State-of-the-art techniques for promoting tissue regeneration: Combination of three-dimensional bioprinting and carbon nanomaterials
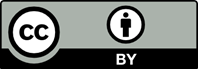
Biofabrication approaches, such as three-dimensional (3D) bioprinting of hydrogels, have recently garnered increasing attention, especially in the construction of 3D structures that mimic the complexity of tissues and organs with the capacity for cytocompatibility and post-printing cellular development. However, some printed gels show poor stability and maintain less shape fidelity if parameters such as polymer nature, viscosity, shear-thinning behavior, and crosslinking are affected. Therefore, researchers have incorporated various nanomaterials as bioactive fillers into polymeric hydrogels to address these limitations. Carbon-family nanomaterials (CFNs), hydroxyapatites, nanosilicates, and strontium carbonates have been incorporated into printed gels for application in various biomedical fields. In this review, following the compilation of research publications on CFNs-containing printable gels in various tissue engineering applications, we discuss the types of bioprinters, the prerequisites of bioink and biomaterial ink, as well as the progress and challenges of CFNs-containing printable gels in this field.
1. Gopinathan J, Noh I, 2018, Recent trends in bioinks for 3D printing. Biomater Res, 22:11.
2. Cha M, Jin YZ, Park JW, et al., 2021, Three-dimensional printed polylactic acid scaffold integrated with BMP-2 laden hydrogel for precise bone regeneration. Biomater Res, 25:35.
3. Murphy SV, Atala A, 2014, 3D bioprinting of tissues and organs. Nat Biotechnol, 32:773–785.
4. Guvendiren M, Molde J, Soares RMD, et al., 2016, Designing biomaterials for 3D printing. ACS Biomater Sci Eng, 2:1679–1693.
5. Kang MS, Kwon M, Lee SY, et al., 2022, In situ crosslinkable collagen-based hydrogels for 3D printing of dermis-mimetic constructs. ECS J Solid State Sci Technol, 11:045014.
6. Kang MS, Kwon M, Lee SH, et al., 2022, 3D printing of skin equivalents with hair follicle structures and epidermalpapillary-dermal layers using gelatin/hyaluronic acid hydrogels. Chem Asian J, 17: e202200620.
7. Ozbolat IT, Peng W, Ozbolat V, 2016, Application areas of 3D bioprinting. Drug Discov Today, 21:1257–1271.
8. Weng T, Zhang W, Xia Y, et al., 2021, 3D bioprinting for skin tissue engineering: Current status and perspectives. J Tissue Eng, 12:20417314211028574.
9. Gu Z, Fu J, Lin H, et al., 2020, Development of 3D bioprinting: From printing methods to biomedical applications. Asian J Pharm Sci, 15:529–557.
10. Gudapati H, Dey M, Ozbolat I, 2016, A comprehensive review on droplet-based bioprinting: Past, present and future. Biomaterials, 102:20–42.
11. Derby B, 2010, Inkjet printing of functional and structural materials: Fluid property requirements, feature stability, and resolution. Annu Rev Mater Res, 40:395–414.
12. Nakamura M, Kobayashi A, Takagi F, et al., 2005, Biocompatible inkjet printing technique for designed seeding of individual living cells. Tissue Eng, 11:1658–1666.
13. Binder KW, Zhao W, Aboushwareb T, et al., 2010, In situ bioprinting of the skin for burns. J Am Coll Surg, 211:S76.
14. Ozbolat IT, Hospodiuk M, 2016, Current advances and future perspectives in extrusion-based bioprinting. Biomaterials, 76:321–343.
15. Koch L, Gruene M, Unger C, et al., 2013, Laser assisted cell printing. Curr Pharm Biotechnol, 14:91–97.
16. Guillemot F, Souquet A, Catros S, et al., 2010, Laser-assisted cell printing: Principle, physical parameters versus cell fate and perspectives in tissue engineering. Nanomedicine, 5:507–515.
17. Koch L, Kuhn S, Sorg H, et al., 2010, Laser printing of skin cells and human stem cells. Tissue Eng Part C Methods, 16:847–854.
