlled vertically aligned structures in polymer composites: Natural inspiration, structural processing, and functional application. Adv Mater, 33: e2103495.
46. Chaudhuri O, Cooper-White J, Janmey PA, et al., 2020, Effects of extracellular matrix viscoelasticity on cellular behaviour. Nature, 584: 535–546.
47. McHugh KJ, Nguyen TD, Linehan AR, et al., 2017, Fabrication of fillable microparticles and other complex 3D microstructures. Science, 357: 1138–1142.
48. Chen S, Carlson MA, Zhang YS, et al., 2018, Fabrication of injectable and superelastic nanofiber rectangle matrices (“peanuts”) and their potential applications in hemostasis. Biomaterials, 179: 46–59.
49. Chen R, Zhao C, Chen Z, et al., 2022, A bionic cellulose nanofiber-based nanocage wound dressing for NIR-triggered multiple synergistic therapy of tumors and infected wounds. Biomaterials, 281: 121330.
50. Jeong GJ, Im GB, Lee TJ, et al., 2021, Development of a stem cell spheroid‐laden patch with high retention at skin wound site. Bioeng Transl Med, 2022: 1–10.
51. Shi Z, Lan G, Hu E, et al., 2022, Targeted delivery of hemostats to complex bleeding wounds with magnetic guidance for instant hemostasis. J Chem Eng, 427: 130916.
52. Xi G, Liu W, Chen M, et al., 2019, Polysaccharide-based lotus seedpod surface-like porous microsphere with precise and controllable micromorphology for ultrarapid hemostasis. ACS Appl Mater Interfaces, 11: 46558–46571.
53. Huang X, Fu Q, Deng Y, et al., 2021, Surface roughness of silk fibroin/alginate microspheres for rapid hemostasis in vitro and in vivo. Carbohydr Polym, 253: 117256.
54. Liu X, Moradi MA, Bus T, et al., 2021, Flower-like colloidal particles through precipitation polymerization of redox-responsive liquid crystals. Angew Chem, 60: 27026–27030.
55. Lv C, Li L, Jiao Z, et al., 2021, Improved hemostatic effects by Fe (3+) modified biomimetic PLLA cotton-like mat via sodium alginate grafted with dopamine. Bioact Mater, 6: 2346–2359.
56. Wu J, Guo J, Linghu C, et al., 2021, Rapid digital light 3D printing enabled by a soft and deformable hydrogel separation interface. Nat Commun, 12: 6070.
57. Peng X, Xu X, Deng Y, et al., 2021, Ultrafast self‐gelling and wet adhesive powder for acute hemostasis and wound healing. Adv Funct Mater, 31: 2102583.
58. Collins MN, Ren G, Young K, et al., 2021, Scaffold fabrication technologies and structure/function properties in bone tissue engineering. Adv Funct Mater, 31: 2010609.
59. Mohamed E, Fitzgerald A, Tsuzuki T, 2021, The role of nanoscale structures in the development of topical hemostatic agents. Mater Today Nano, 16: 100137.
60. Karavasili C, Fatouros DG, 2021, Self-assembling peptides as vectors for local drug delivery and tissue engineering applications. Adv Drug Deliv Rev, 174: 387–405.
61. Udangawa RN, Mikael PE, Mancinelli C, et al., 2019, Novel cellulose-halloysite hemostatic nanocomposite fibers with a dramatic reduction in human plasma coagulation time. ACS Appl Mater Interfaces, 11: 15447–15456.
62. Sun X, Jia P, Zhang H, et al., 2021, Green regenerative hydrogel wound dressing functionalized by natural drug‐food homologous small molecule self‐assembled nanospheres. Adv Funct Mater, 32: 2106572.
63. Yuk H, Wu J, Sarrafian TL, et al., 2021, Rapid and coagulation-independent haemostatic sealing by a paste inspired by barnacle glue. Nat Biomed Eng, 5: 1131–1142.
64. Yang Y, Liang Y, Chen J, et al., 2022, Mussel-inspired adhesive antioxidant antibacterial hemostatic composite hydrogel wound dressing via photo-polymerization for infected skin wound healing. Bioact Mater, 8: 341–354.
65. Ruiz-Esparza GU, Wang X, Zhang X, et al., 2021, Nanoengineered shear-thinning hydrogel barrier for preventing postoperative abdominal adhesions, Micro Nano Lett, 13: 212.
66. Pourshahrestani S, Zeimaran E, Kadri NA, et al, 2020, Polymeric hydrogel systems as emerging biomaterial platforms to enable hemostasis and wound healing. Adv Healthc Mater, 9: e2000905.
67. Zhang F, King MW, 2020, Biodegradable polymers as the pivotal player in the design of tissue engineering scaffolds. Adv Healthc Mater, 9:e1901358.
68. Wang XX, Liu Q, Sui JX, et al., 2019, Recent advances in hemostasis at the nanoscale. Adv Healthc Mater, 8: e1900823.
69. Hao R, Peng X, Zhang Y, et al., 2020, Rapid hemostasis resulting from the synergism of self-assembling short peptide and O-carboxymethyl chitosan. ACS Appl Mater Interfaces, 12: 55574–55583.
