PVDF/AgNP/MXene composites-based near-field electrospun fiber with enhanced piezoelectric performance for self-powered wearable sensors
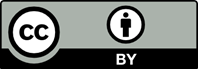
MXenes, as highly electronegative and conductive two-dimensional nanomaterials, are extensively studied for their use in sensors and flexible electronics. In this study, near-field electrospinning was used to prepare a new poly(vinylidene difluoride) (PVDF)/Ag nanoparticle (AgNP)/MXene composite nanofiber film as a self-powered flexible human motion-sensing device. The composite film displayed highly piezoelectric properties with the presence of MXene. Scanning electron microscopy, X-ray diffraction, and Fourier transform infrared spectroscopy revealed that the intercalated MXene in the composite nanofibers was evenly spread out, which not only prevented the aggregation of MXene but also enabled the composite materials to form self-reduced AgNPs. The prepared PVDF/AgNP/MXene fibers displayed exceptional stability and excellent output performance, enabling their use for energy harvesting and powering light-emitting diodes. The doping of MXene/AgNPs increased the electrical conductivity of the PVDF material, improved its piezoelectric properties, and enhanced the piezoelectric constant of PVDF piezoelectric fibers, thereby allowing the production of flexible, sustainable, wearable, and self-powered electrical devices.
1. Xue B, Xie H, Zhao J, et al., 2022, Flexible piezoresistive pressure sensor based on electrospun rough polyurethane nanofibers film for human motion monitoring. Nanomaterials, 12(4): 723. https://doi.org/10.3390/nano12040723
2. Mohapatra Dand Subudhi B, 2022, Development of a cost effective iot-based weather monitoring system. IEEE Consum Electron Mag, 11: 81–86. https://doi.org/10.1109/MCE.2021.3136833
3. Sengupta D, Kamat AM, Smit Q, et al., 2022, Piezoresistive 3D graphene-PDMS spongy pressure sensors for IoT enabled wearables and smart products. Flex Print Electron, 7. https://doi.org/10.1088/2058-8585/ac4d0e
4. Yang X, Liu G, Guo Q, et al., 2022, Triboelectric sensor array for internet of things based smart traffic monitoring and management system. Nano Energy, 92: 106757. https://doi.org/10.1016/j.nanoen.2021.106757
5. Xiao P, Zhou W, Liang Y, et al., 2022, Biomimetic skins enable strain‐perception‐strengthening soft morphing. Adv Funct Mater: 2201812. https://doi.org/10.1002/adfm.202201812
6. He J, Xiao P, Lu W, et al., 2019, A Universal high accuracy wearable pulse monitoring system via high sensitivity and large linearity graphene pressure sensor. Nano Energy, 59: 422–433. https://doi.org/10.1016/j.nanoen.2019.02.036
7. He J, Xiao P, Shi J, et al., 2018, High performance humidity fluctuation sensor for wearable devices via a bioinspired atomic-precise tunable graphene-polymer heterogeneous sensing junction. Chem Mater, 30(13): 4343–4354 https://doi.org/10.1021/acs.chemmater.8b01587
8. Wang S, Xiao P, Liang Y, et al., 2018, Network cracks based wearable strain sensors for subtle and large strain detection of human motions. J Mater Chem C, 6(19): 5140–5147. https://doi.org/10.1039/C8TC00433A
9. Pusty Mand Shirage PM, 2022, Insights and perspectives on graphene-PVDF based nanocomposite materials for harvesting mechanical energy. J Alloys Compd: 164060. https://doi.org/10.1016/j.jallcom.2022.164060
10. Meng K, Xiao X, Wei W, et al., 2022, Wearable pressure sensors for pulse wave monitoring. Adv Mater, 34: e2109357. https://doi.org/10.1002/adma.202109357