18. Serra P, Duocastella M, Fernández-Pradas JM, et al., 2009, Liquids microprinting through laser-induced forward transfer. Appl Surf Sci, 255:5342–5345.
19. Patrascioiu A, Fernández-Pradas JM, Palla-Papavlu A, et al., 2014, Laser-generated liquid microjets: Correlation between bubble dynamics and liquid ejection. Microfluid Nanofluid, 16:55–63.
20. Pan Y, Zeng L, 2019, Simulation and validation of droplet generation process for revealing three design constraints in electrohydrodynamic jet printing. Micromachines, 10:94.
21. Mkhize N, Bhaskaran H, 2022, Electrohydrodynamic jet printing: Introductory concepts and considerations. Small Sci, 2:2100073.
22. Lei Q, He J, Li D, 2019, Electrohydrodynamic 3D printing of layer-specifically oriented, multiscale conductive scaffolds for cardiac tissue engineering. Nanoscale, 11:15195–15205.
23. Jeong HJ, Nam H, Jang J, et al., 2020, 3D bioprinting strategies for the regeneration of functional tubular tissues and organs. Bioengineering, 7:32.
24. Dhariwala B, Hunt E, Boland T, 2004, Rapid prototyping of tissue-engineering constructs, using photopolymerizable hydrogels and stereolithography. Tissue Eng, 10:1316–1322.
25. Melchels FPW, Feijen J, Grijpma DW, 2010, A review on stereolithography and its applications in biomedical engineering. Biomaterials, 31:6121–6130.
26. Lopez de Armentia S, Fernández-Villamarín S, Ballesteros Y, et al., 2022, 3D printing of a graphene-modified photopolymer using stereolithography for biomedical applications: A study of the polymerization reaction. Int J Bioprint, 8:503.
27. Heinrich MA, Liu W, Jimenez A, et al., 2019, 3D bioprinting: From benches to translational applications. Small, 15:e1805510.
28. Moura D, Pereira RF, Gonçalves IC, 2022, Recent advances on bioprinting of hydrogels containing carbon materials. Mater Today Chem, 23:100617.
29. Seo JW, Kim GM, Choi Y, et al., 2022, Improving printability of digital-light-processing 3D bioprinting via photoabsorber pigment adjustment. Int J Mol Sci, 23:5428.
30. Mironov V, Boland T, Trusk T, et al., 2003, Organ printing: Computer-aided jet-based 3D tissue engineering. Trends Biotechnol, 21:157–161.
31. Shafiee A, Atala A, 2016, Printing technologies for medical applications. Trends Mol Med, 22:254–265.
32. Fielding GA, Bandyopadhyay A, Bose S, 2012, Effects of silica and zinc oxide doping on mechanical and biological properties of 3D printed tricalcium phosphate tissue engineering scaffolds. Dent Mater, 28:113–122.
33. Ahmed M, Vadivelu R, John J, et al., 2016, Three-dimensional printing of biological matters. J Sci Adv Mater Devices, 1:1–17.
34. Li Q, Zhang B, Xue Q, et al., 2021, A systematic thermal analysis for accurately predicting the extrusion printability of alginate–gelatin-based hydrogel bioinks. Int J Bioprint, 7:394.
35. Matai I, Kaur G, Seyedsalehi A, et al., 2020, Progress in 3D bioprinting technology for tissue/organ regenerative engineering. Biomaterials, 226:119536.
36. Liu W, Zhang YS, Heinrich MA, et al., 2017, Rapid continuous Multimaterial mxtrusion bioprinting. Adv Mater, 29:1604630.
37. Kreimendahl F, Köpf M, Thiebes AL, et al., 2017, Three dimensional printing and angiogenesis: Tailored agarosetype I collagen blends comprise three-dimensional printability and angiogenesis potential for tissue-engineered substitutes. Tissue Eng Part C Methods, 23:604–615.
38. Noh I, Kim N, Tran HN, et al., 2019, 3D Printable hyaluronic acid-based hydrogel for its potential application as a bioink in tissue engineering. Biomat Res, 23:3.