70. Vassey MJ, Figueredo GP, Scurr DJ, et al., 2020, Immune modulation by design: Using topography to control human monocyte attachment and macrophage differentiation. Adv Sci, 7: 1903392.
71. Zhou K, Chigan D, Xu L, et al., 2021, Anti-sandwich structured photo-electronic wound dressing for highly efficient bacterial infection therapy. Small, 17: e2101858.
72. Chen M, Dong R, Zhang J, et al., 2021, Nanoscale metalorganic frameworks that are both fluorescent and hollow for self-indicating drug delivery. ACS Appl Mater Interfaces, 13: 18554–18562.
73. Gong, Celi N, Zhang D, 2022, Magnetic biohybrid microrobot multimers based on chlorella cells for enhanced targeted drug delivery. ACS Appl Mater Interfaces, 14: 6320–6330.
74. Chen J, Caserto JS, Ang I, et al., 2021, An adhesive and resilient hydrogel for the sealing and treatment of gastric perforation. Bioact Mater, 14: 52–60.
75. Wang J, Li Y, Nie G, 2021, Multifunctional biomolecule nanostructures for cancer therapy. Nat Rev Mater, 6: 1–18.
76. Daikuara LY, Chen X, Yue Z, et al., 2021, 3D bioprinting constructs to facilitate skin regeneration. Adv Funct Mater, 32: 2105080.
77. Li D, Yang Z, Zhao X, et al., 2022, Osteo immunomodulatory injectable lithium-heparin hydrogel with microspheres/TGF-β1 delivery promotes M2 macrophage polarization and osteogenesis for guided bone regeneration. J Chem Eng, 435: 134991.
78. Nasajpour A, Mandla S, Shree S, et al., 2017, Nanostructured fibrous membranes with rose spike-like architecture. Nano Lett, 17:6235–6240.
79. Liang Y, Li Z, Huang Y, et al., 2021, Dual-dynamic-bond cross-linked antibacterial adhesive hydrogel sealants with on-demand removability for post-wound-closure and infected wound healing. ACS Nano, 15: 7078–7093.
80. Hu Y, Cao S, Chen J, et al., 2020, Biomimetic fabrication of icariin loaded nano hydroxyapatite reinforced bioactive porous scaffolds for bone regeneration. J Chem Eng, 394: 124895.
81. Liverani L, Liguori A, Zezza P, et al., 2021, Nanocomposite electrospun fibers of poly(ε-caprolactone)/bioactive glass with shape memory properties. Bioact Mater, 11: 230–239.
82. Adu-Berchie K, Mooney DJ, 2020, Biomaterials as local niches for immunomodulation. Acc Chem Res, 53: 1749–1760.
83. Li Y, Niu F, Zhao X, et al., 2021, Nonwetting nanostructured hemostatic material for bleeding control with minimal adhesion. Adv Mater Interfaces, 8: 2101412.
84. Yan Y, Yan J, Gong X, et al., 2021, All-in-one asymmetric microsupercapacitor with negative Poisson’s ratio structure based on versatile electrospun nanofibers. J Chem Eng, 133580.
85. Guo Y, Huang J, Fang Y, et al., 2022, 1D, 2D, and 3D scaffolds promoting angiogenesis for enhanced wound healing. J Chem Eng, 437: 134690.
86. Yang G, Kong H, Chen Y, et al., 2022, Recent advances in the hybridization of cellulose and carbon nanomaterials: Interactions, structural design, functional tailoring, and applications. Carbohydr Polym, 279: 118947.
87. Wang L, Zhou M, Xu T, et al., 2022, Multifunctional hydrogel as wound dressing for intelligent wound monitoring. J Chem Eng, 433: 134625.
88. Yang H, Liang Y, Wang J, et al., 2021, Multifunctional wound dressing for rapid hemostasis, bacterial infection monitoring and photodynamic antibacterial therapy. Acta Biomater, 135: 179–190.
89. Khodaei A, Jahanmard F, Madaah Hosseini HR, et al., 2021, Controlled temperature-mediated curcumin release from magneto-thermal nanocarriers to kill bone tumors. Bioact Mater, 11: 107–117.
90. Zheng L, Wang Q, Zhang YS, et al., 2021, A hemostatic sponge derived from skin secretion of Andrias davidianus and nanocellulose. J Chem Eng, 416: 129136.
91. Li Y, Zhu J, Cheng H, et al., 2021, Developments of advanced electrospinning techniques: A critical review. Adv Mater Technol, 6: 2100410.
92. Zheng Y, Ma W, Yang Z, et al., 2022, An ultralong hydroxyapatite nanowire aerogel for rapid hemostasis and wound healing. J Chem Eng, 430: 132912.
93. Zhang X, Lei Y, Li C, et al., 2021, Superhydrophobic and multifunctional aerogel enabled by bioinspired salvinia leaflike structure. Adv Funct Mater, 32: 2110830.
94. Chen Y, Shafiq M, Liu M, et al., 2020, Advanced fabrication for electrospun three-dimensional nanofiber aerogels and scaffolds. Bioact Mater, 5: 963–979.
