Tannic Acid-mediated Multifunctional 3D Printed Composite Hydrogel for Osteochondral Regeneration
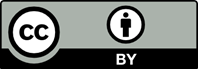
Hydrogels have become an attractive option for tissue repair. A novel multifunctional hydrogel was developed using a two-step method involving photopolymerization and tannic acid (TA) solution incubation. The mechanical properties of this hydrogel were enhanced by the multi-hydrogen bond interaction between the TA and N-acryloyl glycinamide/gelatin methacrylate (NAGA/GelMA). The compressive modulus was doubled. The compressive strengths of the hydrogel were 5.5 MPa. The swelling rate was reduced by a factor of three. The adhesion strength of the composite hydrogel reached 80 KPa. The TA-mediated NAGA/GelMA/Laponite composite hydrogel exhibited excellent anti-fatigue and anti-oxidation properties, as well as printability. In vitro experiments indicated that the TA-mediated hydrogel facilitated the proliferation of bone marrow mesenchymal stem cells and osteogenic and chondrogenic differentiation. The developed multifunctional composite hydrogel has great potential for osteochondral defect repair under osteoarthritis conditions.
1. Fang B, Qiu P, Xia C, et al., 2021, Extracellular Matrix Scaffold Crosslinked with Vancomycin for Multifunctional Antibacterial Bone Infection Therapy. Biomaterials, 268:120603. https://doi.org/10.1016/j.biomaterials.2020.120603
2. Tao B, Lin C, Yuan Z, et al., 2021, Near Infrared Light triggered On-demand Cur Release from Gel-PDA@Cur Composite Hydrogel for Antibacterial Wound Healing. Chem Eng J, 403:126182. https://doi.org/10.1016/j.cej.2020.126182
3. Wang Y, Ma M, Zhang L, et al., 2019, Fabrication of Bi-layer Photocrosslinked GelMA/PEGDA Fibrous Membrane for Guided Bone Regeneration Materials. Mater Lett, 249:112–5. https://doi.org/10.1016/j.matlet.2019.04.076
4. Ahmadian Z, Correia A, Hasany M, et al., 2021, A Hydrogen bonded Extracellular Matrix-mimicking Bactericidal Hydrogel with Radical Scavenging and Hemostatic Function for pH-Responsive Wound Healing Acceleration. Adv Healthc Mater, 10:2001122. https://doi.org/10.1002/adhm.202001122
5. Walker BW, Lara RP, Yu CH, et al., 2019, Engineering a Naturally-derived Adhesive and Conductive Cardiopatch. Biomaterials, 207:89–101. https://doi.org/10.1016/j.biomaterials.2019.03.015
6. Zhou F, Hong Y, Zhang X, et al., 2018, Tough Hydrogel with Enhanced Tissue Integration and in Situ Forming Capability for Osteochondral Defect Repair. Appl Mater Today, 13:32–44. https://doi.org/10.1016/j.apmt.2018.08.005
7. Heinrich MA, Liu W, Jimenez A, et al., 2019, 3D Bioprinting: From Benches to Translational Applications. Small, 15:1805510. https://doi.org/10.1002/smll.201805510
8. Haq M A, Su Y, Wang D, 2017, Mechanical Properties of PNIPAM Based Hydrogels: A Review. Mater Sci Eng C, 70:842–55. https://doi.org/10.1016/j.msec.2016.09.081
9. Mousa M, Evans ND, Oreffo R O C, et al., 2018, Clay nanoparticles for regenerative medicine and biomaterial design: A review of clay bioactivity. Biomaterials, 159:204–14. https://doi.org/10.1016/j.biomaterials.2017.12.024
10. Wei Y, Xiang L, Ou H, et al., 2020, MXene-based Conductive Organohydrogels with Long-term Environmental Stability and Multifunctionality. Adv Funct Mater, 30:2005135. https://doi.org/10.1002/adfm.202005135