39. Pereira RF, Sousa A, Barrias CC, et al., 2018, A single component hydrogel bioink for bioprinting of bioengineered 3D constructs for dermal tissue engineering. Mater Horiz, 5:1100–1111.
40. Ashammakhi N, Ahadian S, Xu C, et al., 2019, Bioinks and bioprinting technologies to make heterogeneous and biomimetic tissue constructs. Mater Today Bio, 1:100008.
41. Suntornnond R, Tan EYS, An J, et al., 2017, A highlyprintable and biocompatible hydrogel c omposite for direct printing of soft and perfusable vasculature-like structures. Sci Rep, 7:16902.
42. Cernencu AI, Lungu A, Dragusin DM, et al., 2021, 3D bioprinting of biosynthetic nanocellulose-filled GelMA inks highly reliable for soft tissue-oriented constructs. Materials, 14:4891.
43. Pereira RF, Bártolo PJ, 2015, 3D bioprinting of photocrosslinkable hydrogel constructs. J Appl Polym Sci, 132:42458.
44. Escobar-Chávez JJ, López-Cervantes M, Naïk A, et al., 2006, Applications of thermo-reversible pluronic F-127 gels in pharmaceutical formulations. J Pharm Sci, 9:339–358.
45. Setayeshmehr M, Hafeez S, van Blitterswijk C, et al., 2021, Bioprinting via a dual-gel bioink based on poly(vinyl alcohol) and solubilized extracellular matrix towards cartilage engineering. Int J Mol Sci, 22:3901.
46. Ouyang L, Highley CB, Sun W, et al., 2017, A generalizable strategy for the 3D bioprinting of hydrogels from nonviscous photo-crosslinkable inks. Adv Mater, 29:1604983.
47. Bertlein S, Brown G, Lim KS, et al., 2017, Thiol-ene clickable gelatin: A platform bioink for multiple 3D biofabrication technologies. Adv Mater, 29:1703404.
48. Wang SJ, Zhang ZZ, Jiang D, et al., 2016, Thermogel-coated poly(ε-caprolactone) composite scaffold for enhanced cartilage tissue engineering. Polymers, 8:200.
49. Zhang K, Xue K, Loh XJ, 2021, Thermo-responsive hydrogels: From recent progress to biomedical applications. Gels, 7:77.
50. Iglesias-Mejuto A, García-González CA, 2022, 3D-printed, dual crosslinked and sterile aerogel scaffolds for bone tissue engineering. Polymers, 14:1211.
51. Mora-Boza A, Włodarczyk-Biegun MK, Del Campo A, et al., 2019, Glycerylphytate as an ionic crosslinker for 3D printing of multi-layered scaffolds with improved shape fidelity and biological features. Biomater Sci, 8:506–516.
52. Olmos-Juste R, Alonso-Lerma B, Pérez-Jiménez R, et al., 2021, 3D printed alginate-cellulose nanofibers based patches for local curcumin administration. Carbohydr Polym, 264:118026.
53. Howell DW, Peak CW, Bayless KJ, et al., 2018, 2D nanosilicates loaded with proangiogenic factors stimulate endothelial sprouting. Adv Biosyst, 2:1800092.
54. Bendtsen ST, Quinnell SP, Wei M, 2017, Development of a novel alginate-polyvinyl alcohol-hydroxyapatite hydrogel for 3D bioprinting bone tissue engineered scaffolds. J Biomed Mater Res Part A, 105:1457–1468.
55. Bonnelye E, Chabadel A, Saltel F, et al., 2008, Dual effect of strontium ranelate: Stimulation of osteoblast differentiation and inhibition of osteoclast formation and resorption in vitro. Bone, 42:129–138.
56. Jeong WY, Kang MS, Lee H, et al., 2021, Recent trends in photoacoustic imaging techniques for 2D nanomaterialbased phototherapy. Biomedicines, 9:80.