95. Tafreshi OA, Mosanenzadeh SG, Karamikamkar S, et al., 2022, A review on multifunctional aerogel fibers: Processing, fabrication, functionalization, and applications. Mater Today Chem, 23: 100736.
96. Zhang X, Li Y, He D, et al., 2021, An effective strategy for preparing macroporous and self-healing bioactive hydrogels for cell delivery and wound healing. J Chem Eng, 425: 130677.
97. Wan Y, Han J, Cheng F, et al., 2020, Green preparation of hierarchically structured hemostatic epoxy-amine sponge. J Chem Eng, 397: 125445.
98. Zhang K, Bai X, Yuan Z, et al., 2019, Layered nanofiber sponge with an improved capacity for promoting blood coagulation and wound healing. Biomater, 204: 70–79.
99. Jiang J, Oguzlu H, Jiang F, 2021, 3D printing of lightweight, super-strong yet flexible all-cellulose structure. J Chem Eng, 405: 126668.
100. Hu Y, Wu B, Xiong Y, et al., 2021, Cryogenic 3D printed hydrogel scaffolds loading exosomes accelerate diabetic wound healing. J Chem Eng, 426: 130634.
101. Zhang H, Cong Y, Osi AR, et al., 2020, Direct 3D printed biomimetic scaffolds based on hydrogel microparticles for cell spheroid growth. Adv Funct Mater, 30: 1910573.
102. Zou F, Wang Y, Zheng Y, et al., 2022, A novel bioactive polyurethane with controlled degradation and L-Arg release used as strong adhesive tissue patch for hemostasis and promoting wound healing. Bioact Mater, 17: 471–487.
103. Biranje SS, Sun J, Cheng L, et al., 2022, Development of cellulose nanofibril/casein-based 3D composite hemostasis scaffold for potential wound-healing application. ACS Appl Mater Interfaces, 14: 3792–3808.
104. Pourchet LJ, Thepot A, Albouy M, et al., 2017, Human skin 3D bioprinting using scaffold-free approach. Adv Healthc Mater, 6: 1–8.
105. Taghizadeh M, Taghizadeh A, Yazdi MK, et al., 2022, Chitosan-based inks for 3D printing and bioprinting. Green Chem, 24: 62–101.
106. Lavrador P, Esteves MR, Gaspar VM, et al., 2020, Stimuli responsive nanocomposite hydrogels for biomedical applications. Adv Funct Mater, 31: 2005941.
107. Yuan H, Chen L, Hong FF, 2020, A biodegradable antibacterial nanocomposite based on oxidized bacterial nanocellulose for rapid hemostasis and wound healing. ACS Appl Mater Interfaces, 12: 3382–3392.
108. Placone JK, Engler AJ, 2018, Recent advances in extrusion based 3d printing for biomedical applications. Adv Healthc Mater, 7: e1701161.
109. Davoodi E, Montazerian H, Zhianmanesh M, et al., 2021, Template-enabled biofabrication of thick three-dimensional tissues with patterned perfusable macro-channels. Adv Healthc Mater, 2021: e2102123.
110. Weems AC, Arno MC, Yu W, et al., 2021, 4D polycarbonates via stereolithography as scaffolds for soft tissue repair. Nat Commun, 12: 3771.
111. Cosola A, Sangermano M, Terenziani D, et al., 2021, dlp 3D-printing of shape memory polymers stabilized by thermos reversible hydrogen bonding interactions. Appl Mater Today, 23: 101060.
112. Kurtuldu F, Mutlu N, Boccaccini AR, et al., 2022, Gallium containing bioactive materials: A review of anticancer, antibacterial, and osteogenic properties. Bioact Mater, 17: 125–146.
113. Pourshahrestani S, Zeimaran E, Kadri NA, et al., 2017, Potency and cytotoxicity of a novel gallium-containing mesoporous bioactive glass/chitosan composite scaffold as hemostatic agents. ACS Appl Mater Interfaces, 9: 31381–31392.
114. Galliger Z, Vogt CD, Panoskaltsis-Mortari A, 2019, 3D bioprinting for lungs and hollow organs. Transl Res, 211: 19–34.
115. Sun Z, Lu Y, Zhao Q, et al., 2022, A new stereolithographic 3D printing strategy for hydrogels with a large mechanical tunability and self-weldability. Addit Manuf, 50: 102563.
116. Palaganas NB, Mangadlao JD, de Leon ACC, et al., 2017, 3D printing of photocurable cellulose nanocrystal composite for fabrication of complex architectures via stereolithography. ACS Appl Mater Interfaces, 9: 34314–34324.
117. Quan H, Zhang T, Xu H, et al., 2020, Photo-curing 3d printing technique and its challenges. Bioact Mater, 5: 110–115.
118. Zhou F, Hong Y, Liang R, et al., 2020, Rapid printing of bio-inspired 3D tissue constructs for skin regeneration. Biomaterials, 258: 120287.
119. Vera D, García-Díaz M, Torras N, et al., Engineering tissue barrier models on hydrogel microfluidic platforms. ACS Appl Mater Interfaces, 13: 13920–13933.