11. Ye Y, Zhang Y, Chen Y, et al., 2020, Cellulose Nanofibrils Enhanced, Strong, Stretchable, Freezing-tolerant Ionic Conductive Organo hydrogel for Multi-Functional Sensors. Adv Funct Mater, 30:2003430. https://doi.org/10.1002/adfm.202003430
12. Hong S, Sycks D, Chan HF, et al., 2015, 3D Printing of Highly Stretchable and Tough Hydrogels into Complex, Cellularized Structures. Adv Mater, 27:4035–40. https://doi.org/10.1002/adma.201501099
13. Maiti C, Imani KB, Yoon J, 2021, Recent Advances in Design Strategies for Tough and Stretchable Hydrogels. ChemPlusChem, 86:601–11. https://doi.org/10.1002/cplu.202100074
14. Chen Y, Qiu Y, Wang Q, et al., 2020, Mussel-inspired Sandwich-like Nanofibers/Hydrogel Composite with Super Adhesive, Sustained Drug Release and Anti-infection Capacity. Chem Eng J, 399:125668. https://doi.org/10.1016/j.cej.2020.125668
15. Zhai X, Ma Y, Hou C, et al., 2017, 3D-Printed High Strength Bioactive Supramolecular Polymer/Clay Nanocomposite Hydrogel Scaffold for Bone Regeneration. ACS Biomater Sci Eng, 3:1109–18. https://doi.org/10.1021/acsbiomaterials.7b00224
16. Liu X, Yang Y, Niu X, et al., 2017, An in Situ Photocrosslinkable Platelet Rich Plasma-complexed Hydrogel Glue with Growth Factor Controlled Release Ability to Promote Cartilage Defect Repair. Acta Biomater, 62:179–87. https://doi.org/10.1016/j.actbio.2017.05.023
17. Yu Y, Shen X, Luo Z, et al., 2018, Osteogenesis Potential of Different Titania Nanotubes in Oxidative Stress Microenvironment. Biomaterials, 167:44–57. https://doi.org/10.1016/j.biomaterials.2018.03.024
18. Atashi F, Modarressi A, Pepper MS, 2015, The Role of Reactive Oxygen Species in Mesenchymal Stem Cell Adipogenic and Osteogenic Differentiation: A Review. Stem Cells Dev, 24:1150–63. https://doi.org/10.1089/scd.2014.0484
19. Jiao H, Xiao E, Graves DT, 2015, Diabetes and Its Effect on Bone and Fracture Healing. Curr Osteop Reports, 13:327–35. https://doi.org/10.1007/s11914-015-0286-8
20. Nadgorny M, Collins J, Xiao Z, et al., 2018, 3D-printing of Dynamic Self-healing Cryogels with Tuneable Properties. Polymer Chem, 9:1684–92. https://doi.org/10.1039/C7PY01945A
21. Zhou L, Ramezani H, Sun M, et al., 2020, 3D Printing of High-strength Chitosan Hydrogel Scaffolds without any Organic Solvents. Biomater Sci, 8:5020–8. https://doi.org/10.1039/d0bm00896f
22. Ge W, Cao S, Shen F, et al., 2019, Rapid Self-healing, Stretchable, Moldable, Antioxidant and Antibacterial Tannic Acid-cellulose Nanofibril Composite Hydrogels. Carbohydrate Polymers, 224:115147. https://doi.org/10.1016/j.carbpol.2019.115147
23. Lin F, Wang Z, Shen Y, et al., 2019, Natural Skin-inspired Versatile Cellulose Biomimetic Hydrogels. J Mater Chem A, 7:26442–55. https://doi.org/10.1039/C9TA10502F
24. Li Q, Xu S, Feng Q, et al., 2021, 3D Printed Silk-gelatin Hydrogel Scaffold with Different Porous Structure and Cell Seeding Strategy for Cartilage Regeneration. Bioactive Mater, 6:3396–410. https://doi.org/10.1016/j.bioactmat.2021.03.013
25. Osi AR, Zhang H, Chen J, et al., 2021, Three-Dimensional-Printable Thermo/Photo-Cross-Linked Methacrylated Chitosan-Gelatin Hydrogel Composites for Tissue Engineering. ACS Appl Mater Interfaces, 13:22902–13. https://doi.org/10.1021/acsami.1c01321
26. Shirahama H, Lee BH, Tan LP, et al., 2016, Precise Tuning of Facile One-Pot Gelatin Methacryloyl (GelMA) Synthesis. Sci Reports, 6:11. https://doi.org/10.1038/srep31036