57. Yu D, Goh K, Wang H, et al., 2014, Scalable synthesis of hierarchically structured carbon nanotube–graphene fibres for capacitive energy storage. Nat Nanotechnol, 9:555–562.
58. Rueda-Gensini L, Serna JA, Cifuentes J, et al., 2021, Graphene oxide-embedded extracellular matrix-derived hydrogel as a multiresponsive platform for 3D bioprinting applications. Int J Bioprint, 7:353.
59. Raja IS, Song SJ, Kang MS, et al., 2019, Toxicity of zero- and one-dimensional carbon nanomaterials. Nanomaterials, 9:1214.
60. Shin YC, Song SJ, Jeong SJ, et al., 2018, Graphene-based nanocomposites as promising options for hard tissue regeneration. Adv Exp Med Biol, 1078:103–117.
61. Kang MS, Song S-J, Cha JH, et al., 2020, Increased neuritogenesis on ternary nanofiber matrices of PLCL and laminin decorated with black phosphorus. J Ind Eng Chem, 92:226–235.
62. Raja IS, Kang MS, Kim KS, et al., 2020, Two-dimensional theranostic nanomaterials in cancer treatment: State of the art and perspectives. Cancers, 12:1657.
63. Raja IS, Lee JH, Hong SW, et al., 2021, A critical review on genotoxicity potential of low dimensional nanomaterials. J Hazard Mater, 409:124915.
64. Mahor A, Singh PP, Bharadwaj P, et al., 2021, Carbon-based nanomaterials for delivery of biologicals and therapeutics: A cutting-edge technology. C, 7:19.
65. Perkins BL, Naderi N, 2016, Carbon nanostructures in bone tissue engineering. Open Orthop J, 10:877–899.
66. Rauti R, Musto M, Bosi S, et al., 2019, Properties and behavior of carbon nanomaterials when interfacing neuronal cells: How far have we come? Carbon, 143:430–446.
67. Kang MS, Lee JH, Hong SW, et al., 2021, Nanocomposites for enhanced osseointegration of dental and orthopedicimplants revisited: Surface functionalization by carbon nanomaterial coatings. J Compos Sci, 5:23.
68. Kang MS, Jang HJ, Lee SH, et al., 2021, Potential of carbon-based nanocomposites for dental tissue engineering and regeneration. Materials, 14:5104.
69. Li Q, Song J, Besenbacher F, et al., 2015, Two-dimensional material confined water. Acc Chem Res, 48:119–127.
70. Yu D, Goh K, Wang H, et al., 2014, Scalable synthesis of hierarchically structured carbon nanotube-graphene fibres for capacitive energy storage. Nat Nanotechnol, 9:555–562.
71. Liu Y, Dong X, Chen P, 2012, Biological and chemical sensors based on graphene materials. Chem Soc Rev, 41:2283–2307.
72. Raja IS, Vedhanayagam M, Preeth DR, et al., 2021, Development of two-dimensional nanomaterials based electrochemical biosensors on enhancing the analysis of food toxicants. Int J Mol Sci, 22:3277.
73. Shin YC, Bae J-H, Lee JH, et al., 2022, Enhanced osseointegration of dental implants with reduced graphene oxide coating. Biomater Res, 26:11.
74. Vedhanayagam M, Raja IS, Molkenova A, et al., 2021, Carbon dots-mediated fluorescent scaffolds: Recent trends in image-guided tissue engineering applications. Int J Mol Sci, 22:5378.
75. Kang MS, Lee H, Jeong S, et al., 2022, State of the art in carbon nanomaterials for photoacoustic imaging. Biomedicines, 10:1374.
76. Konios D, Stylianakis MM, Stratakis E, et al., 2014, Dispersion behaviour of graphene oxide and reduced graphene oxide. J Colloid Interface Sci, 430:108–112.
77. Li H, Song SI, Song GY, et al., 2014, Non-covalently functionalized carbon nanostructures for synthesizing carbon-based hybrid nanomaterials. J Nanosci Nanotechnol, 14:1425–1440.
78. Adorinni S, Rozhin P, Marchesan S, 2021, Smart hydrogels meet carbon nanomaterials for new frontiers in medicine. Biomedicines, 9:570.