120. Rezaei FS, Sharifianjazi F, Esmaeilkhanian A, et al., Chitosan films and scaffolds for regenerative medicine applications: A review. Carbohydr Polym, 273: 118631.
121. Illath K, Kar S, Gupta P, et al., 2021, Microfluidic nanomaterials: From synthesis to biomedical applications. Biomaterials, 2021: 121247.
122. Xu Z, Tian W, Wen C, et al., 2022, Cellulose-based cryogel microspheres with nanoporous and controllable wrinkled morphologies for rapid hemostasis. Nano Letters, 22: 6350–6358.
123. Wang M, Hu J, Ou Y, et al., 2022, Shape-recoverable hyaluronic acid–waterborne polyurethane hybrid cryogel accelerates hemostasis and wound healing. ACS Appl Mater Interfaces, 14: 17093–17108.
124. Huang Y, Zhao X, Wang C, et al., 2022, High-strength antibacterial composite cryogel for lethal noncompressible hemorrhage hemostasis: Synergistic physical hemostasis and chemical hemostasis. J Chem Eng, 427: 131977.
125. Teng L, Xia K, Qian T, et al., 2022, Shape-recoverable macroporous nanocomposite hydrogels created via ice templating polymerization for noncompressible wound hemorrhage. ACS Biomater Sci Eng, 8: 2076–2087.
126. Zhu Y, Liu H, Qin S, et al., 2022, Antibacterial sericin cryogels promote hemostasis by facilitating the activation of coagulation pathway and platelets. Adv Healthc Mater, 2022: 2102717.
127. Zhang Y, Wang Y, Chen L, et al., An injectable antibacterial chitosan-based cryogel with high absorbency and rapid shape recovery for noncompressible hemorrhage and wound healing. Biomaterials, 285: 121546.
128. Bai Q, Teng L, Zhang X, et al., 2022, Multifunctional single component polypeptide hydrogels: The gelation mechanism, superior biocompatibility, high performance hemostasis, and scarless wound healing. Adv Healthc Mater, 11: 2101809.
129. Duan S, Wu R, Xiong Y-H, et al., 2022, Multifunctional antimicrobial materials: From rational design to biomedical applications. Prog Mater Sci, 125: 100887.
130. Liu J, Zhou X, Zhang Y, et al., 2022, Rapid hemostasis and excellent antibacterial cerium-containing mesoporous bioactive glass/chitosan composite sponge for hemostatic material. Mater Today Chem, 23: 100735.
131. Cao C, Yang N, Zhao Y, et al., 2021, Biodegradable hydrogel with thermo-response and hemostatic effect for photothermal enhanced anti-infective therapy. Nano Today, 39: 101165.
132. Choudhary M, Chhabra P, Tyagi A, et al., Scar free healing of full thickness diabetic wounds: A unique combination of silver nanoparticles as antimicrobial agent, calcium alginate nanoparticles as hemostatic agent, fresh blood as nutrient/growth factor supplier and chitosan as base matrix. Int J Biol Macromol, 178: 41–52.
133. Zheng Y, Pan N, Liu Y, et al., 2021, Novel porous chitosan/N-halamine structure with efficient antibacterial and hemostatic properties. Carbohydr Polym, 253: 117205.
134. Yin M, Wang Y, Zhang Y, et al., 2020, Novel quaternarized N-halamine chitosan and polyvinyl alcohol nanofibrous membranes as hemostatic materials with excellent antibacterial properties. Carbohydr Polym, 232: 115823.
135. Zhou J, Zhang H, Fareed MS, et al., 2022, An injectable peptide hydrogel constructed of natural antimicrobial peptide j-1 and adp shows anti-infection, hemostasis, and antiadhesion efficacy. ACS Nano, 16: 7636–7657.
136. Liang Y, Liang Y, Zhang H, et al., 2022, Antibacterial biomaterials for skin wound dressing. Asian J Pharm Sci, 17: 353–384.
137. Chen J, He J, Yang Y, et al., 2022, Antibacterial adhesive self-healing hydrogels to promote diabetic wound healing. Acta Biomater, 146: 119–130.
138. Yu R, Li M, Li Z, et al., 2022, Supramolecular thermos contracting adhesive hydrogel with self‐removability simultaneously enhancing noninvasive wound closure and mrsa‐infected wound healing. Adv Healthc Mater, 2022: 2102749.
139. Liang Y, Li M, Yang Y, et al., 2022, Ph/glucose dual responsive metformin release hydrogel dressings with adhesion and self-healing via dual-dynamic bonding for athletic diabetic foot wound healing. ACS Nano, 16: 3194–3207.
140. Wang Y, Wu Y, Long L, et al., 2021, Inflammation-responsive drug-loaded hydrogels with sequential hemostasis, antibacterial, and anti-inflammatory behavior for chronically infected diabetic wound treatment. ACS Appl Mater Interfaces,13: 33584–33599.
141. Yang L, Pijuan-Galito S, Rho HS, et al., 2021, High-throughput methods in the discovery and study of biomaterials and materiobiology. Chem Rev, 121: 4561–4677.