27. Dong L, Bu Z, Xiong Y, et al., 2021, Facile Extrusion 3D Printing of Gelatine Methacrylate/Laponite Nanocomposite Hydrogel with High Concentration Nanoclay for Bone Tissue Regeneration. Int J Biol Macsromol, 188:72–81. https://doi.org/10.1016/j.ijbiomac.2021.07.199
28. Kim BJ, Oh DX, Kim S, et al., 2014, Mussel-Mimetic Protein-Based Adhesive Hydrogel. Biomacromolecules, 15:1579–85. https://doi.org/10.1021/bm4017308
29. Hong KH, 2017, Polyvinyl Alcohol/tannic Acid Hydrogel Prepared by a Freeze-thawing Process for Wound Dressing Applications. Polymer Bull, 74:2861–72. https://doi.org/10.1007/s00289-016-1868-z
30. He X, Liu X, Yang J, et al., 2020, Tannic Acid-reinforced Methacrylated Chitosan/Methacrylated Silk Fibroin Hydrogels with Multifunctionality for Accelerating Wound Healing. Carbohydrate Polymers, 247:116689. https://doi.org/10.1016/j.carbpol.2020.116689
31. Jin Y, Liu C, Chai W, et al., 2017, Self-Supporting Nanoclay as Internal Scaffold Material for Direct Printing of Soft Hydrogel Composite Structures in Air. ACS Appl Mater Interfaces, 9:17456–65. https://doi.org/10.1021/acsami.7b03613
32. Liang Q, Gao F, Zeng Z, et al., 2020, Coaxial Scale-Up Printing of Diameter-Tunable Biohybrid Hydrogel Microtubes with High Strength, Perfusability, and Endothelialization. Adv Funct Mater, 30:2001485. https://doi.org/10.1002/adfm.202001485
33. Fan Y, Zhou G, Zhang G, et al., 2020, Comparative Study on the Mechanical Behavior of the Interface between Natural Cartilage and Artificial Cartilage. Soft Mater, 19:1–20. https://doi.org/10.1080/1539445X.2020.1851258
34. Gaharwar AK, Rivera CP, Wu CJ, et al., 2011, Transparent, Elastomeric and Tough Hydrogels from Poly(ethylene Glycol) and Silicate Nanoparticles. Acta Biomater, 7:4139–48. https://doi.org/10.1016/j.actbio.2011.07.023
35. Kong HJ, Wong E, Mooney DJ, 2003, Independent Control of Rigidity and Toughness of Polymeric Hydrogels. Macromolecules, 36:4582–8. https://doi.org/10.1021/ma034137w
36. Chen YC, Lin RZ, Qi H, et al., 2012, Functional Human Vascular Network Generated in Photocrosslinkable Gelatin Methacrylate Hydrogels. Adv Funct Mater, 22:2027–39. https://doi.org/10.1002/adfm.201101662
37. Cui C, Fan C, Wu Y, et al., 2019, Water-Triggered Hyperbranched Polymer Universal Adhesives: From Strong Underwater Adhesion to Rapid Sealing Hemostasis. Adv Mater, 31:1905761. https://doi.org/10.1002/adma.201905761
38. Li X, 2017, 2-Phenyl-4,4,5,5-tetramethylimidazoline-1-oxyl 3-Oxide (PTIO) Radical Scavenging: A New and Simple Antioxidant Assay In Vitro. J Agric Food Chem, 65:6288–97. https://doi.org/10.1021/acs.jafc.7b02247
39. Nadgorny M, Collins J, Xiao Z, et al., 2017, 3D-printing of Dynamic Self-healing Cryogels with Tuneable Properties. Polymer Chem, 9:01945. https://doi.org/9. 10.1039/C7PY01945A
40. Zhang Y, Yang B, Zhang X, et al., 2012, A Magnetic Self healing Hydrogel. Chem Commun, 48:9305–7. https://doi.org/10.1039/C2CC34745H
41. Deng G, Li F, Yu H, et al., 2012, Dynamic Hydrogels with an Environmental Adaptive Self-Healing Ability and Dual Responsive Sol-Gel Transitions. ACS Macro Lett, 1:275–9. https://doi.org/10.1021/mz200195n
42. Discher D, Janmey P, Wang YL, 2005, Tissue Cells Feel and Respond to the Stiffness of Their Substrate. Science, 310:1139–43. https://doi.org/10.1126/science.1116995
43. Yang J, Liang J, Zhu Y, et al., 2021, Fullerol-hydrogel Microfluidic Spheres for In Situ Redox Regulation of Stem Cell Fate and Refractory Bone Healing. Bioactive Mater, 6:4801–15. https://doi.org/10.1016/j.bioactmat.2021.05.024