Hybrid biomanufacturing systems applied in tissue regeneration
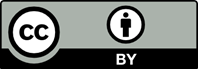
Scaffold-based approach is a developed strategy in biomanufacturing, which is based on the use of temporary scaffold that performs as a house of implanted cells for their attachment, proliferation, and differentiation. This strategy strongly depends on both materials and manufacturing processes. However, it is very difficult to meet all the requirements, such as biocompatibility, biodegradability, mechanical strength, and promotion of cell-adhesion, using only single material. At present, no single bioprinting technique can meet the requirements for tissue regeneration of all scales. Thus, multi-material and mixing-material scaffolds have been widely investigated. Challenges in terms of resolution, uniform cell distribution, and tissue formation are still the obstacles in the development of bioprinting technique. Hybrid bioprinting techniques have been developed to print scaffolds with improved properties in both mechanical and biological aspects for broad biomedical engineering applications. In this review, we introduce the basic multi-head bioprinters, semi-hybrid and fullyhybrid biomanufacturing systems, highlighting the modifications, the improved properties and the effect on the complex tissue regeneration applications.
1. Bártolo P, Chua C, Almeida H, et al., 2009, Biomanufacturing for tissue engineering: present and future trends. Virtual Phys Prototyp, 4: 203–216.
2. Xu Y, Chen C, Hellwarth PB, et al., 2019, Biomaterials for stem cell engineering and biomanufacturing. Bioact Mater, 4: 366–379. https://doi.org/10.1016/j.bioactmat.2019.11.002
3. Vyas C, Pereira R, Huang B, et al., 2017, Engineering the vasculature with additive manufacturing. Curr Opin Biomed Eng, 2: 1–13.
4. Mota C, Puppi D, Chiellini F, et al., 2015, Additive manufacturing techniques for the production of tissue engineering constructs. J Tissue Eng Regen Med, 9: 174–190. https://doi.org/10.1002/term.1635
5. Vyas C, Poologasundarampillai G, Hoyland J, et al., 2017, 3D printing of biocomposites for osteochondral tissue engineering. In: Biomedical Composites. 2nd ed. Elsevier, Netherlands. p.261–302.
6. Tavakoli S, Klar AS, 2021, Bioengineered skin substitutes: Advances and future trends. Appl Sci, 11: 1493.
7. Xue J, Singh S, Zhou Y, et al., 2022, A biodegradable 3D woven magnesium-based scaffold for orthopedic implants. Biofabrication, 14: 034107. https://doi.org/10.1088/1758-5090/ac73b8
8. Pillai S, Upadhyay A, Khayambashi P, et al., 2021, Dental 3D-printing: Transferring art from the laboratories to the clinics. Polymers (Basel), 13: 157. https://doi.org/10.3390/polym13010157
9. Lafuente-Merchan M, Ruiz-Alonso S, García-Villén F, et al., 2022, Progress in 3D bioprinting technology for osteochondral regeneration. Pharmaceutics, 14: 1578. https://doi.org/10.3390/pharmaceutics14081578
10. Alonzo M, El Khoury R, Nagiah N, et al., 2022, 3D Biofabrication of a cardiac tissue construct for sustained longevity and function. ACS Appl Mater Interfaces, 14: 21800–21813. https://doi.org/10.1021/acsami.1c23883
11. Li X, Liu B, Pei B, et al., 2020, Inkjet bioprinting of biomaterials. Chem Rev, 120: 10793–10833.
12. Yusupov V, Churbanov S, Churbanova E, et al., 2020, Laser induced forward transfer hydrogel printing: A defined route for highly controlled process. Int J Bioprint,6: 271. https://doi.org/10.18063/ijb.v6i3.271
13. Jiang T, Munguia-Lopez JG, Flores-Torres S, et al., Extrusion bioprinting of soft materials: An emerging technique for biological model fabrication. Appl Phys Rev, 6: 011310.