11. Zeng X, Deng H-T, Wen D-L, et al., 2022, Wearable multi-functional sensing technology for healthcare smart detection. Micromachines, 13(2): 254. https://doi.org/10.3390/mi13020254
12. Lo L-W, Zhao J, Wan H, et al., 2022, A Soft sponge sensor for multimodal sensing and distinguishing of pressure, strain, and temperature. ACS Appl Mater Interfaces, 14(7): 9570–9578. https://doi.org/10.1021/acsami.1c21003
13. Dong K, Wu Z, Deng J, et al., 2018, A stretchable yarn embedded triboelectric nanogenerator as electronic skin for biomechanical energy harvesting and multifunctional pressure sensing. Adv Mater, 30(43): 1804944. https://doi.org/10.1002/adma.201804944
14. Zhu M, Chng SS, Cai W, et al., 2020, Piezoelectric polymer nanofibers for pressure sensors and their applications in human activity monitoring. RSC Adv, 10(37): 21887–21894. https://doi.org/10.1039/D0RA03293J
15. Hou X, Zhang S, Yu J, et al., 2020, Flexible piezoelectric nanofibers/polydimethylsiloxane‐based pressure sensor for self‐powered human motion monitoring. Energy Technol, 8(3): 1901242. https://doi.org/10.1002/ente.201901242
16. Stadlober B, Zirkl Mand Irimia-Vladu M, 2019, Route towards sustainable smart sensors: ferroelectric polyvinylidene fluoride-based materials and their integration in flexible electronics. Chem Soc Rev, 48(6): 1787–1825. https://doi.org/10.1039/C8CS00928G
17. Ribeiro C, Sencadas V, Correia DM, et al., 2015, Piezoelectric polymers as biomaterials for tissue engineering applications. Colloids Surf B, 136: 46–55. https://doi.org/10.1016/j.colsurfb.2015.08.043
18. Yu G-F, Yan X, Yu M, et al., 2016, Patterned, highly stretchable and conductive nanofibrous PANI/PVDF strain sensors based on electrospinning and in situ polymerization. Nanoscale, 8(5): 2944–2950. https://doi.org/10.1039/C5NR08618C
19. Zhou H, Zhang Y, Qiu Y, et al., 2020, Stretchable piezoelectric energy harvesters and self-powered sensors for wearable and implantable devices. Biosens, 168: 112569. https://doi.org/10.1016/j.bios.2020.112569
20. Chorsi MT, Curry E J, Chorsi HT, et al., 2019, Piezoelectric biomaterials for sensors and actuators. Adv Mater, 31(1): 1802084. https://doi.org/10.1002/adma.201802084
21. Safari Aand Akdogan E K, (eds) 2008, Piezoelectric and acoustic materials for transducer applications. Springer Science & Business Media, New York. https://doi.org/10.1007/978-0-387-76540-2
22. Cui C, Xue F, Hu W-J, et al., 2018, Two-dimensional materials with piezoelectric and ferroelectric functionalities. NPJ 2D MaterApp, 2(1): 1–14. https://doi.org/10.1038/s41699-018-0063-5
23. Kapat K, Shubhra QTH, Zhou M, et al., 2020, Piezoelectric nano‐biomaterials for biomedicine and tissue regeneration. Adv Funct Mater, 30(44): 1909045. https://doi.org/10.1002/adfm.201909045
24. Bowen CR, Kim H A, Weaver P M, et al., 2014, Piezoelectric and ferroelectric materials and structures for energy harvesting applications. Energy Environ Sci, 7(1): 25–44. https://doi.org/10.1039/C3EE42454E
25. Mias, S., & Camon, H. (2008). A review of active optical devices: II. Phase modulation. Journal of Micromechanics and Microengineering, 18(8), 083002. http://dx.doi.org/10.1088/0960-1317/18/8/083002
26. Tichý J, Erhart J, Kittinger E, et al., 2010, Fundamentals of piezoelectric sensorics: mechanical, dielectric, and thermodynamical properties of piezoelectric materials. Springer Science & Business Media, London. https://doi.org/10.1007/978-3-540-68427-5