142. Khalil AS, Jaenisch R, Mooney DJ, 2020, Engineered tissuesand strategies to overcome challenges in drug development. Adv Drug Deliv Rev, 158: 116–139.
143. Sun L, Yu Y, Chen Z, et al., 2020, Biohybrid robotics with living cell actuation. Chem Soc Rev, 49: 4043–4069.
144. Kim K, Ryu Ji H, Koh M-Y, et al., Coagulopathy-independent, bioinspired hemostatic materials: A full research story from preclinical models to a human clinical trial. Sci Adv, 7: eabc9992.
145. Gonzalez-Pujana A, Vining KH, Zhang DKY, et al., 2020, Multifunctional biomimetic hydrogel systems to boost the immunomodulatory potential of mesenchymal stromal cells. Biomaterials, 257: 120266.
146. Ceylan H, Dogan NO, Yasa IC, et al., 2021, 3D printed personalized magnetic micromachines frompatient blood–derived biomaterials. Sci Adv, 7: eabh0273.
147. Geng H, Pan Yc, Zhang R, et al., 2021, Binding to amyloid‐β protein by photothermal blood‐brain barrier‐penetrating nanoparticles for inhibition and disaggregation of fibrillation. Adv Funct Mater, 31: 2102953.
148. García‐Astrain C, Lenzi E, Jimenez de Aberasturi D, et al., 2020, 3D‐printed biocompatible scaffolds with built‐in nanoplasmonic sensors. Adv Funct Mater, 30:2005407.
149. Zhang D, Chen Q, Shi C, et al., 2020, Dealing with the foreign‐body response to implanted biomaterials: Strategies and applications of new materials. Adv Funct Mater, 31: 2007226.
150. Balabiyev A, Podolnikova NP, Kilbourne JA, et al., 2021, Fibrin polymer on the surface of biomaterial implants drives the foreign body reaction. Biomaterials, 277: 121087.
151. Lin X, Li F, Bing Y, et al., 2021, Biocompatible multifunctional e-skins with excellent self-healing ability enabled by clean and scalable fabrication. Micro Nano Lett, 13: 200.
152. Kim JS, Hwang H, Lee D, et al., 2021, Electro spinnable, neutral coacervates for facile preparation of solid phenolic bioadhesives. ACS Appl Mater Interfaces, 13: 37989–37996.
153. Li J, Celiz AD, Yang J, et al., 2017, Tough adhesives for diverse wet surfaces. Science, 357: 378–381.
154. Wang Z, Wang Y, Yan J, et al., 2021, Pharmaceutical electrospinning and 3D printing scaffold design for bone regeneration. Adv Drug Deliv Rev, 174: 504–534.
155. Chen J, Dai S, Liu L, et al., 2021, Photo-functionalized TiO2 nanotubes decorated with multifunctional Ag nanoparticles for enhanced vascular biocompatibility. Bioact Mater, 6: 45–54.
156. Cheng F, Liu C, Li H, et al., 2018, Carbon nanotube-modified oxidized regenerated cellulose gauzes for hemostatic applications. Carbohydr Polym, 183: 246–253.
157. Leonhardt EE, Kang N, Hamad MA, et al., 2019, Absorbable hemostatic hydrogels comprising composites of sacrificial templates and honeycomb-like nanofibrous mats of chitosan. Nat Commun, 10: 2307.
158. Yan J, Wang Y, Li X, et al., 2021, A bionic nano-band-aid constructed by the three-stage self-assembly of peptides for rapid liver hemostasis. Nano Lett, 21: 7166–7174.
159. Madruga LYC, Popat KC, Balaban RC, et al., 2021, Enhanced blood coagulation and antibacterial activities of carboxymethyl-kappa-carrageenan-containing nanofibers. Carbohydr Polym, 273: 118541.
160. Tavakoli S, Kharaziha M, Nemati S, et al., 2021, Nanocomposite hydrogel based on carrageenan-coated starch/cellulose nanofibers as a hemorrhage control material. Carbohydr Polym, 251: 117013.
161. Mohamed E, Coupland LA, Crispin PJ, et al., 2021, Nonoxidized cellulose nanofibers as a topical hemostat: In vitro thromboelastometry studies of structure vs function. Carbohydr Polym, 265: 118043.
162. Liu R, Dai L, Si C, et al., 2018, Antibacterial and hemostatic hydrogel via nanocomposite from cellulose nanofibers. Carbohydr Polym, 195: 63–70.
163. Cheng F, Liu C, Wei X, et al., 2017, Preparation and characterization of 2,2,6,6-tetramethylpiperidine-1-oxyl (tempo)-oxidized cellulose nanocrystal/alginate biodegradable composite dressing for hemostasis applications. ACS Sustain Chem Eng, 5: 3819–3828.
164. Lou P, Liu S, Wang Y, et al., 2021, Injectable self-assembling peptide nanofiber hydrogel as a bioactive 3D platform to promote chronic wound tissue regeneration. Acta Biomater, 135: 100–112.
165. Adeli-Sardou M, Yaghoobi MM, Torkzadeh-Mahani M, et al., 2019, Controlled release of lawsone from polycaprolactone/gelatin electrospun nano fibers for skin tissue regeneration. Int J Biol Macromol, 124: 478–491.