14. Ng WL, Lee JM, Zhou M, et al., Vat polymerization-based bioprinting-process, materials, applications and regulatory challenges. Biofabrication, 12: 022001. https://doi.org/10.1088/1758-5090/ab6034
15. Gauvin R, Chen YC, Lee JW, et al., 2012, Microfabrication of complex porous tissue engineering scaffolds using 3D projection stereolithography. Biomaterials, 33: 3824–3834. https://doi.org/10.1016/j.biomaterials.2012.01.048
16. Shen Y, Tang H, Huang X, et al., 2020, DLP printing photocurable chitosan to build bio-constructs for tissue engineering. Carbohydr Polym, 235: 115970. https://doi.org/10.1016/j.carbpol.2020.115970
17. Song J, Michas C, Chen CS, et al., 2020, From simple to architecturally complex hydrogel scaffolds for cell and tissue engineering applications: Opportunities presented by two photon polymerization. Adv Healthc Mater, 9: 1901217.
18. Kumar P, Ebbens S, Zhao X, 2021, Inkjet printing of mammalian cells-theory and applications. Bioprinting, 23: e00157.
19. Gudapati H, Dey M, Ozbolat I, 2016, A comprehensive review on droplet-based biomanufacturing: past, present and future. Biomaterials 102:20-42.
20. Li J, Chen M, Fan X, et al., 2016, Recent advances in biomanufacturing techniques: Approaches, applications and future prospects. J Transl Med, 14: 271.
21. Hölzl K, Lin S, Tytgat L, et al., 2016. Bioink properties before, during and after 3D biomanufacturing. Biofabrication, 8: 032002.
22. Li K, Liu JK, Chen WS, et al., 2018, Controllable printing droplets on demand by piezoelectric inkjet: Applications and methods. Microsyst Technol, 24: 879–889.
23. Saunders RE, Derby B, 2014, Inkjet printing biomaterials for tissue engineering: Biomanufacturing. Int Mater Rev, 59: 430–448.
24. Okubo N, Qureshi A, Dalgarno K, et al., 2019, Cost-effective microvalve-assisted bioprinter for tissue engineering. Biomanufacturing, 13: e00043.
25. Yan X, Zhang L, Sett S, et al., 2019, Droplet jumping: Effects of droplet size, surface structure, pinning, and liquid properties. ACS Nano, 13: 1309–1323.
26. Graham AD, Olof SN, Burke MJ, et al., 2017, High-resolution patterned cellular constructs by droplet-based 3D printing. Sci Rep, 7: 7004.
27. Negro A, Cherbuin T, Lutolf MP, 2018, 3D inkjet printing of complex, cell-laden hydrogel structures. Sci Rep, 8: 17099. https://doi.org/10.1038/s41598-018-35504-2
28. Odde DJ, Renn MJ, 1999, Laser-guided direct writing for applications in biotechnology. Trends Biotechnol, 17: 385–389. https://doi.org/10.1016/s0167-7799(99)01355-4
29. Sears NA, Seshadri DR, Dhavalikar PS, et al., 2016, A review of three-dimensional printing in tissue engineering. Tissue Eng Part B: Rev, 22: 298–310.
30. Kérourédan O, Rémy M, Oliveira H, et al., 2018, Laser assisted biomanufacturing of cells for tissue engineering. In: Laser Printing of Functional Materials: 3D Microfabrication, Electronics and Biomedicine. United States: Wiley. p349–373.
31. Martinez-Rivas A, González-Quijano GK, Proa-Coronado S, et al., 2017, Methods of micropatterning and manipulation of cells for biomedical applications. Micromachines (Basel), 8: 347. https://doi.org/10.3390/mi8120347
32. Guillemot F, Souquet A, Catros S, et al., 2010, High throughput laser printing of cells and biomaterials for tissue engineering. Acta biomater, 6: 2494–500. https://doi.org/10.1016/j.actbio.2009.09.029
33. Koch L, Deiwick A, Chichkov B, 2018, Laser-based cell printing. In: Ovsianikov, A., Yoo, J., Mironov, V. (eds) 3D Printing and Biofabrication. Springer, Champaign. p. 303-329. https://doi.org/10.1007/978-3-319-45444-3_11
34. Vinson BT, Sklare SC, Chrisey DB, 2017, Laser-based cell printing techniques for additive biomanufacturing. Curr Opin Biomed Eng, 2: 14–21.
