Bone Sialoprotein Immobilized in Collagen Type I Enhances Bone Regeneration In vitro and In vivo
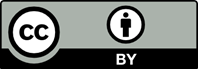
The use of bioactive molecules is a promising approach to enhance the bone healing properties of biomaterials. The aim of this study was to define the role of bone sialoprotein (BSP) immobilized in collagen type I in various settings. In vitro studies with human primary osteoblasts in mono- or in co-culture with endothelial cells demonstrated a slightly increased gene expression of osteogenic markers as well as an increased proliferation rate in osteoblasts after application of BSP immobilized in collagen type I. Two critical size bone defect models were used to analyze bone regeneration. BSP incorporated in collagen type I increased bone regeneration only marginally at one concentration in a calvarial defect model. To induce the mechanical stability, three-dimensional printing was used to produce a stable porous cylinder of polylactide. The cylinder was filled with collagen type I and immobilized BSP and implanted into a femoral defect of critical size in rats. This hybrid material was able to significantly induce bone regeneration. Our study clearly shows the osteogenic effect of BSP when combined with collagen type I as carrier and thereby offers various approaches and options for its use as bioactive molecule in bone substitute materials.
1. Florencio-Silva R, Sasso GR, Sasso-Cerri E, et al., 2015, Biology of Bone Tissue: Structure, Function, and Factors That Influence Bone Cells. Biomed Res Int, 2015:421746. https://doi.org/1155/2015/421746
2. Staines KA, MacRae VE, Farquharson C, 2012, The Importance of the SIBLING Family of Proteins on Skeletal Mineralisation and Bone Remodelling. J Endocrinol, 214:241–55. https://doi.org/1530/JOE-12-0143
3. Vincent K, Durrant MC, 2013, A Structural and Functional Model for Human Bone Sialoprotein. J Mol Graph Model, 39:108–17. https://doi.org/1016/j.jmgm.2012.10.007
4. Gordon JA, Tye CE, Sampaio AV, et al., 2007, Bone Sialoprotein Expression Enhances Osteoblast Differentiation and Matrix Mineralization In Vitro. Bone, 41:462–73. https://doi.org/1016/j.bone.2007.04.191
5. Bouleftour W, Juignet L, Bouet G, et al., 2016, The Role of the SIBLING, Bone Sialoprotein in Skeletal Biology Contribution of Mouse Experimental Genetics. Matrix Biol, 52–54:60–77. https://doi.org/1016/j.matbio.2015.12.011
6. Monfoulet L, Malaval L, Aubin JE, et al., 2010, Bone Sialoprotein, but not Osteopontin, Deficiency Impairs the Mineralization of Regenerating Bone during Cortical Defect Healing. Bone, 46:447–52. https://doi.org/1016/j.bone.2009.09.007
7. Boudiffa M, Wade-Gueye NM, Guignandon A, et al., 2010, Bone Sialoprotein Deficiency Impairs Osteoclastogenesis and Mineral Resorption In Vitro. J Bone Miner Res, 25:2669–79. https://doi.org/1002/jbmr.245
8. Malaval L, Wade-Gueye NM, Boudiffa M, et al., 2008, Bone Sialoprotein Plays a Functional Role in Bone Formation and Osteoclastogenesis. J Exp Med, 205:1145–53. https://doi.org/1084/jem.20071294
9. Bouleftour W, Juignet L, Verdiere L, et al., 2019, Deletion of OPN in BSP Knockout Mice does not Correct Bone Hypomineralization but Results in High Bone Turnover. Bone, 120:411–22. https://doi.org/1016/j.bone.2018.12.001
10. Holm E, Aubin JE, Hunter GK, et al., 2015, Loss of Bone Sialoprotein Leads to Impaired Endochondral Bone Development and Mineralization. Bone, 71:145–54. https://doi.org/1016/j.bone.2014.10.007
11. Baranowski A, Klein A, Ritz U, et al., 2016, Surface Functionalization of Orthopedic Titanium Implants with Bone Sialoprotein. PLoS One, 11:e0153978. https://doi.org/1371/journal.pone.0153978
12. Klein A, Baranowski A, Ritz U, et al., 2018, Effect of Bone Sialoprotein Coated Three-dimensional Printed Calcium Phosphate Scaffolds on Primary Human Osteoblasts. J Biomed Mater Res B Appl Biomater, 106:2565–75. https://doi.org/1002/jbm.b.34073
13. Gomes S, Gallego-Llamas J, Leonor IB, et al., 2013, In Vivo Biological Responses to Silk Proteins Functionalized with Bone Sialoprotein. Macromol Biosci, 13:444–54. https://doi.org/1002/mabi.201200372
14. Baranowski A, Klein A, Ritz U, et al., 2018, Evaluation of Bone Sialoprotein Coating of Three-Dimensional Printed Calcium Phosphate Scaffolds in a Calvarial Defect Model in Mice. Materials (Basel), 11:2336. https://doi.org/3390/ma11112336
15. Klein A, Baranowski A, Ritz U, et al., 2020, Effect of Bone Sialoprotein Coating on Progression of Bone Formation in a Femoral Defect Model in Rats. Eur J Trauma Emerg Surg, 46:277–86. https://doi.org/1007/s00068-019-01159-5
16. Wallace DG, Rosenblatt J, 2003, Collagen Gel Systems for Sustained Delivery and Tissue Engineering. Adv Drug Deliv Rev, 55:1631–49.