166. Liu S, Wang Y, Ming X, et al., 2021, High-speed blow spinning of neat graphene fibrous materials. Nano Lett, 21: 5116–5125.
167. McCarthy A, Saldana L, McGoldrick D, et al., 2021, Largescale synthesis of compressible and re-expandable three dimensional nanofiber matrices. Nano Select, 2: 1566–1579.
168. Jiang Q, Luo B, Wu Z, et al., 2021, Corn stalk/AgNPs modified chitin composite hemostatic sponge with high absorbency, rapid shape recovery and promoting wound healing ability. J Chem Eng, 421: 129815.
169. Clasky AJ, Watchorn JD, Chen PZ, et al., 2021, From prevention to diagnosis and treatment: Biomedical applications of metal nanoparticle-hydrogel composites. Acta Biomater, 122: 1–25.
170. Zadeh Mehrizi T, Eshghi P, 2021, Investigation of the effect of nanoparticles on platelet storage duration 2010–2020. Int Nano Lett, 12:15–45.
171. Kottana RK, Maurizi L, Schnoor B, et al., 2021, Anti-platelet effect induced by iron oxide nanoparticles: Correlation with conformational change in fibrinogen. Small, 17: e2004945.
172. Zhao Y, Liu R, Fan Y, et al., 2021, Self-sealing hemostatic and antibacterial needles by polyphenol-assisted surface self-assembly of multifunctional nanoparticles. J Chem Eng, 425: 130621.
173. Tao B, Lin C, Yuan Z, et al., 2021, Near infrared light triggered on-demand Cur release from Gel-PDA@Cur composite hydrogel for antibacterial wound healing. J Chem Eng, 403: 126182.
174. Yang Z, Fu X, Zhou L, et al., 2021, Chem-inspired synthesis of injectable metal–organic hydrogels for programmable drug carriers, hemostasis and synergistic cancer treatment. J Chem Eng, 423: 130202.
175. Albadawi H, Altun I, Hu J, et al., 2020, Nanocomposite hydrogel with tantalum microparticles for rapidendovascular hemostasis. Adv Sci, 8: 2003327.
176. da Silva D, Kaduri M, Poley M, et al., 2018, Biocompatibility, biodegradation and excretion of polylactic acid (PLA) in medical implants and theranostic systems. J Chem Eng, 340: 9–14.
177. Song C, Zhang X, Wang L, et al., 2019, An injectable conductive three-dimensional elastic network by tangled surgical-suture spring for heart repair. ACS Nano, 13: 14122–14137.
178. Chiong JA, Tran H, Lin Y, et al., 2021, Integrating emerging polymer chemistries for the advancement of recyclable, biodegradable, and biocompatible electronics. Adv Sci, 8: e2101233.
179. Ashammakhi N, Hernandez AL, Unluturk BD, et al., 2021, Biodegradable implantable sensors: Materials design, fabrication, and applications. Adv Funct Mater, 31: 2104149.
180. Bal-Ozturk A, Cecen B, Avci-Adali M, et al., 2021, Tissue adhesives: From research to clinical translation. Nano Today, 36: 101049.
181. Ma C, Sun J, Li B, et al., 2021, Ultra-strong bio-glue from genetically engineered polypeptides. Nat Commun, 12: 3613.
182. Li Y, Huang X, Xu Y, et al., 2022, A bio-inspired multifunctional soy protein-based material: From strong underwater adhesion to 3D printing. J Chem Eng, 430: 133017.
183. He J, Zhang Z, Yang Y, et al., 2021, Injectable self-healing adhesive ph-responsive hydrogels accelerate gastric hemostasis and wound healing. Micro Nano Lett, 13: 80.
184. Poventud-Fuentes I, Kwon KW, Seo J, et al., 2021, A human vascular injury-on-a-chip model of hemostasis. Small, 17: e2004889.
185. Kim KY, Ryu JH, Koh MY, et al., 2021, Coagulopathy independent, bioinspired hemostatic materials A full research story from preclinical models to a human clinical trial. Sci Adv, 7: eabc9992
186. Teng L, Shao Z, Bai Q, et al., 2021, Biomimetic glycopolypeptide hydrogels with tunable adhesion and microporous structure for fast hemostasis and highly efficient wound healing. Adv Funct Mater, 31: 2105628.
187. Faizullin DA, Valiullina YA, Salnikov VV, et al., 2021, Fibrinogen adsorption on the lipid surface as a factor of regulation of fibrin formation. Biophysics, 66: 70–76.
188. Zhou L, Zheng H, Liu Z, et al., 2021, Conductive antibacterial hemostatic multifunctional scaffolds based on ti3c2tx mxene nanosheets for promoting multidrug-resistant bacteria infected wound healing. ACS Nano, 15: 2468–2480.
189. Li Z, Zhao Y, Ouyang X, et al., 2022, Biomimetic hybrid hydrogel for hemostasis, adhesion prevention and promoting regeneration after partial liver resection. Bioact Mater, 11: 41–51.