27. Garcia-Sanchez F, Sáez Aand Dominguez J, 2005, Anisotropic and piezoelectric materials fracture analysis by BEM. Comput Struct, 83(10-11): 804–820. https://doi.org/10.1016/j.compstruc.2004.09.010
28. Kumar D, Chaturvedi Pand Jejurikar N, 2014, Piezoelectric energy harvester design and power conditioning. In 2014 IEEE Students’ Conference on Electrical, Electronics and Computer Science, IEEE, 1–6. https://doi.org/10.1109/SCEECS.2014.6804491
29. Liu Z, Li S, Zhu J, et al., 2022, Fabrication of β-phase-enriched PVDF sheets for self-powered piezoelectric sensing. ACS ApplMater Interfaces, 14(9): 11854–11863. https://doi.org/10.1021/acsami.2c01611
30. Li J, Zhou G, Hong Y, et al., 2022, Highly sensitive, flexible and wearable piezoelectric motion sensor based on PT promoted β-phase PVDF. Sens Actuator A Phys, 337: 113415. https://doi.org/10.1016/j.sna.2022.113415
31. Katsouras I, Asadi K, Li M, et al., 2016, The negative piezoelectric effect of the ferroelectric polymer poly (vinylidene fluoride). Nat mater, 15(1): 78–84. http://dx.doi.org/10.1038/nmat4423
32. Auliya RZ, Ooi PC, Sadri R, et al., 2021, Exploration of 2D Ti3C2 MXene for all solution processed piezoelectric nanogenerator applications. Sci Rep, 11(1): 1–13. https://doi.org/10.1038/s41598-021-96909-0
33. Chen J-J, Li Y, Zheng X-M, et al., 2018, Enhancement in electroactive crystalline phase and dielectric performance of novel PEG-graphene/PVDF composites. Appl Surf Sci, 448: 320–330. https://doi.org/10.1016/j.apsusc.2018.04.144
34. Fan FR, Tang Wand Wang ZL, 2016, Flexible nanogenerators for energy harvesting and self‐powered electronics. Adv Mater, 28(22): 4283–4305. https://doi.org/10.1002/adma.201504299
35. Tang C-W, Li B, Sun L, et al., 2012, The effects of nanofillers, stretching and recrystallization on microstructure, phase transformation and dielectric properties in PVDF nanocomposites. Eur Polym J, 48(6): 1062–1072. https://doi.org/10.1016/j.eurpolymj.2012.04.002
36. Arshad AN, Wahid MHM, Rusop M, et al., 2019, Dielectric and structural properties of poly (vinylidene fluoride) (PVDF) and poly (vinylidene fluoride-trifluoroethylene) (PVDF-TrFE) filled with magnesium oxide nanofillers. J Nanomater, 2019. https://doi.org/10.1155/2019/5961563
37. Jin Y, Xia Nand Gerhardt RA, 2016, Enhanced dielectric properties of polymer matrix composites with BaTiO3 and MWCNT hybrid fillers using simple phase separation. Nano Energy, 30: 407–416. https://doi.org/10.1016/j.nanoen.2016.10.033
38. Lu L, Ding W, Liu J, et al., 2020, Flexible PVDF based piezoelectric nanogenerators. Nano Energy, 78: 105251. https://doi.org/10.1016/j.nanoen.2020.105251
39. Teo W Eand Ramakrishna S, 2006, A review on electrospinning design and nanofibre assemblies. Nanotechnology, 17(14): R89. http://dx.doi.org/10.1088/0957-4484/17/14/R01
40. Liu ZH, Pan CT, Lin LW, et al., 2013, Direct-write PVDF nonwoven fiber fabric energy harvesters via the hollow cylindrical near-field electrospinning process. Smart Mater Struct, 23(2): 025003. http://dx.doi.org/10.1088/0964-1726/23/2/025003
41. Liu ZH, Pan CT, Lin LW, et al., 2013, Piezoelectric properties of PVDF/MWCNT nanofiber using near-field electrospinning. Sens Actuators A: Phys, 193: 13–24. https://doi.org/10.1016/j.sna.2013.01.007
42. Zaarour B, Zhu L, Huang C, et al., 2018, Fabrication of a polyvinylidene fluoride cactus-like nanofiber through one step electrospinning. RSC Adv, 8(74): 42353–42360. https://doi.org/10.1039/C8RA09257E