35. Pereira RF, Bártolo PJ, 2015, 3D biomanufacturing of photocrosslinkable hydrogel constructs. J Appl Polym Sci, 132: 42458. 36. Bourget JM, Kérourédan O, Medina M, et al., 2016, Patterning of endothelial cells and mesenchymal stem cells by laser-assisted biomanufacturing to study cell migration. Biomed Res Int, 2016: 3569843. https://doi.org/10.1155/2016/3569843
37. Keriquel V, Oliveira H, Rémy M, et al., 2017, In situ printing of mesenchymal stromal cells, by laser-assisted biomanufacturing, for in vivo bone regeneration applications. Sci Rep, 7: 1778. https://doi.org/10.1038/s41598-017-01914-x
38. Zhang B, Luo Y, Ma L, et al., 2018, 3D biomanufacturing: An emerging technology full of opportunities and challenges. Bio Des Manuf, 1: 2–13.
39. Datta P, Ayan B, Ozbolat IT, 2017, Biomanufacturing for vascular and vascularized tissue biofabrication. Acta Biomater, 51: 1–20. https://doi.org/10.1016/j.actbio.2017.01.035
40. Mobaraki M, Ghaffari M, Yazdanpanah A, et al., 2020, Bioinks and bioprinting: A focused review. Bioprinting, 18: e00080.
41. Qi X, Pei P, Zhu M, et al., 2017, Three-dimensional printing of calcium sulfate and mesoporous bioactive glass scaffolds for improving bone regeneration in vitro and in vivo. Sci Rep, 7: 42556.
42. Jakus AE, Shah RN, 2017, Multi and mixed 3D‐printing of graphene‐hydroxyapatite hybrid materials for complex tissue engineering. J Biomed Mater Res A, 105: 274–283. https://doi.org/10.1002/jbm.a.35684
43. Kim MH, Lee YW, Jung WK, et al., 2019, Enhanced rheological behaviors of alginate hydrogels with carrageenan for extrusion-based biomanufacturing. J Mech Behav Biomed Mater, 98: 187–194. https://doi.org/10.1016/j.jmbbm.2019.06.014
44. Blaeser A, Million N, Campos DF, et al., 2016, Laser-based in situ embedding of metal nanoparticles into bioextruded alginate hydrogel tubes enhances human endothelial cell adhesion. Nano Res, 9: 3407–3427.
45. Bellini A, 2002, Fused Deposition of Ceramics: A Comprehensive Experimental, Analytical and Computational Study of Material Behavior, Fabrication Process and Equipment Design. Drexel University, United States.
46. Almeida H, Bartolo P, Mota C, et al., 2010, Processoe Equipamento de Fabrico Rápido por Bioextrusao. Portuguese Patent Application. p104247.
47. Liu, F, Wang, W, Mirihanage, W, et al., 2018, A plasma assisted bioextrusion system for tissue engineering. CIRP Ann, 67: 229–232.
48. Wang W, Caetano G, Ambler WS, et al., 2016, Enhancing the hydrophilicity and cell attachment of 3D printed pcl/graphene scaffolds for bone tissue engineering. Materials, 9: 992.
49. Boularaoui S, Al Hussein G, Khan KA, et al., An overview of extrusion-based bioprinting with a focus on induced shear stress and its effect on cell viability. Bioprinting, 20: e00093.
50. Zhang T, Zhao W, Xiahou Z, et al., Bioink design for extrusion-based bioprinting. Appl Mater Today, 25: 101227.
51. Chen DX, 2019, Extrusion biomanufacturing of scaffolds. In: Extrusion Biomanufacturing of Scaffolds for Tissue Engineering Applications. Springer, Berlin. p117–145.
52. Zhang AP, Qu X, Soman P, et al., 2012, Rapid fabrication of complex 3D extracellular microenvironments by dynamic optical projection stereolithography. Adv Mater, 24: 4266–4270.
53. Barraza B, Olate-Moya F, Montecinos G, et al., Superhydrophobic SLA 3D printed materials modified with nanoparticles biomimicking the hierarchical structure of a rice leaf. Sci Technol Adv Mater, 23: 300–321.
