Ovary-derived Decellularized Extracellular Matrix-based Bioink for Fabricating 3D Primary Ovarian Cells-laden Structures for Mouse Ovarian Failure Correction
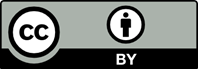
Fertility preservation is becoming a clinical duty in practice. Three-dimensional (3D) bioprinting technology is potentially realize ovarian morphological repair and reproductive endocrine function rebuild. There is no published work on 3D bioprinting ovary using a decellularized extracellular matrix (dECM)-based bioink, though dECM is the preferred matrix choice for an artificial ovary. The study aimed to explore swine ovarian dECM-based bioink to fabricate 3D primary ovarian cells (POCs)-laden structures for mouse ovarian failure correction. In this study, the ovarian dECM was converted to dECM-based bioink by dECM solution mixed with a seaweed gelatin blend solution of bioink that was characterized using scanning electron microscopy, circular dichroism, rheology, hematoxylin and eosin staining, and immunohistochemistry. The 3D scaffolds were, then, printed with or without POCs by the extrusion 3D bioprinter. The laden POCs viability was detected with the live/dead assay kit. A female castrated mouse model was established, and the mice were treated with five different methods. The results revealed that the 3D scaffold encapsulating POCs group had more positive signals in neoangiogenesis, cell proliferation and survival than the 3D scaffold group, and ensured sex hormone secretion. Meanwhile, the expression of germ cells in the 3D scaffold encapsulating POCs group was more intensely than the non-printed hydrogel encapsulating POCs group. The work shows that the 3D bioprinting ovary employing ovarian dECM-based bioink is a promising approach for ovarian failure correction.
1. Siegel RL, Miller KD, Fuchs HE, 2021, Cancer Statistics, 2021. CA Cancer J Clin, 71:7–33. https://doi.org/10.3322/caac.21654
2. Dolmans MM, Luyckx V, Donnez J, et al., 2013, Affiliations Expand Risk of Transferring Malignant Cells with Transplanted Frozen-thawed Ovarian Tissue. Fertil Steril, 99:1514–22. https://doi.org/10.1016/j.fertnstert.2013.03.027
3. Dath C, Dethy A, Van Langendonckt A, et al., 2011, Endothelial Cells are Essential for Ovarian Stromal Tissue Restructuring after Xenotransplantation of Isolated Ovarian Stromal Cells. Hum Reprod, 26:1431–9. https://doi.org/10.1093/humrep/der073
4. Rodgers RJ, Irving-Rodgers HF, Russell DL, 2003, Extracellular Matrix of the Developing Ovarian Follicle. Reproduction, 126:415–24. https://doi.org/10.1530/rep.0.1260415
5. Chiti MC, Dolmans MM, Mortiaux L, et al., 2018, A Novel Fibrin-based Artificial Ovary Prototype Resembling Human Ovarian Tissue in Terms of Architecture and Rigidity. J Assist Reprod Genet, 35:41–8. https://doi.org/10.1007/s10815-017-1091-3
6. Day JR, David A, Cichon AL, et al., 2018, Immunoisolating Poly(Ethylene Glycol) based Capsules Support Ovarian Tissue Survival to Restore Endocrine Function. J Biomed Mater Res A, 106:1381–9. https://doi.org/10.1002/jbm.a.36338
7. Amorim CA, Shikanov A, 2016, The Artificial Ovary: Current Status and Future Perspectives. Future Oncol, 12:2323–32. https://doi.org/10.2217/fon-2016-0202
8. Sellaro TL, Ranade A, Faulk DM, et al., 2010, Maintenance of Human Hepatocyte Function in vitro by Liver-derived Extracellular Matrix Gels. Tissue Eng Part A, 16:1075–82. https://doi.org/10.1089/ten.TEA.2008.0587