43. Pan C-T, Tsai K-C, Wang S-Y, et al., 2017, Development of piezoelectric fibers in smart patch by near-field electrospinning method with closed-loop motor control. Sens Mater, 29: 497–509. http://dx.doi.org/10.18494/SAM.2017.1532
44. Pan C-T, Yen C-K, Wang S-Y, et al., 2015, Near-field electrospinning enhances the energy harvesting of hollow PVDF piezoelectric fibers. RSC Adv, 5(103): 85073–85081. https://doi.org/10.1039/C5RA16604G
45. Pan C-T, Yen C-K, Wu H-C, et al., 2015, Significant piezoelectric and energy harvesting enhancement of poly (vinylidene fluoride)/polypeptide fiber composites prepared through near-field electrospinning. J Mater Chem A, 3(13): 6835–6843. https://doi.org/10.1039/C5TA00147A
46. Pan C-T, Yen C-K, Wang S-Y, et al., 2018, Energy harvester and cell proliferation from biocompatible PMLG nanofibers prepared using near-field electrospinning and electrospray technology. J Nanosci Nanotechnol, 18(1): 156–164. https://doi.org/10.1166/jnn.2018.14596
47. Takamatsu S, Takahata T, Muraki M, et al., 2010, Transparent conductive-polymer strain sensors for touch input sheets of flexible displays. J MicromechMicroeng, 20(7): 075017. http://dx.doi.org/10.1088/0960-1317/20/7/075017
48. Zou J, Yip H-L, Hau SK, et al., 2010, Metal grid/conducting polymer hybrid transparent electrode for inverted polymer solar cells. Appl phys lett, 96(20): 96. https://doi.org/10.1063/1.3394679
49. Emmott CJM, Urbina Aand Nelson J, 2012, Environmental and economic assessment of ITO-free electrodes for organic solar cells. Sol Energy Mater Sol Cells, 97: 14–21. https://doi.org/10.1016/j.solmat.2011.09.024
50. Wu C Mand Chou MH, 2016, Polymorphism, piezoelectricity and sound absorption of electrospun PVDF membranes with and without carbon nanotubes. Compos scitechnol, 127: 127–133. https://doi.org/10.1016/j.compscitech.2016.03.001
51. Sun J-G, Yang T-N, Wang C-Y, et al., 2018, A flexible transparent one-structure tribo-piezo-pyroelectric hybrid energy generator based on bio-inspired silver nanowires network for biomechanical energy harvesting and physiological monitoring. Nano Energy, 48: 383–390. https://doi.org/10.1016/j.nanoen.2018.03.071
52. Pusty M, Sinha Land Shirage PM, 2019, A flexible self-poled piezoelectric nanogenerator based on a rGO–Ag/PVDF nanocomposite. New J Chem, 43(1): 284–294. https://doi.org/10.1039/C8NJ04751K
53. Abolhasani MM, Shirvanimoghaddam Kand Naebe M, 2017, PVDF/graphene composite nanofibers with enhanced piezoelectric performance for development of robust nanogenerators. Compos Sci Technol, 138: 49–56. https://doi.org/10.1016/j.compscitech.2016.11.017
54. Afroj S, Tan S, Abdelkader AM, et al., 2020, Highly conductive, scalable, and machine washable graphene based E‐textiles for multifunctional wearable electronic applications. Adv Funct Mater, 30(23): 2000293. https://doi.org/10.1002/adfm.202000293
55. Ren M, Zhou Y, Wang Y, et al., 2019, Highly stretchable and durable strain sensor based on carbon nanotubes decorated thermoplastic polyurethane fibrous network with aligned wave-like structure. Chem Eng J, 360: 762–777. https://doi.org/10.1016/j.cej.2018.12.025
56. Wu L, Wang L, Guo Z, et al., 2019, Durable and multifunctional superhydrophobic coatings with excellent joule heating and electromagnetic interference shielding performance for flexible sensing electronics. ACS Appl Mater Interfaces, 11(37): 34338–34347. https://doi.org/10.1021/acsami.9b11895