79. Simon J, Flahaut E, Golzio M. 2019, Overview of carbon nanotubes for biomedical applications. Materials, 12:624.
80. Debnath SK, Srivastava R. 2021, Drug delivery with carbon-based nanomaterials as versatile nanocarriers: Progress and prospects. Front Nanotechnol, 3:644564.
81. Luo X, Matranga C, Tan S, et al., 2011, Carbon nanotube nanoreservior for controlled release of anti-inflammatory dexamethasone. Biomaterials, 32:6316–6323.
82. Chae SY, Shin MC, Jeon S, et al., 2021, Simple route to the complexation of lutein with reduced graphene oxide nanocarriers and antioxidant protection against blue light. Int J Nanomed, 16:6843–6860.
83. Yin F, Hu K, Chen Y, et al., 2017, SiRNA delivery with PEGylated graphene oxide nanosheets for combined photothermal and genetherapy for pancreatic cancer. Theranostics, 7:1133–1148.
84. Chen H, Huang J, Fam DWH, et al., 2016, Horizontally aligned carbon nanotube based biosensors for protein detection. Bioengineering, 3:23.
85. Ojeda I, Barrejón M, Arellano LM, et al., 2015, Grafted double walled carbon nanotubes as electrochemical platforms for immobilization of antibodies using a metallic-complex chelating polymer: Application to the determination of adiponectin cytokine in serum, Biosens Bioelectron, 74:24–29.
86. Lee JH, Lee Y, Shin YC, et al., 2016, In situ forming gelatin/graphene oxide hydrogels for facilitated C2C12 myoblast differentiation. Appl Spectrosc Rev, 51:527–539.
87. Ravanbakhsh H, Bao G, Mongeau L, 2020, Carbon nanotubes promote cell migration in hydrogels. Sci Rep, 10: 2543.
88. Jo H, Sim M, Semin K, et al., 2016, Electrically conductive graphene/polyacrylamide hydrogels produced by mild chemical reduction for enhanced myoblast growth and differentiation. Acta Biomater, 48; 100–109.
89. Li H, Tan C, Li L, 2018, Review of 3D printable hydrogels and constructs. Mater Des, 159:20–38.
90. Groll J, Burdick JA, Cho DW, et al., 2018, A definition of bioinks and their distinction from biomaterial inks. Biofabrication, 11:013001.
91. Schuurman W, Khristov V, Pot MW, et al., 2011, Bioprinting of hybrid tissue constructs with tailorable mechanical properties. Biofabrication, 3:021001.
92. Kaushik SN, Kim B, Walma AM, et al., 2016, Biomimetic microenvironments for regenerative endodontics. Biomater Res, 20:14.
93. Bhattacharyya A, Janarthanan G, Noh I, 2021, Nanobiomaterials for designing functional bioinks towards complex tissue and organ regeneration in 3D bioprinting. Addit Manuf, 37:101639.
94. Liu X, Miller AL, Park S, et al., 2019, Two-dimensional black phosphorus and graphene oxide nanosheets synergistically enhance cell proliferation and osteogenesis on 3D printed scaffolds. ACS Appl Mater Interfaces, 11:23558–23572.
95. Olate-Moya F, Arens L, Wilhelm M, et al., 2020, Chondroinductive alginate-based hydrogels having graphene oxide for 3D printed scaffold fabrication. ACS Appl Mater Interfaces, 12:4343–4357.
96. Bordoni M, Karabulut E, Kuzmenko V, et al., 2020, 3D printed conductive nanocellulose scaffolds for the differentiation of human neuroblastoma cells. Cells, 9:682.
97. Janarthanan G, Lee S, Noh I, 2021, 3D printing of bioinspired alginate-albumin based instant gel ink with electroconductivity and its expansion to direct four-axis printing of hollow porous tubular constructs without supporting materials. Adv Funct Mater, 31:2104441.
98. Cui H, Yu Y, Li X, et al., 2019, Direct 3D printing of a tough hydrogel incorporated with carbon nanotubes for bone regeneration. J Mater Chem B, 7:7207–7217.