9. Laronda MM, Jakus AE, Whelan KA, et al., 2015, Initiation of Puberty in Mice Following Decellularized Ovary Transplant. Biomaterials, 50:20–9. https://doi.org/10.1016/j.biomaterials.2015.01.051
10. Liu WY, Lin SG, Zhuo RY, et al., 2017, Xenogeneic Decellularized Scaffold: A Novel Platform for Ovary Regeneration. Tissue Eng Part C Methods, 23:61–71. https://doi.org/10.1089/ten.TEC.2016.0410
11. Hassanpour A, Talaei-Khozani T, Kargar-Abarghouei E, et al., 2018, Decellularized Human Ovarian Scaffold based on a Sodium Lauryl Ester Sulfate (SLES)-treated Protocol, as a Natural Three-dimensional Scaffold for Construction of Bioengineered Ovaries. Stem Cell Res Ther, 9:252. https://doi.org/10.1186/s13287-018-0971-5
12. Pors SE, Ramløse M, Nikiforov D, et al., 2019, Initial Steps in Reconstruction of the Human Ovary: Survival of Pre-antral Stage Follicles in a Decellularized Human Ovarian Scaffold. Hum Reprod, 34:1523–35. https://doi.org/10.1093/humrep/dez077
13. Chiti C, Viswanath A, Vanacker J, et al., 2016, Development of a Transplantable Artificial Ovary: Influence of Follicle Stage on Transplantation Outcome. In: Front Bioeng Biotechnol, Conference Abstract: 10th World Biomaterials Congress. https://doi.org/10.3389/conf.FBIOE.2016.01.00699
14. Moroni L, Boland T, Burdick JA, et al., 2018, Biofabrication: A Guide to Technology and Terminology. Trends Biotechnol, 36:384–402. https://doi.org/10.1016/j.tibtech.2017.10.015
15. Groll J, Burdick JA, Cho DW, et al., 2018, A Definition of Bioinks and their Distinction from Biomaterial Inks. Biofabrication, 11:013001. https://doi.org/10.1088/1758-5090/aaec52
16. Abaci A, Guvendiren M, 2020, Designing Decellularized Extracellular Matrix-Based Bioinks for 3D Bioprinting. Adv Healthc Mater, 9:e2000734. https://doi.org/10.1002/adhm.202000734
17. Hou CX, Zheng JH, Li ZK, et al., 2021, Printing 3D Vagina Tissue Analogues with Vagina Decellularized Extracellular Matrix Bioink. Int J Biol Macromol., 180:177–86. https://doi.org/10.1016/j.ijbiomac.2021.03.070
18. Badylak SF, 2004, Xenogeneic Extracellular Matrix as a Scaffold for Tissue Reconstruction. Transpl Immunol, 12:367–77. https://doi.org/10.1016/j.trim.2003.12.016
19. Petersen TH, Calle EA, Zhao L, et al., 2010, Tissue-engineered Lungs for in vivo Implantation. Science, 329:538–41. https://doi.org/10.1126/science.1189345
20. Uygun BE, Soto-Gutierrez A, Yagi H, et al., 2010, Organ Reengineering Through Development of a Transplantable Recellularized Liver Graft using Decellularized Liver Matrix. Nat Med, 16:814–20. https://doi.org/10.1038/nm.2170
21. Crapo PM, Gilbert TW, Badylak SF, 2011, An Overview of Tissue and whole Organ Decellularization Processes. Biomaterials, 32:3233–43. https://doi.org/10.1016/j.biomaterials.2011.01.057
22. Caralt M, Uzarski JS, Iacob S, et al, 2015, Optimization and Critical Evaluation of Decellularization Strategies to Develop Renal Extracellular Matrix Scaffolds as Biological Templates for Organ Engineering and Transplantation. Am J Transplant, 15:64–75. https://doi.org/10.1111/ajt.12999
23. Badylak SF, Gilbert TW, 2008, Immune Response to Biologic Scaffold Materials. Semin Immunol, 20:109–16. https://doi.org/10.1016/j.smim.2007.11.003