57. Seyedin S, Uzun S, Levitt A, et al., 2020, MXene composite and coaxial fibers with high stretchability and conductivity for wearable strain sensing textiles. Adv Funct Mater, 30(12): 1910504. https://doi.org/10.1002/adfm.201910504
58. Park H, Brown PR, Bulović V, et al., 2012, Graphene as transparent conducting electrodes in organic photovoltaics: studies in graphene morphology, hole transporting layers, and counter electrodes. Nano Lett, 12(1): 133–140. https://doi.org/10.1021/nl2029859
59. Araki T, Jiu J, Nogi M, et al., 2014, Low haze transparent electrodes and highly conducting air dried films with ultra-long silver nanowires synthesized by one-step polyol method. Nano Res, 7(2): 236–245. https://doi.org/10.1007/s12274-013-0391-x
60. Pan C-T, Yen C-K, Lin L, et al., 2014, Energy harvesting with piezoelectric poly (γ-benzyl-l-glutamate) fibers prepared through cylindrical near-field electrospinning. RSC Adv, 4(41): 21563–21570. https://doi.org/10.1039/C4RA01452A
61. Kim GH, Hong S Mand Seo Y, 2009, Piezoelectric properties of poly (vinylidene fluoride) and carbon nanotube blends: β-phase development. Phys Chem Chem Phys, 11(44): 10506–10512. https://doi.org/10.1039/B912801H
62. Wu C Mand Chou MH, 2020, Acoustic-electric conversion and piezoelectric properties of electrospun polyvinylidene fluoride/silver nanofibrous membranes. Express Polym Lett, 14(2): 103–114. https://doi.org/10.3144/expresspolymlett.2020.10
63. Nthunya LN, Derese S, Gutierrez L, et al., 2019, Green synthesis of silver nanoparticles using one-pot and microwave-assisted methods and their subsequent embedment on PVDF nanofibre membranes for growth inhibition of mesophilic and thermophilic bacteria. New J Chem 43(10): 4168–4180. https://doi.org/10.1039/C8NJ06160B
64. Laroche G, Lafrance CP, Prud’homme RE, et al., 1998, Identification and quantification of the crystalline structures of poly (vinylidene fluoride) sutures by wide‐angle X‐ray scattering and differential scanning calorimetry. JBMRA, 39(2): 184–189. h t t p s : / / d o i . o r g / 1 0 . 1 0 0 2 / ( S I C I ) 1 0 9 7 - 4636(199802)39:2%3C184::AID JBM3%3E3.0.CO;2-L
65. Issa AA, Al-Maadeed MA, Luyt AS, et al., 2017, Physicomechanical, dielectric, and piezoelectric properties of PVDF electrospun mats containing silver nanoparticles. C, 3(4): 30. https://doi.org/10.3390/c3040030
66. Lou X, Chen J, Xiong Z, et al., 2021, Porosity design on conjugated microporous poly (aniline) S for exceptional mercury (II) removal. ACS Appl Mater Interfaces, 13: 51.
https://doi.org/10.1016/j.compscitech.2020.108360
67. Samy MM, Mohamed M Gand Kuo S-W, 2020, Pyrene functionalized tetraphenylethylene polybenzoxazine for dispersing single-walled carbon nanotubes and energy storage. Compos Sci Technol, 199: 108360. https://doi.org/10.1016/j.compscitech.2020.108360
68. Mohamed MG, Samy MM, Mansoure T H, et al., 2022, Dispersions of 1, 3, 4-oxadiazole-linked conjugated microporous polymers with carbon nanotubes as a high performance electrode for supercapacitors. ACS Appl Energy Mater, 5(3): 3677–3688. https://doi.org/10.1021/acsaem.2c00100
69. Lu F-H, Chang F-C, Mohamed M-G, et al., 2014, Conducting Ag/oligothiophene complex pastes through a simple quenching/chelation method. J Mater Chem C, 2(30): 6111–6118. https://doi.org/10.1039/C4TC00731J
70. Wang H, Wu Y, Zhang J, et al., 2015, Enhancement of the electrical properties of MXene Ti3C2 nanosheets by posttreatments of alkalization and calcination. Mater Lett, 160: 537–540. https://doi.org/10.1016/j.matlet.2015.08.046