190. Liang Y, Li M, Huang Y, et al., 2021, An integrated strategy for rapid hemostasis during tumor resection and prevention of postoperative tumor recurrence of hepatocellular carcinoma by antibacterial shape memory cryogel. Small, 17: e2101356.
191. Mahmoodzadeh A, Moghaddas J, Jarolmasjed S, et al., 2021, Biodegradable cellulose-based superabsorbent as potent hemostatic agent. J Chem Eng, 418:129252.
192. Wang Y, Zhao Y, Qiao L, et al., 2021, Cellulose fibers reinforced self-expanding porous composite with multiple hemostatic efficacy and shape adaptability for uncontrollable massive hemorrhage treatment. Bioact Mater, 6: 2089–2104.
193. Lu S, Zhang X, Tang Z, et al., 2021, Mussel-inspired blue light- activated cellulose-based adhesive hydrogel with fast gelation, rapid haemostasis and antibacterial property for wound healing. J Chem Eng, 417: 129329.
194. Peers S, Montembault A, Ladaviere C, 2022, Chitosan hydrogels incorporating colloids for sustained drug delivery. Carbohydr Polym, 275: 118689.
195. Kalantari K, Mostafavi E, Saleh B, et al., 2020, Chitosan/pva hydrogels incorporated with green synthesized cerium oxide nanoparticles for wound healing applications. Eur Polym J, 134: 109853.
196. Asghari F, Rabiei Faradonbeh D, Malekshahi ZV, et al., 2021, Hybrid PCL/chitosan-PEO nanofibrous scaffolds incorporated with A. euchroma extract for skin tissue engineering application. Carbohydr Polym, 278: 118926.
197. Jung HY, Le Thi P, HwangBo KH, et al., 2021, Tunable and high tissue adhesive properties of injectable chitosan based hydrogels through polymer architecture modulation. Carbohydr Polym, 261: 117810.
198. Xia L, Wang S, Jiang Z, et al., 2021, Hemostatic performance of chitosan-based hydrogel and its study on biodistribution and biodegradability in rats. Carbohydr Polym, 264: 117965.
199. Vakilian S, Jamshidi-Adegani F, Al Yahmadi A, et al., 2021, A competitive nature-derived multilayered scaffold based on chitosan and alginate, for full-thickness wound healing. Carbohydr Polym, 262: 117921.
200. Montazerian H, Baidya A, Haghniaz R, et al., 2021, Stretchable and bioadhesive gelatin methacryloyl-based hydrogels enabled by in situ dopamine polymerization. ACS Appl Mater Interfaces, 13: 40290–40301.
201. Contessotto P, Orbanić D, Da Costa M, et al., 2021, Elastin-like recombinamers-based hydrogel modulates post-ischemic remodeling in a non-transmural myocardial infarction in sheep. Sci Transl Med, 13: eaaz5380.
202. Nelson DW, Gilbert RJ, 2021, Extracellular matrix-mimetic hydrogels for treating neural tissue injury: A focus on fibrin, hyaluronic acid, and elastin-like polypeptide hydrogels. Adv Healthc Mater, 10: e2101329.
203. Bai Q, Teng L, Zhang X, et al., 2021, Multifunctional single-component polypeptide hydrogels: The gelation mechanism, superior biocompatibility, high performance hemostasis, and scarless wound healing. Adv Healthc Mater, 11: e2101809.
204. Bonito V, Koch SE, Krebber MM, et al., 2021, Distinct effects of heparin and interleukin-4 functionalization on macrophage polarization and in situ arterial tissue regeneration using resorbable supramolecular vascular grafts in rats. Adv Healthc Mater, 10: e2101103.
205. Bae S, DiBalsi MJ, Meilinger N, et al., 2018, Heparin-eluting electrospun nanofiber yarns for antithrombotic vascular sutures. ACS Appl Mater Interfaces, 10: 8426–8435.
206. Hettiaratchi Marian H, Krishnan L, Rouse T, et al., 2020, Heparin-mediated delivery of bone morphogenetic protein-2 improves spatial localization of bone regeneration. Sci Adv, 6: eaay1240.
207. Kucharz K, Kristensen K, Johnsen KB, et al., 2021, Post-capillary venules are the key locus for transcytosis-mediated brain delivery of therapeutic nanoparticles. Nat Commun, 12: 4121.
208. Harris AR, Wallace GG, 2017, Organic electrodes and communications with excitable cells. Adv Funct Mater, 28: 1700587.
209. Wang K, Frewin CL, Esrafilzadeh D, et al., 2019, High performance graphene-fiber-based neural recording microelectrodes. Adv Mater, 31: e1805867.
210. Maisha N, Rubenstein M, Bieberich CJ, et al., 2021, Getting to the core of it all: nanocapsules to mitigate infusion reactions can promote hemostasis and be a platform for intravenous therapies. Nano Lett, 21: 9069–9076.
211. Yang X, Wang C, Liu Y, et al., 2021, Inherent antibacterial and instant swelling ε-poly-lysine/poly(ethylene glycol) diglycidyl ether superabsorbent for rapid hemostasis and bacterially infected wound healing. ACS Appl Mater Interfaces, 13: 36709–36721.