17. Bierbaum S, Hintze V, Scharnweber D, 2012, Functionalization of Biomaterial Surfaces Using Artificial Extracellular Matrices. Biomatter, 2:132–41. https://doi.org/4161/biom.20921
18. Motamedian SR, Hosseinpour S, Ahsaie MG, et al., 2015, Smart Scaffolds in Bone Tissue Engineering: A Systematic Review of Literature. World J Stem Cells, 7:657–68. https://doi.org/4252/wjsc.v7.i3.657
19. Tye CE, Hunter GK, Goldberg HA, 2005, Identification of the Type I Collagen-binding Domain of Bone Sialoprotein and Characterization of the Mechanism of Interaction. J Biol Chem., 280:13487–92. https://doi.org/1074/jbc.M408923200
20. Wang J, Zhou HY, Salih E, et al., 2006, Site-specific In Vivo Calcification and Osteogenesis Stimulated by Bone Sialoprotein. Calcif Tissue Int, 79:179–89. https://doi.org/1007/s00223-006-0018-2
21. Xu L, Anderson AL, Lu Q, et al., 2007, Role of Fibrillar Structure of Collagenous Carrier in Bone Sialoprotein mediated Matrix Mineralization and Osteoblast Differentiation. Biomaterials, 28:750–61. https://doi.org/1016/j.biomaterials.2006.09.022
22. Kruger TE, Miller AH, Wang J, 2013, Collagen Scaffolds in Bone Sialoprotein-Mediated Bone Regeneration. Scientific World Journal, 2013:812718. https://doi.org/1155/2013/812718
23. Gorski JP, Franz NT, Pernoud D, et al., 2021, A Repeated Triple Lysine Motif Anchors Complexes Containing Bone Sialoprotein and the type XI Collagen A1 Chain Involved in Bone Mineralization. J Biol Chem, 296:100436. https://doi.org/1016/j.jbc.2021.100436
24. Serra T, Mateos-Timoneda MA, Planell JA, et al., 2013, 3D Printed PLA-based Scaffolds: A Versatile Tool in Regenerative Medicine. Organogenesis, 9:239–44. https://doi.org/4161/org.26048
25. Guvendiren M, Molde J, Soares RM, et al., 2016, Designing Biomaterials for 3D Printing. ACS Biomater Sci Eng, 2:1679–93. https://doi.org/1021/acsbiomaterials.6b00121
26. Lauer A, Wolf P, Mehler D, et al., 2020, Biofabrication of SDF-1 Functionalized 3D-Printed Cell-Free Scaffolds for Bone Tissue Regeneration. Int J Mol Sci, 21:2175. https://doi.org/3390/ijms21062175
27. Ritz U, Gerke R, Gotz H, et al., 2017, A New Bone Substitute Developed from 3D-Prints of Polylactide (PLA) Loaded with Collagen I: An In Vitro Study. Int J Mol Sci, 18:2569. https://doi.org/3390/ijms18122569
28. Martin V, Ribeiro IA, Alves MM, et al., 2019, Engineering a Multifunctional 3D-Printed PLA-collagen-minocycline-nanoHydroxyapatite Scaffold with Combined Antimicrobial and Osteogenic Effects for Bone Regeneration. Mater Sci Eng C Mater Biol Appl, 101:15–26. https://doi.org/1016/j.msec.2019.03.056
29. Dewey MJ, Johnson EM, Weisgerber DW, et al., 2019, Shape-fitting Collagen-PLA Composite Promotes Osteogenic Differentiation of Porcine Adipose Stem Cells. J Mech Behav Biomed Mater, 95:21–33. https://doi.org/1016/j.jmbbm.2019.03.017
30. Hofmann A, Ritz U, Verrier S, et al., 2008, The Effect of Human Osteoblasts on Proliferation and Neo-vessel Formation of Human Umbilical Vein Endothelial Cells in a Long-term 3D Co-culture on Polyurethane Scaffolds. Biomaterials, 29:4217–26. https://doi.org/1016/j.biomaterials.2008.07.024
31. Wenger A, Stahl A, Weber H, et al., 2004, Modulation of In Vitro Angiogenesis in a Three-dimensional Spheroidal Coculture Model for Bone Tissue Engineering. Tissue Eng, 10:1536–47. https://doi.org/1089/ten.2004.10.1536