71. Huang X, Wu P, 2020, A facile, high‐yield, and freeze and‐ thaw‐assisted approach to fabricate MXene with plentiful wrinkles and its application in on‐chip micro supercapacitors. Adv Funct Mater, 30(12): 1910048. https://doi.org/10.1002/adfm.201910048
72. Cai Y, Shen J, Ge G, et al., 2018, Stretchable Ti3C2T x MXene/carbon nanotube composite based strain sensor with ultrahigh sensitivity and tunable sensing range. ACS Nano, 12(1): 56–62. https://doi.org/10.1021/acsnano.7b06251
73. Mirkhani SA, Shayesteh Zeraati A, Aliabadian E, et al., 2019, High dielectric constant and low dielectric loss via poly (vinyl alcohol)/Ti3C2T x MXene nanocomposites. ACS Appl Mater Interfaces, 11(20): 18599–18608. https://doi.org/10.1021/acsami.9b00393
74. Xia Y, Mathis TS, Zhao M-Q, et al., 2018, Thickness independent capacitance of vertically aligned liquid crystalline MXenes. Nature, 557(7705): 409–412. https://doi.org/10.1038/s41586-018-0109-z
75. Ran J, Gao G, Li F-T, et al., 2017, Ti3C2 MXene co-catalyst on metal sulfide photo-absorbers for enhanced visible light photocatalytic hydrogen production. Nat commun, 8(1): 1–10. https://doi.org/10.1038/ncomms13907
76. Liu LX, Chen W, Zhang HB, et al., 2019, Flexible and multifunctional silk textiles with biomimetic leaf‐like MXene/silver nanowire nanostructures for electromagnetic interference shielding, humidity monitoring, and self derived hydrophobicity. Adv Funct Mater, 29(44): 1905197. https://doi.org/10.1002/adfm.201905197
77. Zhao X, Wang L-Y, Tang C-Y, et al., 2020, Smart Ti3C2T xMXene fabric with fast humidity response and joule heating for healthcare and medical therapy applications. ACS Nano, 14(7): 8793–8805.https://doi.org/10.1021/acsnano.0c03391
78. Yue Y, Liu N, Liu W, et al., 2018, 3D hybrid porous Mxene sponge network and its application in piezoresistive sensor. Nano Energy, 50: 79–87. https://doi.org/10.1016/j.nanoen.2018.05.020
79. Feng Y, Deng Q, Peng C, et al., 2018, An ultrahigh discharged energy density achieved in an inhomogeneous PVDF dielectric composite filled with 2D MXene nanosheets via interface engineering. J Mater Chem C, 6(48): 13283–13292. https://doi.org/10.1039/C8TC05180A
80. Feng Y, Deng Q, Peng C, et al., 2019, High dielectric and breakdown properties achieved in ternary BaTiO3/MXene/PVDF nanocomposites with low-concentration fillers from enhanced interface polarization. Ceram Int, 45(6): 7923–7930. https://doi.org/10.1016/j.ceramint.2019.01.104
81. Tu S, Jiang Q, Zhang X, et al., 2018, Large dielectric constant enhancement in MXene percolative polymer composites. ACS nano, 12(4): 3369–3377. https://doi.org/10.1021/acsnano.7b08895
82. Zhao Q, Yang L, Ma Y, et al., 2021, Highly sensitive, reliable and flexible pressure sensor based on piezoelectric PVDF hybrid film using MXene nanosheet reinforcement. J Alloys Compd, 886: 161069. https://doi.org/10.1016/j.jallcom.2021.161069
83. Wang S, Shao H-Q, Liu Y, et al., 2021, Boosting piezoelectric response of PVDF-TrFE via MXene for self-powered linear pressure sensor. Compos Sci Technol, 202: 108600. https://doi.org/10.1016/j.compscitech.2020.108600
84. Fan X, Ding Y, Liu Y, et al., 2019, Plasmonic Ti3C2T xMXene enables highly efficient photothermal conversion for healable and transparent wearable device. ACS nano, 13(7): 8124–8134. https://doi.org/10.1021/acsnano.9b03161