24. Heeren AM, Iperen LV, Klootwijk DB, et al., 2015, Fernandes Development of the Follicular Basement Membrane during Human Gametogenesis and Early Folliculogenesis. BMC Dev Biol, 15:4. https://doi.org/10.1186/s12861-015-0054-0
25. Streuli C, 1999, Extracellular Matrix Remodelling and Cellular Differentiation. Curr Opin Cell Biol, 11:634–40. https://doi.org/10.1016/s0955-0674(99)00026-5
26. Welham NV, Chang Z, Smith LM, et al., 2013, Proteomic Analysis of a Decellularized Human Vocal Fold Mucosa Scaffold using 2D Electrophoresis and High-resolution Mass Spectrometry. Biomaterials, 34:669–76. https://doi.org/10.1016/j.biomaterials.2012.09.050
27. Turner AEB, Yu C, Bianco J, et al., 2012, The Performance of Decellularized Adipose Tissue Microcarriers as an Inductive Substrate for Human Adipose-derived Stem Cells. Biomaterials, 33:4490–9. https://doi.org/10.1016/j.biomaterials.2012.03.026
28. Yu C, Bianco J, Brown C, et al., 2013, Porous Decellularized Adipose Tissue Foams for Soft Tissue Regeneration. Biomaterials, 34:3290–302. https://doi.org/10.1016/j.biomaterials.2013.01.056
29. Wang XH, Yan YN, Zhang RJ, 2007, Rapid Prototyping as a Tool for Manufacturing Bioartificial Livers. Trends Biotechnol, 25:505–13. https://doi.org/10.1016/j.tibtech.2007.08.010
30. Vijayavenkataraman S, Yan WC, Lu WF, et al., 2018, 3D Bioprinting of Tissues and Organs for Regenerative Medicine. Adv Drug Deliv Rev, 132:296–332. https://doi.org/10.1016/j.addr.2018.07.004
31. Ferlin KM, Prendergast ME, Miller ML, et al., 2016, Influence of 3D Printed Porous Architecture on Mesenchymal Stem Cell Enrichment and Differentiation. Acta Biomater, 32:161–9. https://doi.org/10.1016/j.actbio.2016.01.007
32. Hu K, Cui F, Lv Q, et al., 2008, Preparation of Fibroin/Recombinant Human-like Collagen Scaffold to Promote Fibroblasts Compatibility. J Biomed Mater Res A, 84:483–90. https://doi.org/10.1002/jbm.a.31440
33. Pati F, Ha DH, Jang J, et al., 2015, Biomimetic 3D Tissue Printing for Soft Tissue Regeneration. Biomaterials, 62:164–75. https://doi.org/10.1016/j.biomaterials.2015.05.043
34. Lee A, Hudson AR, Shiwarski DJ, et al., 2019, 3D Bioprinting of Collagen to Rebuild Components of the Human Heart. Science, 365:482–7. https://doi.org/10.1126/science.aav9051
35. Zor F, Selek FN, Orlando G, et al., 2019, Biocompatibility in Regenerative Nanomedicine. Nanomedicine (Lond), 14:2763–75. https://doi.org/10.2217/nnm-2019-0140
36. Brown BN, Freund JM, Han L, et al., 2011, Comparison of Three Methods for the Derivation of a Biologic Scaffold Composed of Adipose Tissue Extracellular Matrix. Tissue Eng Part C Methods, 17:411–21. https://doi.org/10.1089/ten.TEC.2010.0342
37. Dolmans MM, Amorim CA, 2019, Fertility Preservation: Construction and use of Artificial Ovaries. Reproduction, 158:F15–25. https://doi.org/10.1530/REP-18-0536
38. Maghsoudlou P, Georgiades F, Tyraskis A, et al., 2013, Preservation of Micro-architecture and Angiogenic Potential in a Pulmonary Acellular Matrix Obtained using Intermittent Intra-tracheal Flow of Detergent Enzymatic Treatment. Biomaterials, 34:6638–48. https://doi.org/10.1016/j.biomaterials.2013.05.015
39. Kim YJ, Kim YY, Song DY, et al., 2018. Proliferation Profile of Uterine Endometrial Stromal Cells during in vitro Culture with Gonadotropins: Recombinant Versus Urinary Follicle Stimulating Hormone. Tissue Eng Regen Med, 16:131–9. https://doi.org/10.1007/s13770-018-0156-4