54. Raman R, Bhaduri B, Mir M, et al., High‐resolution projection micro stereolithography for patterning of neovasculature. Adv Healthc Mater, 5: 610–619. https://doi.org/10.1002/adhm.201500721
55. Lee SJ, Zhu W, Heyburn L, et al., 2016, Development of novel 3-D printed scaffolds with core-shell nanoparticles for nerve regeneration. IEEE Trans Biomed Eng, 64: 408–418. https://doi.org/10.1109/TBME.2016.2558493
56. Luo Y, Le Fer G, Dean D, et al., 2019, 3D printing of poly (propylene fumarate) oligomers: Evaluation of resin viscosity, printing characteristics and mechanical properties. Biomacromolecules, 20: 1699–1708.
57. Dong D, Su H, Li X, et al., 2022, Microstructures and mechanical properties of biphasic calcium phosphate bioceramics fabricated by SLA 3D printing. J Manuf Processes, 81: 433–443.
58. Admane P, Gupta AC, Jois P, et al., 2019, Direct 3D bioprinted full-thickness skin constructs recapitulate regulatory signaling pathways and physiology of human skin. Bioprinting, 15: e00051.
59. Norotte C, Marga FS, Niklason LE, et al., 2009, Scaffold free vascular tissue engineering using biomanufacturing. Biomaterials, 30: 5910–5917. https://doi.org/10.1016/j.biomaterials.2009.06.034
60. Byambaa B, Annabi N, Yue K, et al., 2017, Bioprinted osteogenic and vasculogenic patterns for engineering 3D bone tissue. Adv Healthc Mater, 6: 1700015. https://doi.org/10.1002/adhm.201700015
61. Langer EM, Allen-Petersen BL, King SM, et al, 2019, Modeling tumor phenotypes in vitro with three-dimensional biomanufacturing. Cell Rep, 26: 608–623. https://doi.org/10.1016/j.celrep.2018.12.090
62. Liu C, Xia Z, Han Z, et al., 2008, Novel 3D collagen scaffolds fabricated by indirect printing technique for tissue engineering. J Biomed Mater Res B Appl Biomater, 85: 519–528. https://doi.org/10.1002/jbm.b.30975
63. Liu L, Yan Y, Xiong Z, 2007, A novel poly (lactic-co-glycolic acid)-collagen hybrid scaffold fabricated via multi-nozzle low-temperature deposition. Virtual Rapid Manuf, 2019, 57–61.
64. Kim JY, Cho DW, 2009, Blended PCL/PLGA scaffold fabrication using multi-head deposition system. Microelectron Eng, 86: 1447–1450.
65. Shim JH, Kim JY, Park M, et al., 2011, Development of a hybrid scaffold with synthetic biomaterials and hydrogel using solid freeform fabrication technology. Biofabrication, 3: 034102. https://doi.org/10.1088/1758-5082/3/3/034102
66. Kim JY, Lee TJ, Cho DW, et al., 2010, Solid free-form fabrication-based PCL/HA scaffolds fabricated with a multi-head deposition system for bone tissue engineering. J Biomater sci Polym Ed, 21: 951–962. https://doi.org/10.1163/156856209X458380
67. Landers R, Mülhaupt R, 2000, Desktop manufacturing of complex objects, prototypes and biomedical scaffolds by means of computer‐assisted design combined with computer‐guided 3D plotting of polymers and reactive oligomers. Macromol Mater Eng, 282: 17–21.
68. You F, Wu X, Zhu N, et al., 2016, 3D printing of porous cell-laden hydrogel constructs for potential applications in cartilage tissue engineering. ACS Biomater Sci Eng, 2: 1200–1210.
69. Jain S, Fuoco T, Yassin MA, et al., 2019, Printability and critical insight into polymer properties during direct extrusion based 3D printing of medical grade polylactide and copolyesters. Biomacromolecules, 21: 388–396.
70. Wei L, Wu S, Kuss M, et al., 2019, 3D printing of silk fibroin based hybrid scaffold treated with platelet rich plasma for bone tissue engineering. Bioact Mater, 4: 256–260. https://doi.org/10.1016/j.bioactmat.2019.09.001
71. Kang HW, Lee SJ, Ko IK, et al., 2016, A 3D biomanufacturing system to produce human-scale tissue constructs with structural integrity. Nat Biotechnol, 34: 312–319.