85. Zhai W, Wang C, Wang S, et al., 2021, Ultra-stretchable and multifunctional wearable electronics for superior electromagnetic interference shielding, electrical therapy and biomotion monitoring. J Mater Chem A, 9(11): 7238–7247. https://doi.org/10.1039/D0TA10991F
86. Saidi A, Gauvin C, Ladhari S, et al., 2021, Advanced functional materials for intelligent thermoregulation in personal protective equipment. Polymers, 13(21): 3711. https://doi.org/10.3390/polym13213711
87. Rana SMS, Rahman MT, Salauddin M, et al., 2021, Electrospun PVDF-TrFE/MXene nanofiber mat-based triboelectric nanogenerator for smart home appliances. ACS Appl Mater Interfaces, 13(4): 4955–4967. https://doi.org/10.1021/acsami.0c17512
88. Kim J, Jang M, Jeong G, et al., 2021, MXene-enhanced β-phase crystallization in ferroelectric porous composites for highly-sensitive dynamic force sensors. Nano Energy, 89: 106409. https://doi.org/10.1016/j.nanoen.2021.106409
89. Pan C-T, Dutt K, Yen C-K, et al., 2022, Characterization of piezoelectric properties of Ag-NPs doped PVDF nanocomposite fibres membrane prepared by near field electrospinning. Comb Chem High Throughput Screen, 25(4): 720–729. https://doi.org/10.2174/1386207324666210302100728
90. Yen C-K, Dutt K, Yao Y-S, et al., 2022, Development of flexible biceps tremors sensing chip of PVDF fibers with nano-silver particles by near-field electrospinning. Polymers, 14(2): 331. https://doi.org/10.3390/polym14020331
91. Chen W-w, An Z-l, He L-b, et al., 2015, Piezoelectric coefficients measurement for PVDF films with pneumatic pressure rig in a sole cavity. In 2015 Symposium on Piezoelectricity, Acoustic Waves, and Device Applications (SPAWDA), IEEE, 111–114. https://doi.org/10.1109/SPAWDA.2015.7364452
92. Huang Z, Zhang Q, Corkovic S, et al., 2006, Comparative measurements of piezoelectric coefficient of PZT films by berlincourt, interferometer, and vibrometer methods. IEEE Trans Ultrason Ferroelectr Freq Control, 53(12): 2287–2293. https://doi.org/10.1109/TUFFC.2006.175
93. Chang C, Tran VH, Wang J, et al., 2010, Direct-write piezoelectric polymeric nanogenerator with high energy conversion efficiency. Nano Lett, 10(2): 726–731. https://doi.org/10.1021/nl9040719
94. Yen CK, Tsao CH, Pan CT, et al., 2014, Electrospun PVDF fibers on bio-wings using multi-spinnerets. In The 9th IEEE International Conference on Nano/Micro Engineered and Molecular Systems (NEMS), IEEE, 609–613. https://doi.org/10.1109/NEMS.2014.6908885
95. Fu J, Hou Y, Zheng M, et al., 2020, Flexible piezoelectric energy harvester with extremely high power generation capability by sandwich structure design strategy. ACS Appl Mater Interfaces, 12(8): 9766–9774. https://doi.org/10.1021/acsami.9b21201
96. Singh HH, Singh Sand Khare N, 2018, Enhanced β‐phase in PVDF polymer nanocomposite and its application for nanogenerator. Polym Adv Technol, 29(1): 143–150. https://doi.org/10.1002/pat.4096
97. Shepelin NA, Sherrell PC, Skountzos EN, et al., 2021, Interfacial piezoelectric polarization locking in printable Ti3C2Tx MXene-fluoropolymer composites. Nat commun, 12(1): 1–11. https://doi.org/10.1038/s41467-021-23341-3
98. Jiang Z, Tan X, Huang Y, 2022, Piezoelectric effect enhanced photocatalysis in environmental remediation: State-ofthe- art techniques and future scenarios. Sci Total Environ, 806: 150924. https://doi.org/10.1016/j.scitotenv.2021.150924
99. Pan C-T, Tsai K-C, Wang S-Y, et al., 2017, Large area piezoelectric PVDF fibers fabricated by near-field electrospinning with multi-spinneret structures. Micromachines, 8(4): 97. https://doi.org/10.3390/mi8040097