212. Wendels S, Averous L, 2021, Biobased polyurethanes for biomedical applications. Bioact Mater, 6: 1083–1106.
213. Xiao M, Yao Y, Fan C, et al., 2021, Multiple H-bonding chain extender-based polyurethane: Ultrastiffness, hot-melt adhesion, and 3D printing finger orthosis. J Chem Eng, 433: 133260.
214. Jiang C, Zhang L, Yang Q, et al., 2021, Self-healing polyurethaneelastomer with mechanical tunability for multiple biomedical applications in vivo. Nat Commun, 12: 4395.
215. Zhang Z, Zhang Y, Li W, et al., 2021, Curcumin/Fe-SiO2 nano composites with multi-synergistic effects for scar inhibition and hair follicle regeneration during burn wound healing. Appl Mater Today, 23: 101065.
216. Wang Y, Ying T, Li J, et al., 2020, Hierarchical micro/nanofibrous scaffolds incorporated with curcumin and zinc ion eutectic metal organic frameworks for enhanced diabetic wound healing via anti-oxidant and anti-inflammatory activities. J Chem Eng, 402: 126273.
217. Liu J, Chen Z, Wang J, et al., 2018, Encapsulation of curcumin nanoparticles with mmp9-responsive and thermos sensitive hydrogel improves diabetic wound healing. ACS Appl Mater Interfaces, 10: 16315–16326.
218. Fahimirad S, Abtahi H, Satei P, et al., 2021, Wound healing performance of PCL/chitosan based electrospun nanofiber electro sprayed with curcumin loaded chitosan nanoparticles. Carbohydr Polym, 259: 117640.
219. Rao BR, Kumar R, Haque S, et al., 2021, Ag2[fe(cn)5no]- fabricated hydrophobic cotton as a potential wound healing dressing: An in vivo approach. ACS Appl Mater Interfaces, 13: 10689–10704.
220. Zhang F, Yang H, Yang Y, et al., 2021, Stretchable and biocompatible bovine serum albumin fibrous films supported silver for accelerated bacteria-infected wound healing. J Chem Eng, 417: 129145.
221. Xiang J, Zhu R, Lang S, et al., 2021, Mussel-inspired immobilization of zwitterionic silver nanoparticles toward antibacterial cotton gauze for promoting wound healing. J Chem Eng, 409: 128291.
222. Chen X, Li H, Qiao X, et al., 2021, Agarose oligosaccharide silver nanoparticle-antimicrobial peptide-composite for wound dressing. Carbohydr Polym, 269: 118258.
223. Kim JW, Mahapatra C, Hong JY, et al., 2017, Functional recovery of contused spinal cord in rat with the injection of optimal-dosed cerium oxide nanoparticles. Adv Sci, 4: 1700034.
224. Zhang M, Zhai X, Ma T, et al., 2021, Multifunctional cerium doped carbon dots nanoplatform and its applications for wound healing. J Chem Eng, 423: 130301.e1900256.
225. Ma X, Cheng Y, Jian H, et al., 2019, Hollow, rough, and nitric oxide-releasing cerium oxide nanoparticles for promotingmultiple stages of wound healing. Adv Healthc Mater, 8:
226. Fan L, Wang W, Wang Z, et al., 2021, Gold nanoparticles enhance antibody effect through direct cancer cell cytotoxicity by differential regulation of phagocytosis. Nat Commun, 12: 6371.
227. Zhu GH, Azharuddin M, Islam R, et al., 2021, Innate immune invisible ultrasmall gold nanoparticles—Framework for synthesis and evaluation. ACS Appl Mater Interfaces, 13: 23410–23422.
228. Hao F, Liu QS, Chen X, et al., 2019, Exploring the heterogeneity of nanoparticles in their interactions with plasma coagulation factor xii. ACS Nano, 13: 1990–2003.
229. Sakoda M, Kaneko M, Ohta S, et al., 2018, Injectable hemostat composed of a polyphosphate-conjugated hyaluronan hydrogel. Biomacromolecules, 19: 3280–3290.
230. Sundaram MN, Mony U, Varma PK, et al., 2021, Vasoconstrictor and coagulation activator entrapped chitosan based composite hydrogel for rapid bleeding control. Carbohydr Polym, 258:117634.
231. Sun C, Yue P, Chen R, et al., 2022, Chitin-glucan composite sponge hemostat with rapid shape-memory from Pleurotuseryngii for puncture wound. Carbohydr Polym, 291:119553.
232. Leonhardt EE, Kang N, Hamad MA, et al., 2019, Absorbable hemostatic hydrogels comprising composites of sacrificial templates and honeycomb-like nanofibrous mats of chitosan. Nat Commun, 10:1–9.
233. Borges-Vilches J, Figueroa T, Guajardo S, et al., 2022, Novel and effective hemostats based on graphene oxide-polymer aerogels: In vitro and in vivo evaluation. Biomater Adv, 139:213007.
234. de Moraes FM, Philippi JV, Belle F, et al., 2022, Lotacarrageenan/xyloglucan/serine powders loaded with tranexamic acid for simultaneously hemostatic, antibacterial, and antioxidant performance. Biomater Adv, 137: 212805.