32. Livak KJ, Schmittgen TD, 2001, Analysis of Relative Gene Expression Data Using Real-time Quantitative PCR and the 2(-Delta Delta C(T)) Method. Methods, 25:402–8. https://doi.org/1006/meth.2001.1262
33. Schindelin J, Rueden CT, Hiner MC, et al., 2015, The ImageJ Ecosystem: An Open Platform for Biomedical Image Analysis. Mol Reprod Dev, 82:518–29. https://doi.org/1002/mrd.22489
34. Doube M, Klosowski MM, Arganda-Carreras I, et al., 2010, BoneJ: Free and Extensible Bone Image Analysis in ImageJ. Bone, 47:1076–9. https://doi.org/1016/j.bone.2010.08.023
35. Unger RE, Dohle E, Kirkpatrick CJ, 2015, Improving Vascularization of Engineered Bone through the Generation of Pro-angiogenic Effects in Co-culture Systems. Adv Drug Deliv Rev, 94:116–25. https://doi.org/1016/j.addr.2015.03.012
36. Unger RE, Halstenberg S, Sartoris A, et al., 2011, Human Endothelial and Osteoblast Co-cultures on 3D Biomaterials. Methods Mol Biol, 695:229–41. https://doi.org/1007/978-1-60761-984-0_15
37. Xia B, Wang J, Guo L, et al., 2011, Effect of Bone Sialoprotein on Proliferation and Osteo differentiation of Human Bone Marrow-derived Mesenchymal Stem Cells In Vitro. Biologicals, 39:217–23. https://doi.org/1016/j.biologicals.2011.04.004
38. Shi Z, Neoh KG, Kang ET, et al., 2009, Surface Functionalization of Titanium with Carboxymethyl Chitosan and Immobilized Bone Morphogenetic Protein-2 for Enhanced Osseointegration. Biomacromolecules, 10:1603–11. https://doi.org/1021/bm900203w
39. van der Zande M, Walboomers XF, Briest A, et al., 2008, The Effect of Combined Application of TGFbeta-1, BMP-2, and COLLOSS E on the Development of Bone Marrow Derived Osteoblast-like Cells In Vitro. J Biomed Mater Res Part A, 86:788–95. https://doi.org/1002/jbm.a.31645
40. Deckers MM, van Bezooijen RL, van der Horst G, et al., 2002, Bone Morphogenetic Proteins Stimulate Angiogenesis through Osteoblast-derived Vascular Endothelial Growth Factor A. Endocrinology, 143:1545–53. https://doi.org/1210/endo.143.4.8719
41. Im B J, Lee SC, Lee MH, et al., 2016, Promotion of Osteoblastic Differentiation and Osteogenic Transcription Factor Expression on a Microgroove Titanium Surface with Immobilized Fibronectin or Bone Sialoprotein II. Biomed Mater, 11:035020. https://doi.org/1088/1748-6041/11/3/035020
42. Schaeren S, Jaquiery C, Wolf F, et al., 2010, Effect of Bone Sialoprotein Coating of Ceramic and Synthetic Polymer Materials on In Vitro Osteogenic Cell Differentiation And In Vivo Bone Formation. J Biomed Mater Res Part A, 92:1461–7. https://doi.org/1002/jbm.a.32459
43. Fujisawa R, Nodasaka Y, Kuboki Y, 1995, Further Characterization of Interaction Between Bone Sialoprotein (BSP) and Collagen. Calcif Tissue Int, 56:140–4. https://doi.org/1007/BF00296346
44. Baht GS, Hunter GK, Goldberg HA, 2008, Bone Sialoproteincollagen Interaction Promotes Hydroxyapatite Nucleation. Matrix Biol, 27:600–8. https://doi.org/1016/j.matbio.2008.06.004
45. Hudieb M, Haddad A, Bakeer M, et al., 2021, Influence of Age on Calvarial Critical Size Defect Dimensions: A Radiographic and Histological Study. J Craniofac Surg, 32:2896–900. https://doi.org/1097/SCS.0000000000007690