99. Lee SJ, Zhu W, Nowicki M, et al., 2018, 3D printing nano conductive multi-walled carbon nanotube scaffolds for nerve regeneration. J Neural Eng, 15:016018.
100. Li L, Qin S, Peng J, et al., 2020, Engineering gelatin-based alginate/carbon nanotubes blend bioink for direct 3D printing of vessel constructs. Int J Biol Macromol, 145:262–271.
101. Liu X, George MN, Park S, et al., 2020, 3D-printed scaffolds with carbon nanotubes for bone tissue engineering: Fast and homogeneous one-step functionalization. Acta Biomater, 111:129–140.
102. Serafin A, Murphy C, Rubio MC, et al., 2021, Printable alginate/gelatin hydrogel reinforced with carbon nanofibers as electrically conductive scaffolds for tissue engineering. Mater Sci Eng C Mater Biol Appl, 122:111927.
103. Bilge S, Ergene E, Talak E, et al., 2021, Recycled algae-based carbon materials as electroconductive 3D printed skeletal muscle tissue engineering scaffolds. J Mater Sci Mater Med, 32:73.
104. Huang CT, Kumar Shrestha L, Ariga K, et al., 2017, A graphene-polyurethane composite hydrogel as a potential bioink for 3D bioprinting and differentiation of neural stem cells. J Mater Chem B, 5:8854–8864.
105. Ajiteru O, Sultan MT, Lee YJ, et al., 2020, A 3D printable electroconductive biocomposite bioink based on silk fibroin conjugated graphene oxide. Nano Lett, 20:6873–6883.
106. Kang MS, Kang JI, Le Thi P, et al., 2021, Three-dimensional printable gelatin hydrogels incorporating graphene oxide to enable spontaneous myogenic differentiation. ACS Macro Lett, 10:426–432.
107. Xavier Mendes A, Moraes Silva S, O’Connell CD, et al., 2021, Enhanced electroactivity, mechanical properties, and printability through the addition of graphene oxide to photo-cross-linkable gelatin methacryloyl hydrogel. ACS Biomater Sci Eng, 7:2279–2295.
108. Izadifar M, Chapman D, Babyn P, et al., 2018, UV-assisted 3D bioprinting of nanoreinforced hybrid cardiac patch for myocardial tissue engineering. Tissue Eng Part C Methods, 24:74–88.
109. Liu J, Li L, Suo H, et al., 2019, 3D printing of biomimetic multi-layered GelMa/nHA scaffold for osteochondral defect repair. Materi Des, 171:107708.
110. Gandra N, Wang DD, Zhu Y, et al., 2013 Virus-mimetic cytoplasm-cleavable magnetic/silica nanoclusters for enhanced gene delivery to mesenchymal stem cells. Angew Chem, 52:11278–11281.
111. Rudiansyah M, El-Sehrawy AA, Ahmad I, et al., 2022, Osteoporosis treatment by mesenchymal stromal/stem cells and their exosomes: Emphasis on signaling pathways and mechanisms. Life Sci, 306:120717.
112. Bhatt RA, Rozental TD, 2012, Bone graft substitutes. Hand Clin, 28:457–468.
113. Calikoglu Koyuncu AC, Gurel Pekozer G, Ramazanoglu M, et al., 2017, Cartilage tissue engineering on microporous scaffolds using human tooth germ stem cells. J Tissue Eng Regen Med, 11:765–777.
114. Ito A, Yamamoto Y, Sato M, et al., 2014, Induction of functional tissue-engineered skeletal muscle constructs by defined electrical stimulation. Sci Rep, 4:4781.
115. Dvir T, Timko BP, Brigham MD, et al., 2011, Nanowired three dimensional cardiac patches. Nat Nanotechnol, 6:720–725.
116. Kota AK, Cipriano BH, Duesterberg MK, et al., 2007, Electrical and rheological percolation in polystyrene/ MWCNT nanocomposites. Macromolecules, 40:7400–7406.