72. Merceron TK, Burt M, Seol YJ, et al., 2015, A 3D bioprinted complex structure for engineering the muscle-tendon unit. Biofabrication, 7: 035003. https://doi.org/10.1088/1758-5090/7/3/035003
73. Kim JH, Seol YJ, Ko IK, et al., 2018, 3D bioprinted human skeletal muscle constructs for muscle function restoration. Sci Rep, 8: 1–15.
74. Lee J, Kim KE, Bang S, et al., 2017, A desktop multi-material 3D bio-printing system with open-source hardware and software. Int J Precision Eng Manuf, 18: 605–612.
75. Shim JH, Lee JS, Kim JY, et al., 2012, Biomanufacturing of a mechanically enhanced three-dimensional dual cellladen construct for osteochondral tissue engineering using a multi-head tissue/organ building system. J Micromech Microeng, 22: 085014.
76. Jung JW, Lee JS, Cho DW, 2016, Computer-aided multiple head 3D printing system for printing of heterogeneous organ/tissue constructs. Sci Rep, 6: 21685. https://doi.org/10.1038/srep21685
77. Ozbolat IT, Chen H, Yu Y, 2014, Development of ‘Multi-arm Bioprinter’for hybrid biofabrication of tissue engineering constructs. Robot Comput Integrated Manuf, 30: 295–304.
78. Yu Y, Moncal KK, Li J, et al., 2016, Three-dimensional biomanufacturing using self-assembling scalable scaffoldfree “tissue strands” as a new bioink. Sci Rep, 6: 28714.
79. Moncal KK, Heo DN, Godzik KP, et al., 2018, 3D printing of poly (ε-caprolactone)/poly (D, L-lactide-co-glycolide)/hydroxyapatite composite constructs for bone tissue engineering. J Mater Res, 33: 1972–1986.
80. Schuurman W, Khristov V, Pot MW, et al., 2011, Biomanufacturing of hybrid tissue constructs with tailorable mechanical properties. Biofabrication, 3: 021001.
81. Visser J, Peters B, Burger TJ, et al., 2013, Biofabrication of multi-material anatomically shaped tissue constructs. Biofabrication 5 (3):035007.
82. Raphael B, Khalil T, Workman VL, et al., 2017, 3D cell biomanufacturing of self-assembling peptide-based hydrogels. Mater Lett, 190: 103–106.
83. Zhuang P, Ng WL, An J, et al., 2019, Layer-by-layer ultraviolet assisted extrusion-based (UAE) biomanufacturing of hydrogel constructs with high aspect ratio for soft tissue engineering applications. PLoS One, 14: e0216776. https://doi.org/10.1371/journal.pone.0216776
84. Visscher DO, Bos EJ, Peeters M, et al., 2016, Cartilage tissue engineering: preventing tissue scaffold contraction using a 3D-printed polymeric cage. Tissue Eng Part C Methods, 22: 573–584. https://doi.org/10.1089/ten.TEC.2016.0073
85. Müller M, Öztürk E, Arlov Ø, et al., 2017, Alginate sulfatenano cellulose bioinks for cartilage biomanufacturing applications. Ann Biomed Eng, 45: 210–223. https://doi.org/10.1007/s10439-016-1704-5
86. Sultan S, Siqueira G, Zimmermann T, et al., 2017, 3D printing of nano-cellulosic biomaterials for medical applications. Curr Opin Biomed Eng, 2: 29–34.
87. Liu S, Li L, 2017, Ultrastretchable and self-healing double network hydrogel for 3D printing and strain sensor. ACS Appl Mater Interfaces, 9: 26429–26437.
88. Xu T, Binder KW, Albanna MZ, et al., 2012, Hybrid printing of mechanically and biologically improved constructs for cartilage tissue engineering applications. Biofabrication, 5: 015001.
89. Huang B, Aslan E, Jiang Z, Daskalakis E, et al., 2020. Engineered dual-scale poly (ε-caprolactone) scaffolds using 3D printing and rotational electrospinning for bone tissue regeneration. Addit Manufac, 36: 101452.
90. Liu F, Wang W, Hinduja S, et al., 2018, Hybrid additive manufacturing system for zonal plasma treated scaffolds. 3D Print Addit Manuf, 5: 205–213.