46. Forster Y, Rentsch C, Schneiders W, et al., 2012, Surface Modification of Implants in Long Bone. Biomatter, 2:149–57. https://doi.org/4161/biom.21563
47. Bielajew BJ, Hu JC, Athanasiou KA, 2020, Collagen: Quantification, Biomechanics and Role of Minor Subtypes in Cartilage. Nat Rev Mater, 5:730–47. https://doi.org/1038/s41578-020-0213-1
48. Zang S, Mu R, Chen F, et al., 2019, Injectable Chitosan/Beta-glycerophosphate Hydrogels with Sustained Release of BMP-7 and Ornidazole in Periodontal Wound Healing of Class III Furcation Defects. Mater Sci Eng C Mater Biol Appl, 99:919–28. https://doi.org/1016/j.msec.2019.02.024
49. Schlickewei C, Klatte TO, Wildermuth Y, et al., 2019, A Bioactive Nano-calcium Phosphate Paste for In-Situ Transfection of BMP-7 and VEGF-A in a Rabbit Critical-size Bone Defect: Results of an In Vivo Study. J Mater Sci Mater Med, 30:15. https://doi.org/1007/s10856-019-6217-y
50. El Bialy I, Jiskoot W, Nejadnik MR, 2017, Formulation, Delivery and Stability of Bone Morphogenetic Proteins for Effective Bone Regeneration. Pharm Res, 34:1152–70. https://doi.org/1007/s11095-017-2147-x
51. Ritz U, Eberhardt M, Klein A, et al., 2018, Photocrosslinked Dextran-Based Hydrogels as Carrier System for the Cells and Cytokines Induce Bone Regeneration in Critical Size Defects in Mice. Gels, 4:63. https://doi.org/3390/gels4030063
52. Papanagiotou M, Dailiana ZH, Karachalios T, et al., 2017, Heterotopic Ossification after the Use of Recombinant Human Bone Morphogenetic Protein-7. World J Orthop, 8:36–41. https://doi.org/5312/wjo.v8.i1.36
53. Williams JC, Maitra S, Anderson MJ, et al., 2015, BMP-7 and Bone Regeneration: Evaluation of Dose-Response in a Rodent Segmental Defect Model. J Orthop Trauma, 29:e336–41. https://doi.org/1097/BOT.0000000000000307
54. Gillman CE, Jayasuriya AC, 2021, FDA-approved Bone Grafts and Bone Graft Substitute Devices in Bone Regeneration. Mater Sci Eng C Mater Biol Appl, 130:112466. https://doi.org/1016/j.msec.2021.112466
55. Choi YJ, Lee JY, Lee SJ, et al., 2012, Determination of Osteogenic or Adipogenic Lineages in Muscle-derived Stem Cells (MDSCs) by a Collagen-binding Peptide (CBP) Derived from Bone Sialoprotein (BSP). Biochem Biophys Res Commun, 419:326–32. https://doi.org/1016/j.bbrc.2012.02.022
56. Choi YJ, Lee JY, Chung CP, et al., 2013, Enhanced Osteogenesis by Collagen-binding Peptide from Bone Sialoprotein In Vitro and In Vivo. J Biomed Mater Res Part A, 101:547–54. https://doi.org/1002/jbm.a.34356
57. Chou YC, Yeh WL, Chao CL, et al., 2016, Enhancement of Tendon-bone Healing via the Combination of Biodegradable Collagen-loaded Nanofibrous Membranes and a Three dimensional Printed Bone-anchoring Bolt. Int J Nanomed, 11:4173–86. https://doi.org/2147/IJN.S108939
58. Nashchekina Y, Nikonov P, Nashchekin A, et al., 2020, Functional Polylactide Blend Films for Controlling Mesenchymal Stem Cell Behaviour. Polymers (Basel), 12:1969. https://doi.org/3390/polym12091969
59. Teixeira BN, Aprile P, Mendonca RH, et al., 2019, Evaluation of Bone Marrow Stem Cell Response to PLA Scaffolds Manufactured by 3D Printing and Coated with Polydopamine and Type I Collagen. J Biomed Mater Res B Appl Biomater, 107:37–49. https://doi.org/1002/jbm.b.34093